Differential regulation of the proteome and phosphoproteome along the dorso-ventral axis of the early Drosophila embryo
Figures
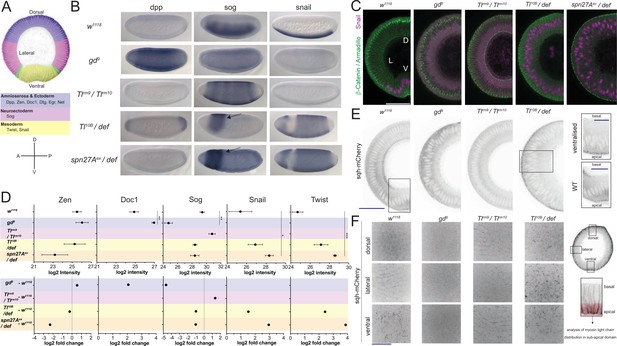
Validation of mutants as representatives of embryonic cell populations.
(A) Top: color-coded schematic of the cell populations along the dorso-ventral axis of a Drosophila embryo during gastrulation: blue, 'dorsal': ectoderm and amnioserosa; magenta, 'lateral': neuroectoderm; green, mesectoderm; yellow 'ventral': mesoderm. Middle: examples of dorso-ventral fate determinants of the domains shown in the top panel. Bottom: dorso-ventral and anterior-posterior axes for reference in panel B. (B) RNA in situ hybridisations using probes for genes expressed in dorsal (dpp), lateral (sog) and ventral (snail) cell populations in wild type (w1118) and embryos derived from mothers mutant for dorso-ventral patterning genes (gd9, dorsalized, Tlrm9 /Tlrmrm10 lateralized, Toll10B/def and spn27Aex/def both ventralized). Notice the expansion of dpp, sog and snail expression in dorsalized, lateralized and ventralized embryos respectively. Arrow indicates remaining neuroectodermal polarity in ventralized embryos. (C) Images (confocal, max-projected) of physical cross-sections from heat-fixed embryos stained using antibodies against β-Catenin/Armadillo (green) and Snail (magenta). D is dorsal domain; L is lateral domain; V is ventral domain. Scale bar is 50 μm. (D) log2 intensity (top) and log2 fold change (FC, bottom) of proteins in wild type, dorsalized (blue), lateralized (magenta), and ventralized (yellow) embryos. Bars depict mean and standard error of the mean across replicates. Absence of a dot indicates the protein was not detected or log2FC calculation not feasible; absence of error bars in log2 intensity indicates protein was detected only in a single biological replicate. Dotted line indicates log2FC = 0. Mean log2 intensity values were compared using one-way ANOVA, followed by pairwise unpaired t-test comparisons (FDR corrected). Significance: * is p<0.05, ** is p<0.01, *** is p<0.001. See Supplementary file 2 for ANOVA and pairwise comparison p-values. (E) Cross-section images (two-photon, single sections) showing Myosin Light Chain (sqh-mCherry) distribution in living wild type, dorsalized, lateralized and ventralized embryos. Insets show magnified ectopic sqh-mCherry signal distribution in wild type vs. ventralized embryos. Scale bar is 50 μm for full view and 25 μm for insets. (F) Images (spinning disk, max-projected) showing myosin distribution in the sub-apical domain of living wild type, dorsalized, lateralized and ventralized embryos along their dorso-ventral axis. Scale bar is 25 μm.
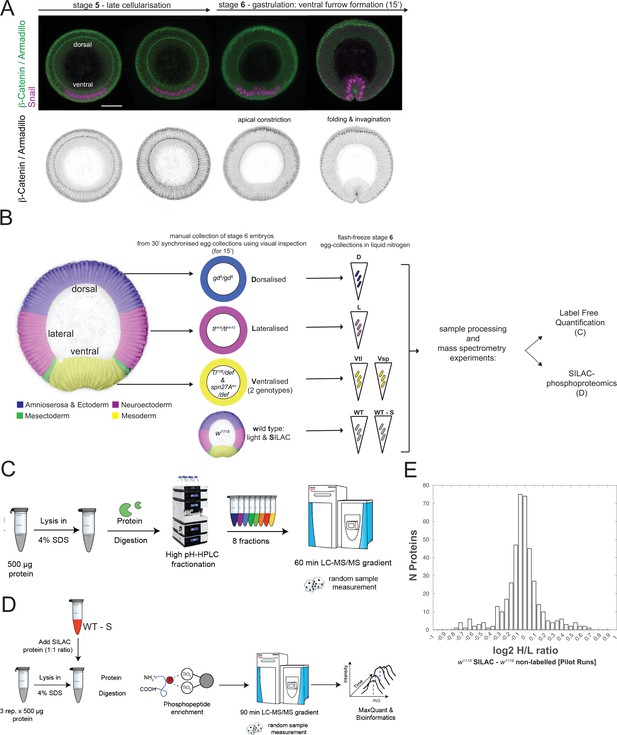
Proteomic and phosphoproteomic strategy in Drosophila embryos at the point of gastrulation.
(A) Images (confocal, max-projected) of physical cross-sections of heat-fixed embryos showing the transition from stage 5 (late cellularization) to stage 6, and the progression of gastrulation during stage 6 (ventral furrow formation), stained using antibodies against β-Catenin/Armadillo (green in top panel; greyscale in bottom panel) and Snail (magenta in top panel). (B) Scheme explaining the strategy for proteomic and phosphoproteomic experiments. Synchronized collections of embryos from wild type or mutant mothers, representing the dorsal ectodermal (dorsalized: gd9), neuroectodermal (lateralized: Tlrm9/Tlrmrm10) and mesodermal (ventralized: Toll10B/def & spn27Aex/def) cell populations were allowed to develop for 2 hr 30' at 25 °C, manually selected under visual inspection for 15' to secure the collection of stage 6 embryos and immediately frozen in liquid nitrogen. For the phosphoproteomic experiments, we used SILAC metabolic labeling to optimize the quantification strategy. (C) Workflow for quantitative label free proteomics using high-pH offline peptide fractionation followed by LC-MS/MS of individual fractions. (D) Workflow for quantitative SILAC-phosphoproteomic analyses. Phosphopeptide enrichment was performed using TiO2 beads, and LC-MS/MS based identification and quantification. For the mass-spectrometry analysis of each replicate, we combined equal amounts of protein from wild type embryos labeled with SILAC-Lys 13/6, and embryos of the target genotype (500 μg target genotype: 500 μg SILAC wild type). (E) Histogram showing the distribution of the number of protein groups with respect to the log2 fold change between the intensity values measured for a given protein group in SILAC wild type vs. non-SILAC wild type embryos. H stands for heavy (or SILAC) and L stands for light (or non-SILAC) in pilot runs.
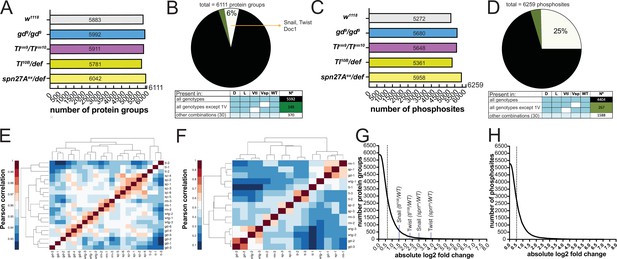
Proteomes and phosphoproteomes of wildtype and mutant embryos.
(A and C) Number of protein groups (A) or phosphosites (C) detected in wildtype, dorsalized (gd9), lateralized (Tlrm9/Tlrmrm10), and ventralized embryos (Toll10B/def and spn27Aex/def). (B and D) Intersection analysis of detected protein groups (B) or phosphosites (D). Black: detected in at least 1 replicate in all genotypes; green: detected in at least 1 replicate in all genotypes except 1 ventralized condition; white: detected in at least 1 replicate in any other combination. (E and F) Correlation matrix between the replicates of the proteomic (E) and phosphoproteomic (F) experiments using the Pearson correlation coefficient. Protein groups and phosphosites detected in all of the replicates in all of the genotypes were used to construct the correlation matrices. Proteomic (LFQ) analyses were performed using three technical replicates, with the exception of spn27aex/def and gd9 genotypes in which we used two biological replicates with three technical replicates each, making a total of six replicates for these two genotypes. For SILAC phosphoproteomic analyzes the protein lysate from embryos of each genotype was split in three and conducted three separate analyses. (G and H) Distribution of the number of protein groups (G) or phosphosites (H) exceeding an absolute fold change (vs. wild type, in log2 scale). Dotted line depicts the absolute fold change corresponding to 50% of the analyzed protein groups (G) or phosphosites (H).
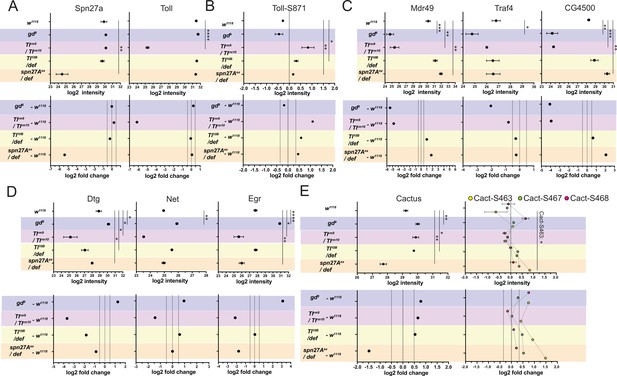
Proteomic validation of dorso-ventral embryonic cell populations.
(A) log2 intensity (top) and log2 fold change (FC, bottom) of Toll and Spn27A proteins. (B) log2 intensity (top) and log2 fold change (bottom) of Toll phosphosite S871. (C) log2 intensity (top) and log2 fold change (bottom) of additional mesodermal proteins. (D) log2 intensity (top) and log2 fold change (bottom) of additional ectodermal fate determinants. (E) log2 intensity (top) and log2 fold change (bottom) of Cactus protein (left panels) and Cactus phosphosites (right panels): S463 (yellow dots), S467 (green dots), and S468 (magenta dots). Colors depict mutant genotypes and their comparisons against wild type: blue: dorsalized, magenta: lateralized, yellow: ventralized (Toll10B/def and spn27Aex/def). Bars depict mean and standard error of the mean across replicates. Absence of a dot indicates that the protein was not detected in a particular condition or that the log2 FC calculation was not feasible; absence of error bars in log2 intensity indicates that the protein was detected only in a single replicate. Dotted line indicates log2 FC = 0, log2 FC = 0.5 (for proteins) or log2 FC = 0.35 (for phosphosites). For all mean log2 intensity comparisons, we conducted a one-way ANOVA, followed by pairwise unpaired t-test comparisons (FDR corrected). Significance: * is p<0.05, ** is p<0.01, *** is p<0.001 and **** is p<0.0001. See Supplementary file 2 for ANOVA and pairwise comparison p-values.
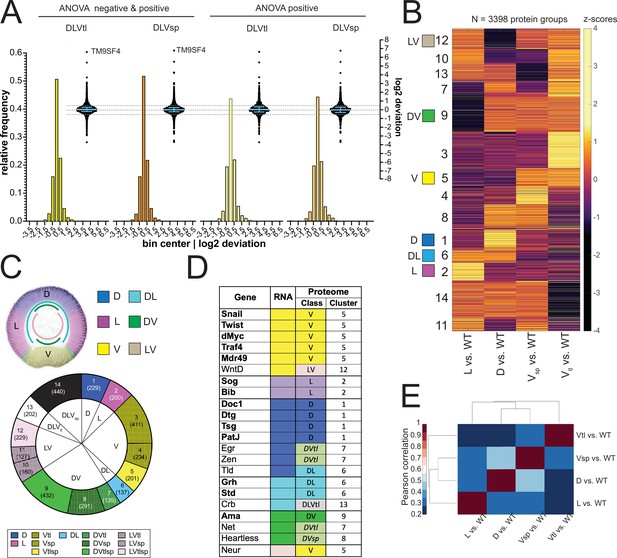
Analysis of the proteomes.
(A) Two different representations, a histogram and a swarm plot, of the deviation parameter (in log2 scale) calculated for each of the two ventralized genotypes (D: dorsalized, L: lateralized, Vtl: Toll10B/def, Vsp: spn27Aex/def). This was done once for all proteins present in all genotypes, and once only for those that were ANOVA positive. In the swarm plot, each dot represents a protein. The y-axis for the histograms is shown on the left, for swarm plots on the right. Blue bars show the median with interquartile range (IQR). The median is close to zero and the IQRs range from –0.18 to 0.3. The dotted line indicates the 0.5 and –0.5 deviation in swarm plots. Histograms and swarm plots were assembled with proteins detected in all genotypes. (B) Clustergram of the hierarchical clustering (dendrograms not shown) of 3398 filtered proteins. Z-scores were calculated using the thresholded fold changes between DV mutants and wild type. Numbers identify the different clusters for reference across panels and figures. Coloured boxes indicate the DV clusters that show consistent behavior for the two ventralising genotypes (for color-coding see panel C). (C) Top: Regulation categories emerging from hierarchical clustering: D (blue), increased abundance in dorsal domain; L (magenta), increased abundance in lateral domain; V (yellow), increased abundance in ventral domain; DL (cyan) increased abundance in dorsal and lateral domains; DV (green), increased abundance in dorsal and ventral domains; LV (pink) increased abundance in lateral and ventral domains. Bottom: Pie chart showing the number of protein groups (in brackets) allocated to each cluster in (B), grouped by their regulation category. (D) Genes with restricted dorso-ventral expression with their reported RNA expression pattern, their allocation to proteome clusters (numbers refer to clusters in panel B and C), and their regulation category along the dorso-ventral axis. (E) Correlation matrices using Pearson correlation coefficient between the fold changes of each DV mutant vs. wild type in the proteome experiment.
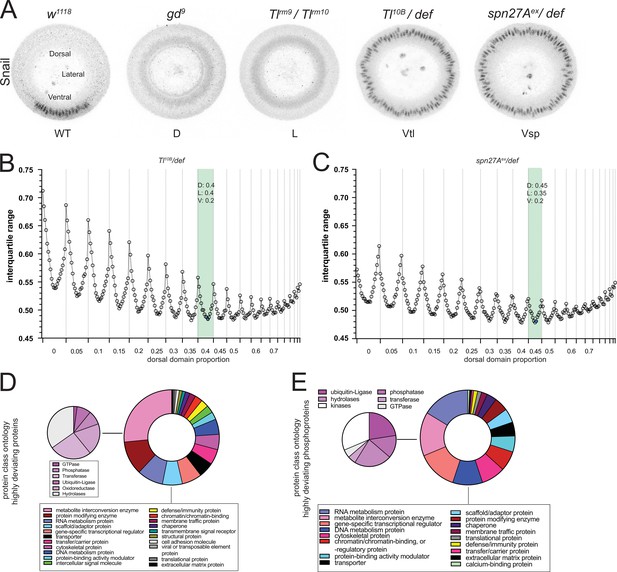
Proteomic validation of dorso-ventral embryonic cell populations.
(A) The protein-level expression of the transcription factor Snail in DV mutants phenocopies the expression pattern across DV cell populations in wild type embryos. Images (confocal, max-projected) of physical cross-sections from heat-fixed embryos showing Snail (grayscale) antibody signal in wild type, dorsalized (D), lateralized (L) and ventralized embryos (Vtl: Tl10B/def and Vsp: spn27Aex/def). (B and C) Outcome of the systematic exploration for the optimal combination of cross-sectional proportions for dorsal (D), lateral (L) and ventral (V) cell populations, with each population being varied in steps of 0.05. On the x axis, the 233 possible combinations are plotted in the order of the proportions assigned to the D population, and the remaining proportion distributed between L and V. Only the values for D are indicated on the axis, see Supplementary file 6 for the L and V values. The y axis shows the Interquartile Range (IQR) of the distribution of the log2 Deviations obtained with each possible combination of D, L and V proportions. Calculations were performed independently for the Toll10B/def (B) or spn27Aex/def (C) data. Light blue dots indicate the best combinations with a ventral domain proportion (V=0.2) that matches the experimentally determined region occupied by the mesoderm. (D) Pie charts showing the protein class ontology (http://www.pantherdb.org/) of proteins with the highest absolute deviations (95th percentile). Inset pie chart shows the children ontology terms for the 'Metabolite interconversion enzyme' class. (E) Pie charts showing the protein class (Panther) ontology of the host proteins for phosphosites with the 5% most extreme absolute deviations (>95 th percentile). The left pie chart shows the children ontology terms for the 'Metabolite interconversion enzyme' class.
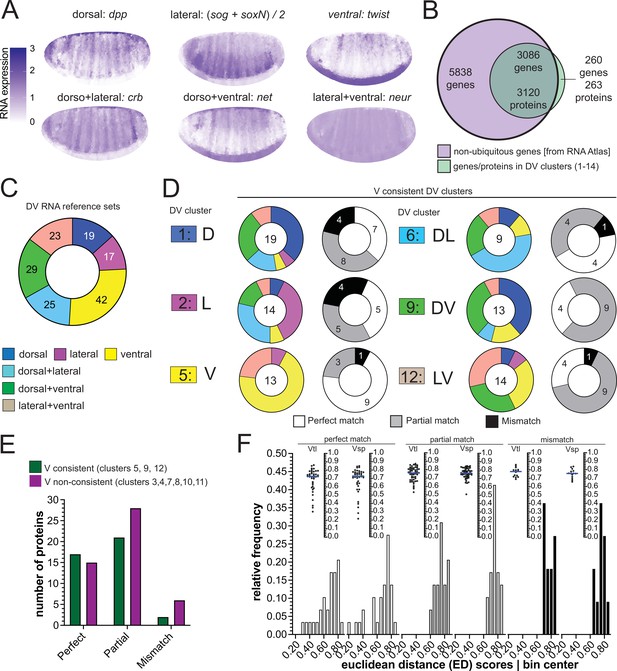
Comparison of RNA and protein expression patterns.
(A) Expression patterns of the reference genes used for assembling the RNA reference sets. Scale bar indicates RNA expression strength. Blue depicts high RNA expression. (B) Venn diagram showing the intersection between the genes of the RNA atlas with non-ubiquitous expression (8924) and the genes that encode the proteins assigned to DV clusters (3383/3398 proteins were successfully matched to a FBgn gene identifier). (C) Number of non-ubiquitous genes allocated to each DV RNA reference set that trespassed the corresponding filter/threshold values (155/8924 genes, see Methods and Supp. B–D). (D) Matching of genes in the RNA reference sets with the proteome DV clusters in which the ventralized mutants display a consistent behavior against the wild type (DV clusters 1, 2, 5, 6, 9, and 12, see Figure 3B and C). Coloured pie charts represent the allocation of genes within each DV cluster to the RNA reference sets (color code as in Figure 3). Grayscale pie charts represent the same sets of proteins, but marked by the outcome of the comparison between RNA and protein expression inferred from clusters: white: perfect match; gray: partial, if the RNA reference included the correct match but also another region; black: mismatch, where the protein expression did not overlap with the RNA reference. Values in the center of pie charts indicate the number of genes compared. Numbers in grayscale pie charts indicate the number of proteins with a perfect (white), partial (gray) or mismatch (black). (E) Number of proteins in each outcome of the RNA-proteome comparison (perfect, partial, mismatch) in ventralized-consistent clusters (dark green) and ventralized non-consistent clusters (dark magenta) that belong to the same regulation categories: ventral (3,4,5), dorsal + ventral (7,8,9) and lateral + ventral (10,11,12). (F) Two different representations, histogram and a swarm plot of the distributions of the Euclidean distance score for proteins that had a perfect, partial or mismatching overlay with the DV RNA reference sets. The histogram and the scatter plots are shown separately for calculations using each ventralized genotype: Vtl = Toll10B/def; Vsp = spn27Aex/def. In the swarm plots, each dot is a protein.
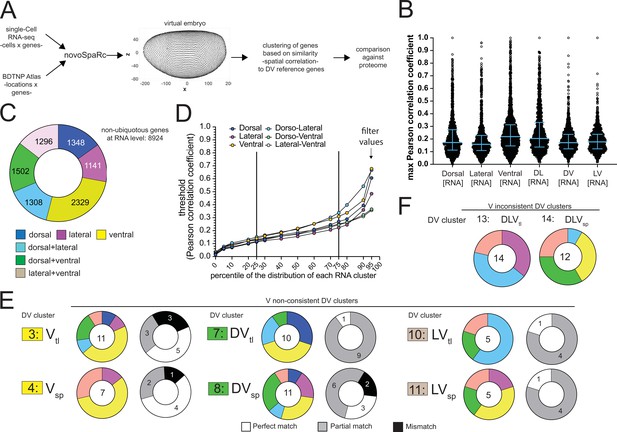
Methodology and supporting data for the comparison between RNA and protein abundance.
(A) Methodology for clustering non-ubiquitous genes according to their dorso-ventral RNA expression. (B) Swarm plot showing the distribution of the maximum Pearson spatial correlation values, used for the assignment of non-ubiquitous genes to a particular DV RNA set. Each dot is a gene. DV RNA sets are mutually exclusive. Bars indicate median and the interquartile range (IQR). (C) Number of non-ubiquitous genes allocated to each DV RNA reference set using the Pearson spatial correlation to reference genes (this figure: panel B and Figure 4A). (D) Maximum Pearson correlation values for increasing percentiles within the distributions of each RNA reference set. To assemble the DV RNA reference sets (Figure 4C), we filtered the genes that at the RNA level displayed the largest variation along the dorso-ventral axis. For this, we selected genes with a maximum Pearson correlation value larger than the 95% percentile (arrow) of the corresponding DV RNA set distribution. (E) Matching of genes in the RNA reference sets with the proteome DV clusters in which the ventralized mutants display a non-consistent behavior against the wild type (DV clusters 3, 4, 7, 8, 10, and 11, see Figure 3B and C). Coloured pie charts represent the allocation of genes within each DV cluster to the RNA reference sets (color code as in Figure 3C). Grayscale pie charts represent the outcome of the comparison for filtered genes: white: perfect for an exact match, gray: partial, if the RNA reference included the correct match but also another region, black: mismatch, where the protein expression did not overlap with the RNA reference. Values in the center of pie charts indicate the number of genes compared. (F) Comparison between the identity of genes in the RNA reference sets with DV clusters 13 and 14 (Figure 3B and C), with proteins that displayed increased abundance in all DV mutants (vs. wild type) with the exception of either of the ventralized genotypes (DLVtl or DLVsp). Coloured pie charts represent the allocation of genes within these DV clusters to the DV RNA reference sets (Blue: dorsal, magenta: lateral, yellow: ventral, cyan: dorsal + lateral, green: dorsal +ventral, pink: lateral +ventral).
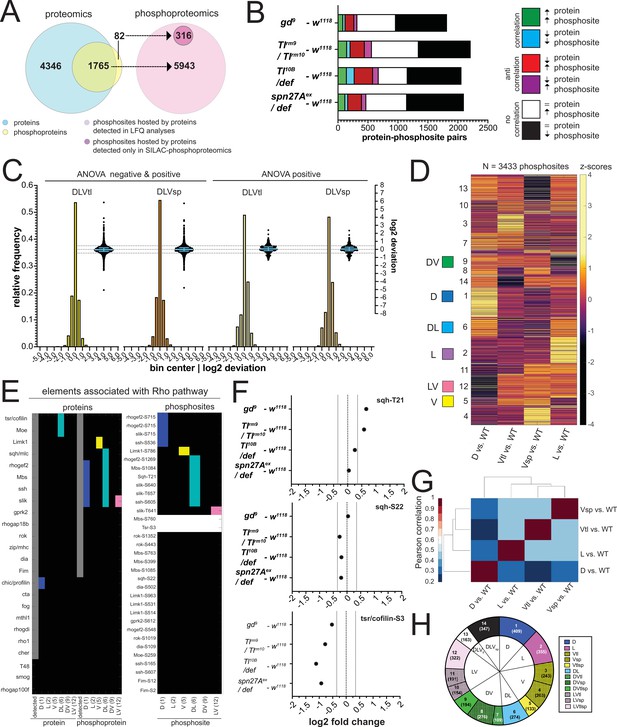
The phosphoproteomes of the mutant embryos.
(A) Match between detected protein groups in the proteomic and phosphoproteomic experiments. Left: light blue: protein groups detected in proteomes (LFQ); overlay between light blue and yellow: protein groups detected both in proteomic and phosphoproteomic experiments; non-overlapping yellow: protein groups not detected in proteomes but detected in phosphoproteomes. Right: pink: phosphosites hosted by a protein group detected in the proteomic analyses; magenta, phosphosites that could not be matched to a protein group detected in the proteomes. (B) Correlation between the fold changes (FCs) of phosphosites and their host proteins in DV mutants vs. wild type. Correlations: FC of protein and phosphosite are both positive (green) or negative (blue). Anti-correlation: FC of protein and phosphosite have different signs (red and magenta). No correlation: protein levels are unchanged but phosphosite FC is positive (white) or negative (black). Bars represent the number of phosphosite-host protein pairs falling in each correlation category within each DV mutant vs. wild type comparison. (C) Two different representations, a histogram and a swarm plot, of the deviation parameter (in log2 scale) calculated for each of the two ventralized genotypes (D: dorsalized, L: lateralized, Vtl: Toll10B/def, Vsp: spn27Aex/def). This was done once for all phosphosites present in all genotypes, and once only for those that were ANOVA positive. In the swarm plot, each dot represents a phosphosite. The y-axis for the histograms is shown on the left, for swarm plots on the right. Blue bars show the median with interquartile range (IQR). The median is close to zero and the IQRs range from –0.2387 to 0.3197. The dotted line indicates the 0.35 and –0.35 deviation in swarm plots. Histograms and swarm plots were assembled with phosphosites detected in all genotypes. (D) Clustergram of the hierarchical clustering of 3433 phosphosites. Z-scores were calculated using the thresholded fold changes between mutants and wild type. Coloured boxes indicate the clusters with consistent behavior for the two ventralising genotypes (for color-coding see Figure 3D). Numbers identify the different clusters for reference across panels, and are equivalent to the proteome (Figure 3B and C). (E) Detection and predicted regulation (DV clusters) of Rho pathway proteins and phosphoproteins (left panel) and the corresponding phosphosites (right panel). Colors mark proteins, phosphoproteins or phosphosites in DV clusters with ventralized consistent behavior(DV clusters 1, 2, 5, 6, 9, and 12). Gray boxes represent the detection of a particular protein or phosphoprotein in the wild type genotype. White boxes represent an increased or decreased abundance in all DV mutants vs. wild type. (F) log2 fold changes (FC) of known phosphosites in sqh: T21 (top) and S22 (center) and tsr/cofilin S3 (bottom). Dotted lines indicate log2 FC = 0, 0.35 and –0.35. Colors depict DV mutant genotypes and their corresponding comparisons against wild type: blue: dorsalized, magenta: lateralized, yellow: ventralized (Toll10B/def and spn27Aex/def). Bars depict mean and standard error of the mean across replicates. Absence of a dot indicates the protein was not detected in a particular condition or log2 FC calculation not feasible, absence of error bars in log2 intensity indicate protein was detected in a single replicate. Dotted line indicates log2 FC = 0 and log2 FC = 0.35 (for phosphosites). (G) Correlation matrices using Pearson correlation coefficient between the fold changes of each mutant vs. wild type comparison in the phosphoproteome experiment. (H) Pie chart showing the number of phosphosites allocated to each DV class in (C).
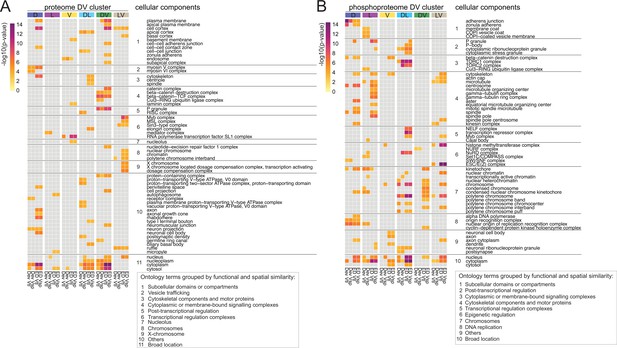
Diffused network analyses of DV proteomes and phosphoproteomes.
Heatmap representations of cellular component ontology terms that were significantly enriched in at least two networks across all DV clusters and showed a consistent behavior in the ventralized genotypes (DV clusters 1, 2, 5, 6, 9, and 12). Ontology terms were grouped based on spatial and functional association. (A) Cellular components enriched in networks emerging from the proteome. (B) Cellular components enriched in networks emerging from the phosphoproteome. Calibration bar indicates the -log10(p-value) for a measure of statistical significance across ontology terms and DV clusters. For each DV class, four diffused networks were generated using the deviation ('Dev'), or the euclidean distances ('ED') to score the nodes of emerging networks. Calculations were performed independently for each score and each ventralized genotype, Vtl (Toll10B/def) and Vsp (spn27Aex/def).
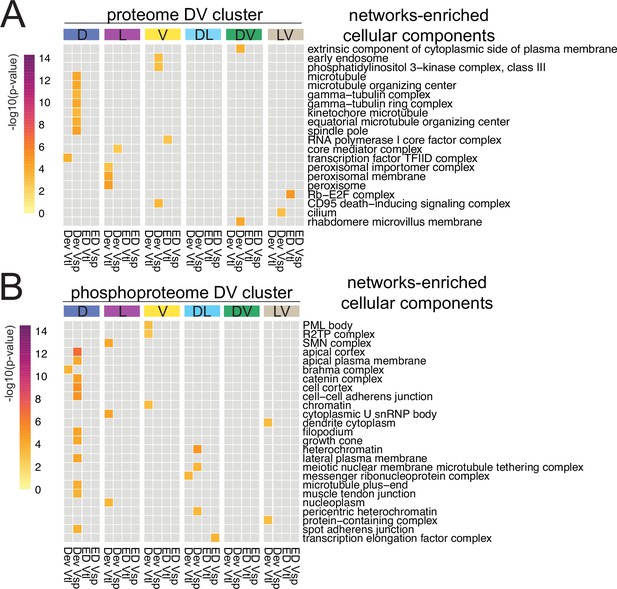
Cellular component terms significantly enriched in diffused networks.
Heatmap representation of the significantly enriched cellular component terms significantly enriched in a single network across all DV clusters. In (A) filtered out proteome ontology terms; in (B) filtered out phosphoproteome ontology terms.
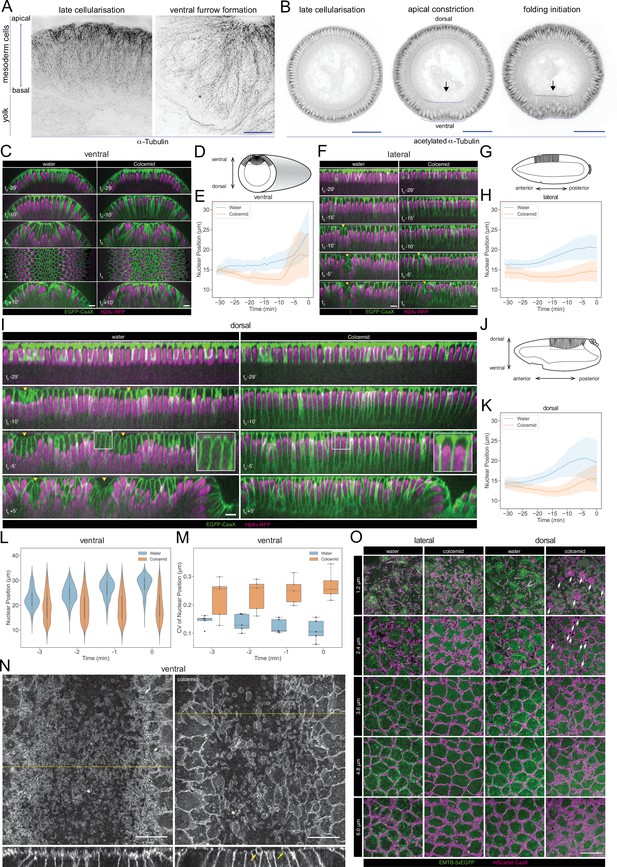
Microtubule organization and in vivo functions.
(A) Images (OMX super-resolution microscope, max-projected) of mesoderm cells (ventral domain) using physical cross-sections from fixed embryos stained with antibodies against α-Tubulin. Left panel: onset of gastrulation, right panel: contractile mesoderm during ventral furrow formation. Scale bar is 10 μm. (B) Images (confocal, max-projected) of physical cross-sections from fixed embryos stained with an antibody against acetylated α-Tubulin at the onset (left panel) and during ventral furrow formation (center panel: initiation of gastrulation, apical constriction; right panel: mesoderm folding). Arrow indicates detection of acetylated α-Tubulin specifically in basal-lateral microtubules (inverted basket). Dotted blue line encloses mesodermal cells, in which a progressive reduction of acetylated α-tubulin is detected during ventral furrow formation. Scale bar is 50 μm. (C, D, E) Phenotypic effect of colcemid injection on the ventral side of the embryo during cellularization and early gastrulation. (C) Time-lapse series of Z re-slice (and a surface projection for the t0 time point) showing cellular and tissue architecture with membrane (EGFP-CaaX) and nucleus (H2Av-RFP) labels. (D) A schematic drawing of tissue architecture during ventral furrow formation with a dotted rectangular box depicting the ROI of the re-slice view in panel C. (E) Nuclear position, that is distance from the embryo surface, as a function of time during cellularization (Water: 26-266 nulcei from 5 embryos; Colcemid: 112-350 nulcei from 5 embryos). (F, G, H) Same as above for the lateral side of the embryo with (F) time-lapse series of Z re-slice, (G) a schematic drawing during cephalic furrow formation and a dotted rectangular box for the ROI in panel F, and (H) nuclear position as a function of time (Water: 82-158 nulcei from 3 embryos; Colcemid: 100-161 nulcei from 4 embryos). (I, J, K) Same as above for the dorsal side of the embryo with (I) time-lapse series of Z re-slice, (J) a schematic drawing during dorsal fold formation and a dotted rectangular box for the ROI in panel E, and (K) nuclear position as a function of time (Water: 88-208 nulcei from 5 embryos; Colcemid: 83-211 nulcei from 7 embryos). Insets, enlarged view showing the shape of the apical dome. (L, M) Colcemid treatment leads to a wider distribution of nuclear positions in apically constricting VF cells during the early phase of apical constriction, shown in a violin plot for nuclear centroid position (L; Water: 7-125 nulcei from 5 embryos; Colcemid: 67-180 nulcei from 5 embryos) and a box plot for its coefficient of variation (M; Water: 5 embryos; Colcemid: 5 embryos). For all of the above, t0 represents the onset of gastrulation, as defined in M&M. Yellow arrowheads: surface clefts resulting from cephalic furrow (F) and dorsal fold (I) initiation. (N) Apical surface projection (top row) of membrane (3xmScarlet-CaaX) and Z re-slice (bottom row; taken from the yellow dotted lines in the top row) showing enlarged membrane blebs (yellow arrows) after colcemid injection during ventral furrow apical constriction. (O) Apical membrane phenotypes in the lateral and dorsal cells observed at different Z positions, each with a 1.2 μm projection, visualized with membrane (3xmScarlet-CaaX) and microtubule (EMTB-3xEGFP) labels. White arrows: abnormal membrane blebs that are devoid of microtubules and observed exclusively on the dorsal side. Scale bars: 10 μm.
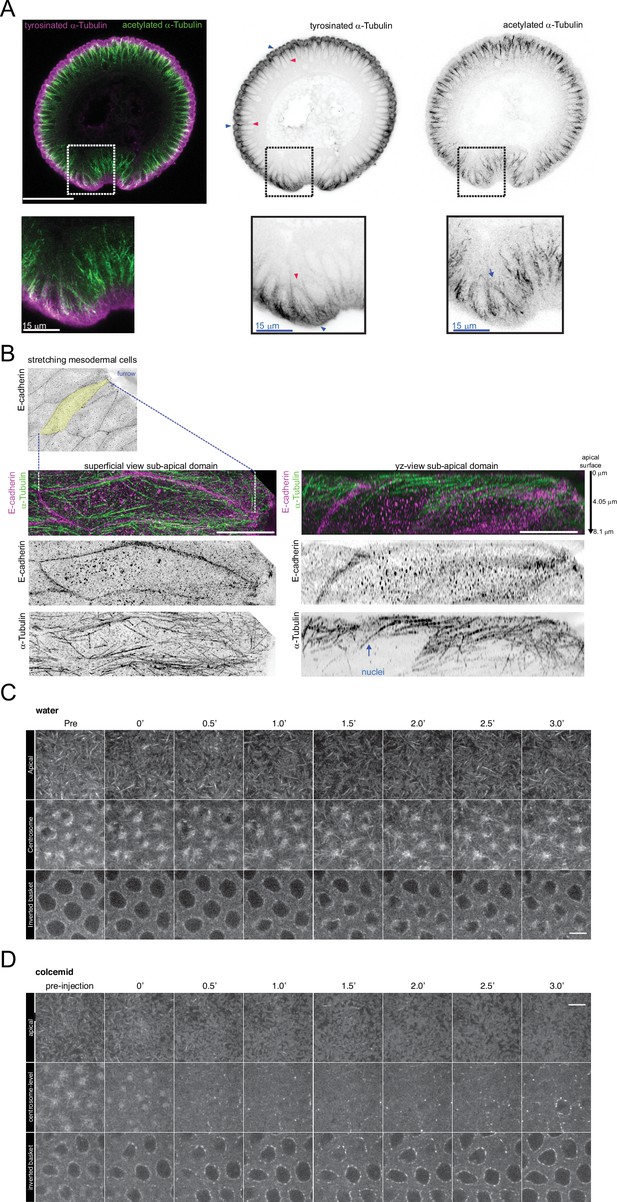
Microtubule analyses during gastrulation and calibration of colcemid injections.
(A) Image (confocal, max-projected) of a physical cross-section from fixed embryos stained with antibodies against tyrosinated (magenta) and acetylated (green) α-tubulin during ventral furrow formation. Arrow: detection of acetylated α-tubulin specifically in basal-lateral microtubules (inverted basket); blue arrowhead: tyrosinated α-tubulin in sub-apical microtubules; red arrowhead: tyrosinated α-tubulin in basal-lateral microtubules. Insets show acetylated and tyrosinated α-tubulin in stretching and constricting mesodermal cells; arrowheads/arrow in insets show differential acetylation and tyrosination of microtubules in a mesodermal cell undergoing stretching. Scale bar is 50 μm. (B) Images (OMX super-resolution microscope, max-projected) of stretching mesoderm cells (ventral domain) from fixed embryos stained using antibodies against E-cadherin (magenta in top panels; grayscale in middle panels) and α-Tubulin (green in top panels; grayscale in bottom panels). A stretching mesodermal cell (yellow-coloured) was cropped and shown with superficial (left panel) and resliced (to show 'y-z' planes) views. The ventral furrow is located to the right of the field of view. Arrow indicates a microtubule in the sub-apical domain that is bent towards the direction of cell stretching (furrow). Scale bar is 10 μm. (C, D) Time lapse series of microtubules labeled with EMTB-3xEGFP at the levels of the apical cell surface, centrosomes and nuclei (inverted basket) prior to and following water (C) or colcemid (D) injection. The time gap between pre-injection recording and post-injection t=0 is~2’.
Videos
Live imaging of ventral furrow formation in a representative Drosophila embryo (gastrulation stage 6), after water (control, left panel) or Colcemid injection (right panel).
Membranes are labeled in green (EGFP-CaaX) and nuclei are labeled in magenta (H2Av-mRFP1). Scale bar is 10 μm.
Live imaging of cephalic furrow formation along the lateral side of a representative Drosophila embryo (gastrulation stage 6), after water (control, left panel) or Colcemid injection (right panel).
Membranes are labeled in green (EGFP-CaaX) and nuclei are labeled in magenta (H2Av-mRFP1 [71]). Scale bar is 10 μm.
Live imaging of dorsal fold formation (mid-sagittal view) of a representative Drosophila embryo (gastrulation stage 6), after water (control, left panel) or Colcemid injection (right panel).
Membranes are labeled in green (EGFP-CaaX) and nuclei are labeled in magenta (H2Av-mRFP1 [71]). Scale bar is 10 μm.
Additional files
-
Supplementary file 1
Summary of the expression pattern of DV fate markers (dpp, sog, snail) in the wild type and DV patterning mutants.
- https://cdn.elifesciences.org/articles/99263/elife-99263-supp1-v2.docx
-
Supplementary file 2
Summary the statistical analyses (ANOVA and t-tests) of protein groups and phosphosites that are shown in figures.
p values are presented as -log10(p-value).
- https://cdn.elifesciences.org/articles/99263/elife-99263-supp2-v2.xlsx
-
Supplementary file 3
Protcome (LFQ) data.
p values are presented as -log10(p-value). Empty cell in gene and protein name indicate the detected protein had not been given a gene and/or a protein name in the Uniprot database version used in this study. NaN indicates a protein that was not detected in a particular replicate.
- https://cdn.elifesciences.org/articles/99263/elife-99263-supp3-v2.xlsx
-
Supplementary file 4
SILAC Phosphoproteomics data.
p values are presented as -log10(p-value). Empty cell in gene and protein name indicate the detected phosphosite is hosted by a protein that had not been given a gene and/or a protein name in the Uniprot database version used in this study. NaN indicates a phosphosite that was not detected in a particular replicate.
- https://cdn.elifesciences.org/articles/99263/elife-99263-supp4-v2.xlsx
-
Supplementary file 5
Linear model implementation: IQR values of the deviation distributions obtained with the systematic exploration of dorsal, lateral and ventral domain proportions.
- https://cdn.elifesciences.org/articles/99263/elife-99263-supp5-v2.xlsx
-
Supplementary file 6
Log2 deviation values of proteins and phosphosites detected in all genotypes using the dorso-ventral domain proportions: D: 0.4 L: 0.4 V: 0.2.
For each experiment (LFQ/Proteomics, SILAC-phosphoproteomics), there is a list of the deviation values of the complete (ANOVA positive and negative) and regulated (ANOVA positive) proteins or phosphosites.
- https://cdn.elifesciences.org/articles/99263/elife-99263-supp6-v2.xlsx
-
Supplementary file 7
List of proteins and phosphosites within all DV clusters (1-14).
Empty cell in gene and protein name indicate the detected protein (or protein that hosts a phosphosite) had not been given a gene and/or a protein name in the Uniprot database version used in this study.
- https://cdn.elifesciences.org/articles/99263/elife-99263-supp7-v2.xlsx
-
Supplementary file 8
RNA Proteome comparison: outcome of the RNA-proteome comparison for the list of genes with a mesoderm label in BDGP (https://insitu.fruitfly.org/cgi-bin/ex/insitu.pl), that are also present in the DV clusters.
- https://cdn.elifesciences.org/articles/99263/elife-99263-supp8-v2.xlsx
-
Supplementary file 9
RNA Proteome comparison: list of filtered genes from the RNA atlas (155/8924), with their corresponding: DV RNA reference set, proteome DV cluster, Pearson correlation value that allocated each gene to its DV RNA reference set, and the outcome of the RNA-Proteome comparison.
The outcome of the RNA-Proteome comparison (perfect, partial or mismatch) is indicated in two different ways: 1 = 'yes' and 0 = 'no' for each type of outcome, and by coloring the rows (each compared gene-protein distribution); white is perfect overlap, gray is partial overlap and black is a mismatch. Red rows indicate proteins were not assigned to any outcome in the RNA-proteome comparison (Clusters 13 -DLVTl10B/def- and 14 -DLVspn27Aex/def-).
- https://cdn.elifesciences.org/articles/99263/elife-99263-supp9-v2.xlsx
-
Supplementary file 10
Deviation values of proteins and phosphosites in DV clusters 1-12.
Empty cell in gene and protein name indicate the detected protein (or protein that hosts a phosphosite) had not been given a gene and/or a protein name in the Uniprot database version used in this study. NaN indicates it was not possible to calculate the deviation using a particular ventralized mutant.
- https://cdn.elifesciences.org/articles/99263/elife-99263-supp10-v2.xlsx
-
Supplementary file 11
Euclidean distance scores of proteins and phosphosites in DV clusters 1-12.
Empty cell in gene and protein name indicate the detected protein (or protein that hosts a phosphosite) had not been given a gene and/or a protein name in the Uniprot database version used in this study. NaN indicates it was not possible or did not correspond to calculate the Euclidean distance using a particular ventralized mutant.
- https://cdn.elifesciences.org/articles/99263/elife-99263-supp11-v2.xlsx
-
Supplementary file 12
Proteins and phosphoproteins associated with morphogenesis-related cellular components that are significantly-enriched in diffused networks.
- https://cdn.elifesciences.org/articles/99263/elife-99263-supp12-v2.xlsx
-
Supplementary file 13
List of proteins and phosphosites with extreme deviations from the linear model.
- https://cdn.elifesciences.org/articles/99263/elife-99263-supp13-v2.xlsx
-
MDAR checklist
- https://cdn.elifesciences.org/articles/99263/elife-99263-mdarchecklist1-v2.pdf