Caspar specifies primordial germ cell count and identity in Drosophila melanogaster
Figures
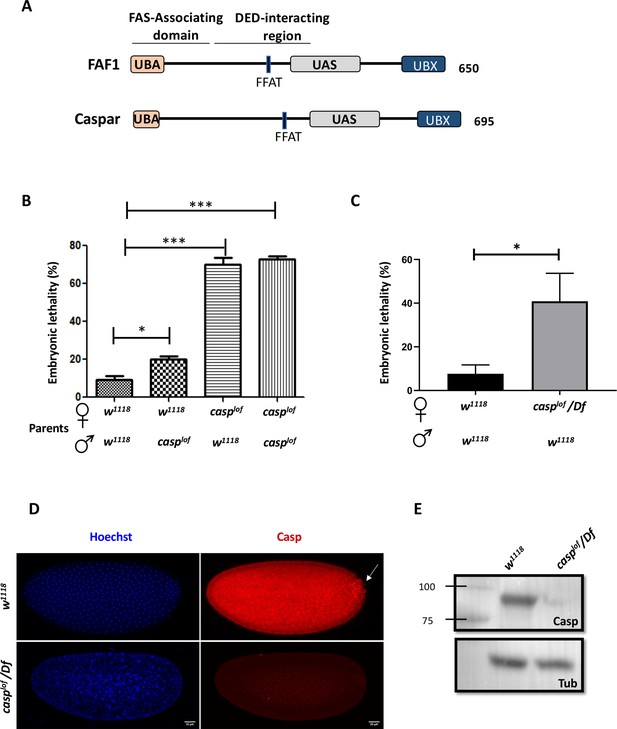
casp is a maternal effect gene.
(A) Comparison between human FAF1 and Casp shows conserved protein domains, which are described in the text. (B) Embryos laid by homozygous casplof females show ~70% lethality, irrespective of the paternal genotype (w1118 or casplof), suggesting a strictly maternal function of casp (C) The use of a deficiency in the casp locus validates the lethal phenotype associated with the mutation, which drops to ~40%. In panels B and C, the parental genotype is listed on the X-axis, with percent embryonic lethality plotted as a bar graph. N=3, ordinary one-way ANOVA/ unpaired t-test, (***) p<0.001, (*) p<0.05. (D) Immunofluorescence images of 0–3 hr embryos derived from w1118 and casplof/Df females, stained with Hoechst and Casp (E) Casp protein levels were assessed in 0–3 embryos laid by w1118 and Casplof/Df animals, evaluated via western blotting. Tubulin is used as a loading control.
-
Figure 1—source data 1
PDF file containing original western blots for Figure 1E, indicating the relevant bands.
- https://cdn.elifesciences.org/articles/98584/elife-98584-fig1-data1-v1.pdf
-
Figure 1—source data 2
Original unedited blots for western blot analysis displayed in Figure 1E.
- https://cdn.elifesciences.org/articles/98584/elife-98584-fig1-data2-v1.zip
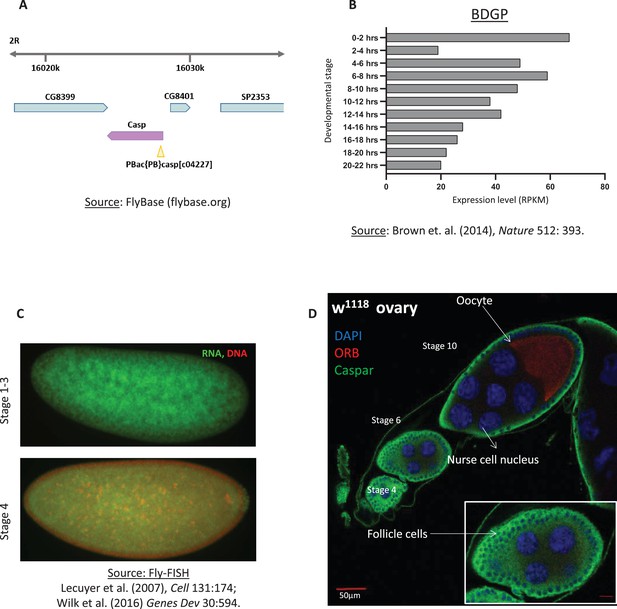
Caspar mRNA is deposited maternally in the oocyte.
(A) Schematic of the chromosomal location of the casp locus and the associated transposon insertion that generates the caspc04227 allele. This allele is referred to as casplof hereafter. (B) RNA expression data from the modENCODE database, plotted as a bar chart (C). DAPI marks the nuclei, orb marks the oocyte. Casp marks the follicle cells, but is not significantly enriched in the germline (egg, nurse cells).
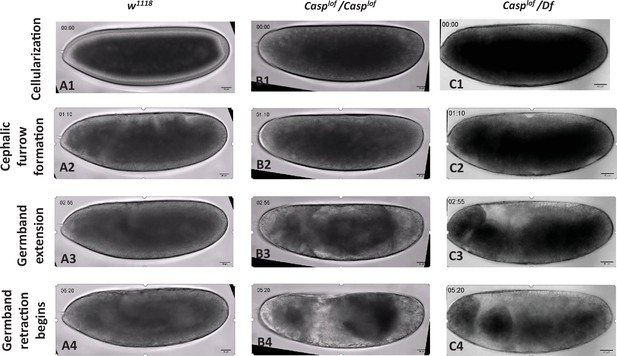
Time-lapse images are presented for the videos (Figure 1—videos 1–3) of w1118 and casp mutants.
Out of 96 time points imaged, four are shown for each genotype. Images were acquired from a single, constant plane unique to each embryo across 5-min intervals. The developmental trajectory for w1118 can be followed from the cellularization stage to the beginning of germ band retraction, for the time points indicated. Crucial developmental milestones are highlighted (A1–A4). casp mutants did not exhibit characteristic stages of morphogenesis at comparable time points (B1–B4, C1–C4), n=10. ~70% embryos laid by the homozygous casplof females failed to hatch into larvae (Figure 1B). Moreover, the number of embryos that failed to hatch did not change substantially when casplof virgin females were mated either with w1118 males or casplof males. That the extent of viability was independent of the paternal genotype established that casp is a maternal effect gene and its activity is required for viability (Figure 1B). To rule out the possibility that the lethality was induced by non-specific background mutations, we procured multiple deficiency (Df) lines spanning the 52D locus and confirmed that casplof/Df mothers also displayed significant embryonic lethality (40%; Figure 1C). Lastly, as in the case of casplof females, casplof males are also semi-fertile which was assessed by mating the mutant males with w1118 virgin females (Figure 1B).
Time lapse imaging of live embryos.
Representative movie for w1118.
Time lapse imaging of live embryos.
Representative movie for casp lof.
Time lapse imaging of live embryos.
Representative movie for casplof /Df.
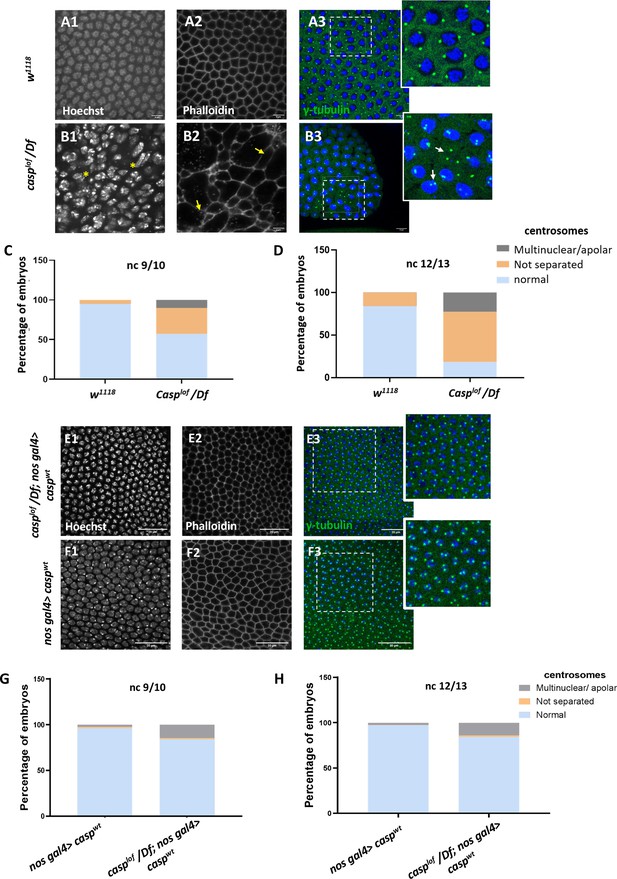
A significant proportion of embryos (~45%) laid by casplof/Df mothers display nuclear division and cytoskeletal defects.
(A, B) Confocal images of nuclear cycle 13/14 embryos (single sections) of the indicated genotypes stained with Hoechst, phalloidin, and gamma-tubulin. Both the regular arrangement and uniform density of nuclei are disrupted in the mutants, as indicated by a yellow asterisk. F-actin, marked with phalloidin, shows a regular, hexagonal compartmentalization in w1118 (panel A2), while disorganized F-actin (white arrows; panel B3) is observed in the mutant. Defective centrosomes marked with gamma-tubulin can also be observed in the Casp mutant (inset of B3, compare to inset A3), indicated by yellow arrows. The extent of defects is quantified in nuclear cycle(nc) 9/10 (C) and nc 12/13 (D). (E, F) Confocal images of sections of nc 13/14 embryos of the indicated genotypes stained with Hoechst, phalloidin, and gamma-tubulin. The nuclear, cytoskeletal, and centrosomal defects are rescued (panel E) when wild-type casp is expressed in the casplof/Df background (compare panels G, H to C, D). Overexpression of casp on its own (panel F) does not appear to affect nuclear or cytoskeletal architecture. The bar charts represent the percentage of defective centrosomes in nuclear cycle 9/10 and 12/13 embryos. Number of embryos imaged ~15.
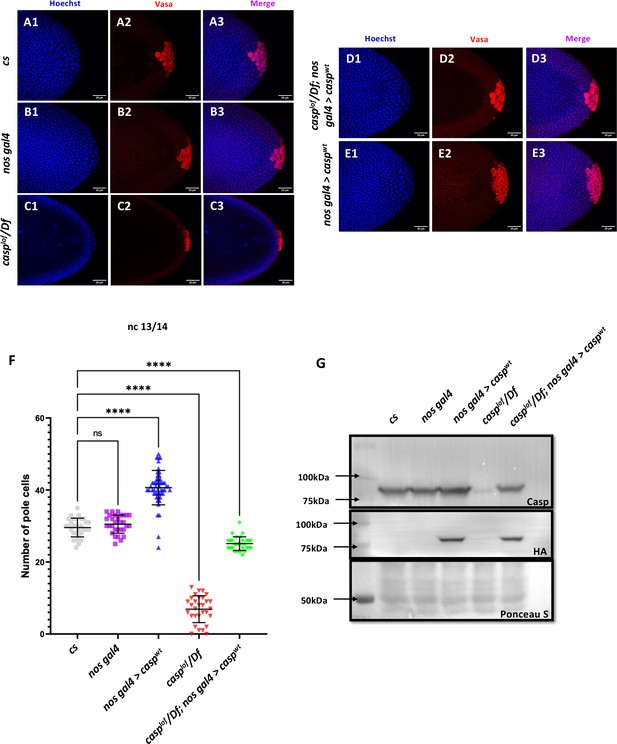
Casp influences total PGC count.
(A–E): Shown are the Confocal images of the posterior terminii of nuclear cycle 13/14 embryos of the following genotypes: cs (A1–A3), nos gal4 (B1–B3), casplof/Df (C1–C3), casplof/Df; nos gal4>caspwt (D1–D3) and nos gal4>caspwt (E1–E3). Embryos were immunostained with Vasa (1:50) antibody. Hoechst marks the nuclei. (F) The total number of germ cells marked with Vasa were quantified and plotted as bar graphs. N (embryos)=30. Ordinary one-way ANOVA, (****) p<0.0001. (G) Casp protein levels were assessed in 0–3 embryos via western blotting. Rabbit anti-Casp (1:10,000) and rabbit anti-HA (1:2000) antibodies were used to probe the blot. Ponceau is used as a loading control. N=3.
-
Figure 3—source data 1
PDF file containing original western blots for Figure 3G, indicating the relevant bands.
- https://cdn.elifesciences.org/articles/98584/elife-98584-fig3-data1-v1.pdf
-
Figure 3—source data 2
Original unedited blots for western blot analysis displayed in Figure 3G.
- https://cdn.elifesciences.org/articles/98584/elife-98584-fig3-data2-v1.zip

TER94 and germline components are major interactors of Casp.
(A) Shows a list of the 24 proteins enriched after a Casp IP, followed by mass spectrometry, listed in the order of their peptide counts. The peptide counts are averaged from 4 biological replicates. A subset of interactors with known functions or expression in the germline are tabulated in (B) a schematic of the interaction of Casp with both TER94 and VAPB (Tendulkar et al., 2022). TER94 interacts with the Casp UBX domain, while VAPB with the FFAT motif. (C) List of proteins that are associated with Casp and are previously identified as pole-cell components. (D, E) Immunostaining of wild-type nuclear cycle 13/14 embryos with Casp (D2) and TER94-specific (E2) antibodies indicates expression in the primordial germ cells. Hoechst marks nuclei and Vasa marks the germ cells.
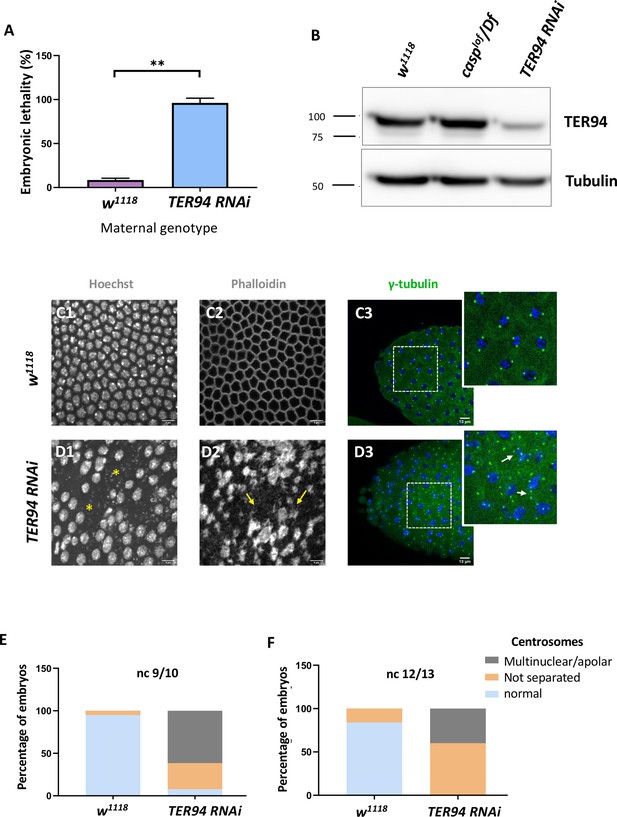
Maternal requirement of TER94 during early embryogenesis.
(A) Viability of embryos derived from w1118 and Mat-αtubGAL4>TER94 i (referred to as TER94 RNAi henceforth) mothers is represented as a bar graph. N=3, ordinary one-way ANOVA, (***) p<0.001. (B) Western blot analysis indicates the knockdown efficiency of TER94 in the 0–3 hr embryo, estimated to be ~90%., levels remain unaffected in casplof/Df embryos. Tubulin was used as a loading control. N=3. (C, D) Confocal images of sections of nuclear cycle 13/14 embryos of the indicated genotypes stained with Hoechst, phalloidin, and gamma-tubulin. Nuclei and F-actin are visualized with Hoechst and phalloidin respectively. Nuclear disruption (D, asterisks) and F-actin aggregates (D2, arrows) are observed in the mutant. Defective centrosomes (D3, arrows, inset) can be observed in the TER94 RNAi embryos. (E, F) The percentage of defective centrosomes in nuclear cycles 9/10 and 12/13 are represented as bar charts. n=10.
-
Figure 4—source data 1
PDF file containing original western blots for Figure 4B, indicating the relevant bands.
- https://cdn.elifesciences.org/articles/98584/elife-98584-fig4-data1-v1.pdf
-
Figure 4—source data 2
Original unedited blots for western blot analysis displayed in Figure 4B.
- https://cdn.elifesciences.org/articles/98584/elife-98584-fig4-data2-v1.zip
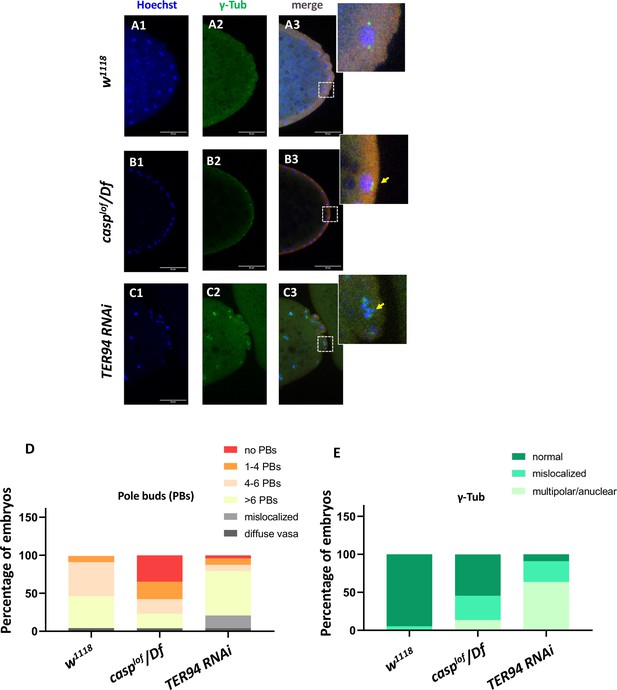
casp and TER94 regulate pole bud formation.
(A–C) Confocal sections of the posterior termini of nuclear cycle 9/10 embryos derived from w1118 (A1–A3), casplof/Df (B1–B3), and TER94 RNAi (C1–C3) mothers immunostained with gamma-tubulin. γ-tubulin labels centrosomes of pole buds, identified based on Vasa staining. Nuclei are stained with Hoechst. The inset(s) for B3 and C3 highlight the centrosomal defects (arrow) when compared with A3. Defects were quantified and plotted as bar graphs in (D) and (E) respectively. n=20.
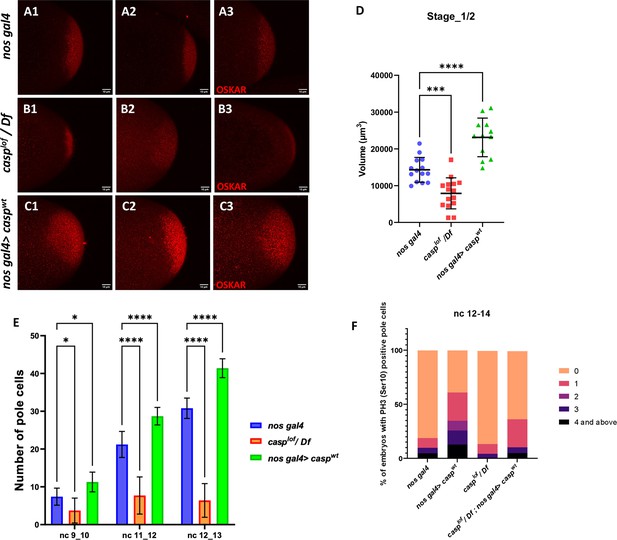
Casp regulates Oskar levels and modulates cell division.
(A–C) Confocal microscopy images of stage 1/2 embryo laid by w1118, casplof/Df, and nos gal4>caspwt females and immunostained with Oskar antibodies. Replicate (1-3) are shown to highlight variable reduction in Oskar levels. In addition, as compared to controls (w1118) embryos (A1–A3), spread of Oskar appears to be restricted in casplof/Df (B1–B3) whereas it is expanded in nos gal4>caspwt (C1–C3). (D) Volume occupied by Oskar, per embryo is measured and plotted for all three genotypes (w1118, casplof/Df, and nos gal4>caspwt), n=8. Ordinary one-way ANOVA, (**) p<0.01, (*) p<0.05. (E) Number of pole buds (nc 9/10) and pole cells (nc 11/12, nc 12/13) in 0- to 3-hr-old nos gal4, casplof/Df and nos gal4>caspwt embryos at different nuclear cycles. Two-way ANOVA, (*) p<0.05, (****) p<0.0001. n=10 (F) Graph showing the distribution of actively dividing pole cells as indicated by the presence of phospho-histone 3 (Ser10) antibody. Quantitative analysis was performed using nuclear cycle 12–14 in 0–3 hr old, nos gal4, casplof/Df, nos gal4>caspWT and casplof/Df;nos gal4>caspWT embryos.
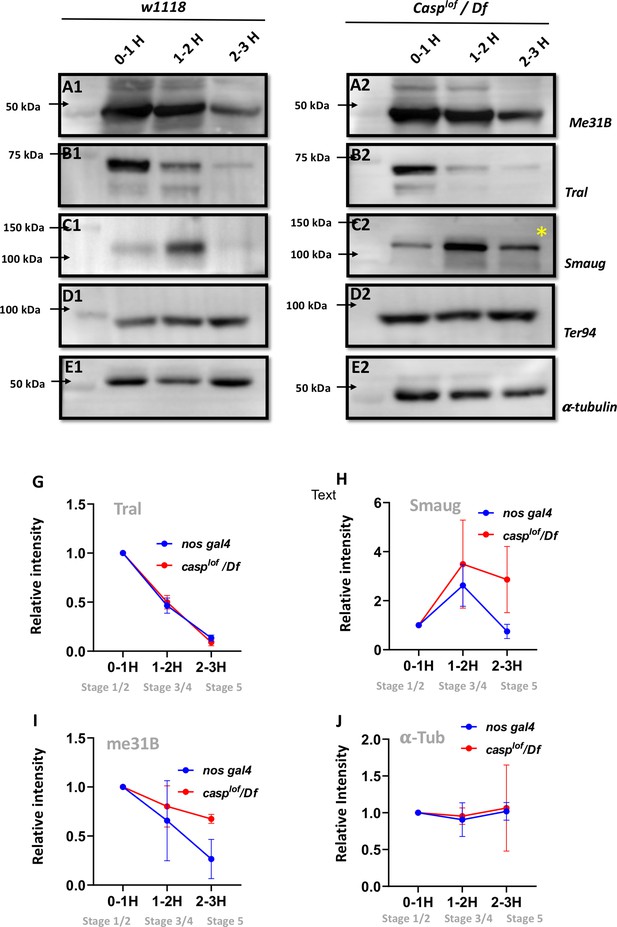
Reduction in Casp activity specifically affects Smaug degradation during the MZT.
At the MZT, a few maternal proteins are actively degraded (Cao et al., 2020). Embryos from mothers with genotype w1118 (A1–E1) and casplof/Df (A2–E2) were collected using three time intervals (0–1, 1–2, 2–3 hr), and embryonic lysates were separated on SDS-PAGE gels. The western blots were probed with antibodies against ME31B (A1, A2), Tral (B1, B2) and Smaug (C1, C2) to assess the extent of protein degradation, as part of the MZT. α-tubulin (E1, E2) was used as a loading control, while TER94 (D1, D2) was used as a negative control as it is a maternal protein that does not undergo degradation at MZT. (G–J) Quantitation of the intensity of change of protein band (normalized to 1), for Tral (G), Smaug (H), ME31B (I) and tubulin (J). Each data point is an average of over five western blots. The intensities at each time point for nos gal4 vs casplof/Df are statistically not significant.
-
Figure 7—source data 1
PDF file containing original western blots for Figure 9B, indicating the relevant bands.
- https://cdn.elifesciences.org/articles/98584/elife-98584-fig7-data1-v1.pdf
-
Figure 7—source data 2
Original unedited blots for western blot analysis displayed in Figure 9B.
- https://cdn.elifesciences.org/articles/98584/elife-98584-fig7-data2-v1.zip
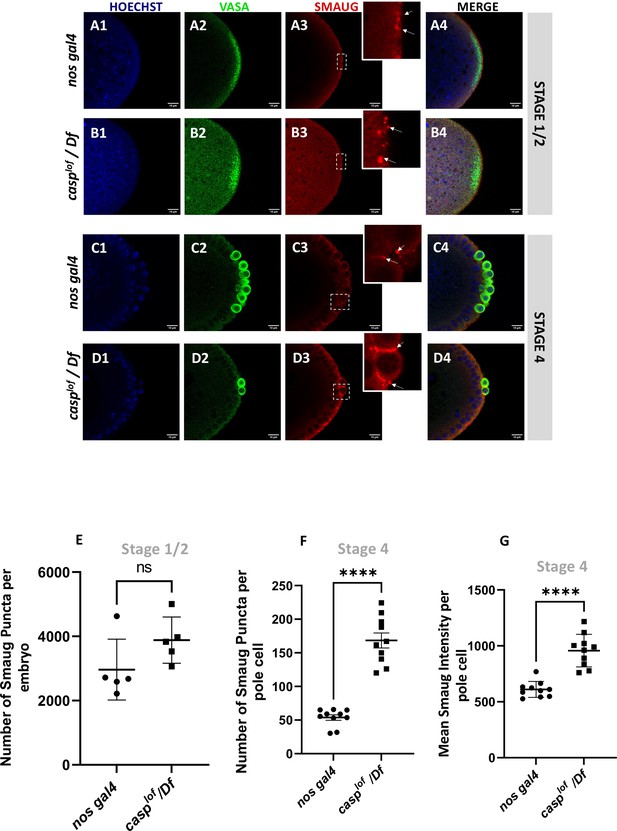
Decrease in Casp correlates with an increase in Smaug levels in the pole buds and PGCs.
Confocal microscopy images of the posterior termini of stage 1–2, and 4 embryos derived from nos gal4 and casplof/Df females. Embryos were immunostained with Vasa (green) and Smaug (red) antibodies. Hoechst marks the nuclei (A–D). Smaug expression was quantified across the two genotypes and plotted as bar graphs (E–G). N=5 embryos (Stage 2) and 10 embryos (Stage 4).
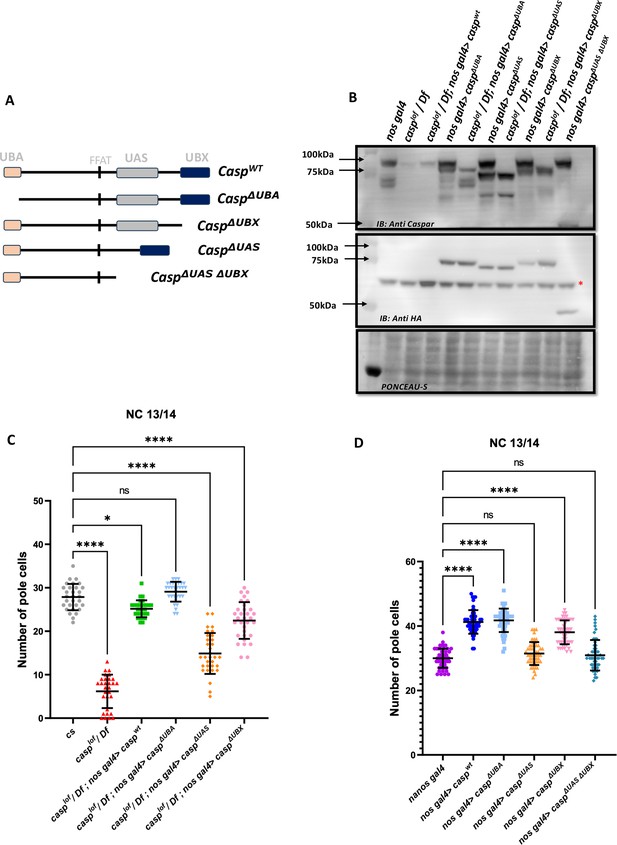
Structure-function analysis of Casp protein domains in regulating pole cell number.
(A) Schematic representation of different domain deletion variants of Casp. As shown in the schematic, the WT Casp protein consists of different functional domains including UBA, UAS, UBX and the FFAT-like motif. (B) Western blot of the truncated Casp proteins, deficient in the domains indicated, probed with rabbit anti-casp (1:10,000) and rabbit anti-HA (1:2000) antibody. nos gal4 served as a control. Ponceau staining was used as a loading control. Asterisk (*) denotes non-specific antibody binding. (C) The total number of germ cells from nc 13/14 embryos derived from mothers expressing different casp domain deletion constructs in the casplof/Df background were marked with Vasa antibodies, quantified and plotted as a bar graph. n=30, Ordinary one-way ANOVA, (****) p<0.0001, (*) p<0.05. (D) The total number of germ cells from nc 13/14, derived from mothers expressing casp domain deletion, in an otherwise wild-type background, were stained with anti-Vasa antibodies. Vasa positive cells (i.e. PGCs) were quantified and plotted as a bar graph. n=30, Ordinary one-way ANOVA, (****) p<0.0001.
-
Figure 9—source data 1
PDF file containing original western blots for Figure 9B, indicating the relevant bands.
- https://cdn.elifesciences.org/articles/98584/elife-98584-fig9-data1-v1.pdf
-
Figure 9—source data 2
Original unedited blots for western blot analysis displayed in Figure 9B.
- https://cdn.elifesciences.org/articles/98584/elife-98584-fig9-data2-v1.zip

Casp:VAP interaction is not required for defining pole cell number.
(A) Casp interacts with VAPB via its FFAT-like motif (B) represents embryonic lethality at 25 ° C plotted as a bar graph. The genotype of the mother is listed on the X-axis. N=3. n=200, ordinary one-way ANOVA, (****) p<0.0001. (C) Casp protein levels were assessed in 0–3 hr embryos via western blotting. Rabbit anti Casp (1:10,000) and rabbit anti HA (1:2000) antibodies were used to probe the blot. Mouse anti Tubulin (1:10,000) is used as a loading control. (D–F) Confocal microscopy images of the posterior of nuclear cycle 13/14 embryos from mated nos gal4 (D1–D3), nos gal4>casp∆FFAT (E1–E3), and casplof/Df; nos gal4>casp∆FFAT (F1–F3) females immunostained with vasa antibody (1:50). Hoechst marks the nuclei. (G) The germ cells at the posterior marked by vasa quantified and plotted as bar graphs. n=30–40. Ordinary one-way ANOVA, (****) p<0.0001.
-
Figure 9—figure supplement 1—source data 1
PDF file containing original western blots for Figure 9—figure supplement 1 indicating the relevant bands.
- https://cdn.elifesciences.org/articles/98584/elife-98584-fig9-figsupp1-data1-v1.pdf
-
Figure 9—figure supplement 1—source data 2
Original unedited blots for western blot analysis displayed in Figure 9—figure supplement 1.
- https://cdn.elifesciences.org/articles/98584/elife-98584-fig9-figsupp1-data2-v1.zip
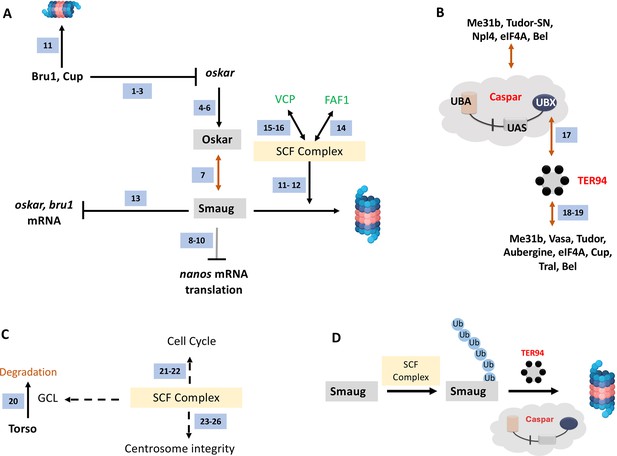
A model for Casp function in PGCs.
(A) Smaug is marked for degradation by the SCF complex. Specific pole plasm components including Cup, Oskar, and Smaug are actively degraded. oskar is regulated by Bru1 and Cup (ref 1–3; Nakamura et al., 2004; Kim et al., 2015; Bayer et al., 2023). Oskar (ref 4–6; Mahowald, 2001; Huynh and St Johnston, 2004; Lehmann, 2016) is the master determinant of PGC fate, and hence the stability of the Oskar:Smaug complex (ref 7; Kubíková et al., 2023) is a key to PGC determination and proper specification. Oskar also regulates posterior cell fate by regulating nos translation which is modulated by Smaug (ref 8–10; Dahanukar et al., 1999; Zaessinger et al., 2006; Jeske et al., 2011). The SCF complex is a multi-protein E3 ubiquitin ligase complex, and Smaug is one of its prominent targets (ref 11–12; Cao et al., 2020; Cao et al., 2022). Smaug appears to repress the translation of oskar and Bruno 1 mRNA (ref 13; Siddiqui et al., 2023). In mammals (marked with green font), FAF1 modulates the SCF complex (ref 14; Morais-de-Sá et al., 2013) with VCP/p97 assisting in the degradation of ubiquitinated Smaug (ref 15–16; Li et al., 2014; Reim et al., 2014), suggesting potential conservation of the activities in the fly orthologs, Casp and TER94. (B) Casp/TER94 interact with an overlapping set of proteins. TER94 has earlier been demonstrated to be associated with Oskar (Ruden et al., 2000). Our data points to an association between Drosophila Casp and TER94 (this study and ref 17; Tendulkar et al., 2022) that participates in protein degradation. In a proteomic analysis of germ cell components, TER94 was identified (this study and ref 18; Thomson et al., 2008), along with other bona-fide germ cell constituents including Cup, Tral, Bel, eIF4A, Tud and Vasa (ref 18 and 19 Thomson et al., 2008; DeHaan et al., 2017). Both, Casp and TER94 are thus enriched in the pole cells and interact with proteins that specify PGC fate. (C). The SCF Complex, Gcl and Centrosome integrity. The SCF complex is localized to the centrosome. Gcl interacts with Cullin 3 to degrade Torso receptor to promote PGC fate (ref 20; Pae et al., 2017). The SCF complex regulates the cell cycle (ref 21–22; Margottin-Goguet et al., 2003; Rogers et al., 2009) and is a known regulator of centrosomal integrity (ref 23–26; Wojcik et al., 2000; Murphy, 2003; Phuong Thao et al., 2006; Cunha-Ferreira et al., 2009), thereby influencing PGC specification (Lerit et al., 2017). (D) Casp and TER94 assist in the degradation of Smaug. The SCF complex works as a ubiquitin E3 ligase to mark Smaug for Degradation and PolyUb-Smaug is degraded by the action of Casp and TER94 during the late-MZT.