Biochemical and neurophysiological effects of deficiency of the mitochondrial import protein TIMM50
Figures
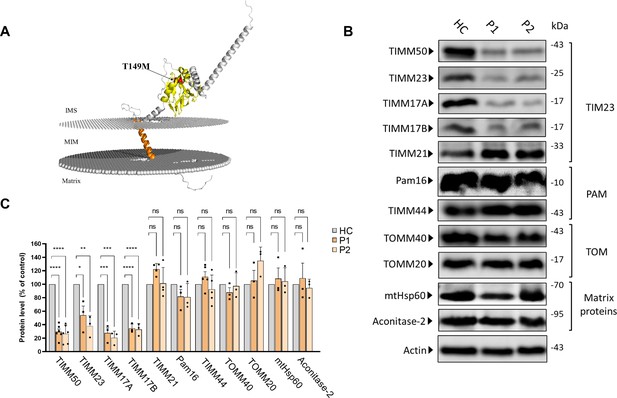
Differential effects of TIMM50 mutation on the expression of TIM23, presequence translocase-associated motor (PAM) and TOM subunits and matrix-destined proteins.
(A) Predicted AlphaFold (Tunyasuvunakool et al., 2021) human TIMM50 structure displaying the position of the T149M mutation. PPM webserver (Lomize et al., 2022) was used to embed the predicted structure into the mitochondrial inner membrane (MIM). Orange – transmembrane domain, Yellow – FCP1-like domain, Red – Threonine 149. (B) Healthy control- and patients-derived primary fibroblasts were lysed and analyzed by immunoblot with the indicated antibodies. Actin was used as the loading control. Full results and original blots are found in Figure 1—source data 1; Figure 1—source data 2. (C) Band density analysis of the blots presented in A and in Figure 1—source data 1; Figure 1—source data 2, showing significant decrease in levels of TIMM50, TIMM23, and TIMM17A/B, but not in TIMM21, PAM subunits, TOM subunits, and matrix TIM23 complex substrates. Band signals were normalized to the loading control and compared to the level measured in the healthy control sample, taken as 100%. Data are shown as means ± SEM, n=6 biological repeats for TIMM50 antibody, n=3–5 biological repeats for all other antibodies, *p-value <0.05, **p-value <0.01, ***p-value <0.001, ****p-value <0.0001, Ordinary one-way ANOVA.
-
Figure 1—source data 1
PDF file containing original immunoblots for Figure 1, indicating the relevant bands and samples running order.
- https://cdn.elifesciences.org/articles/99914/elife-99914-fig1-data1-v1.zip
-
Figure 1—source data 2
Original files for immunoblot analysis are displayed in Figure 1.
- https://cdn.elifesciences.org/articles/99914/elife-99914-fig1-data2-v1.zip
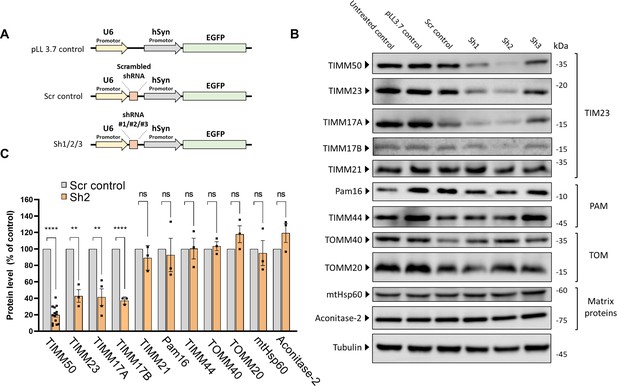
TIMM50 knockdown in mouse primary cortical neurons serves as a model system to study TIMM50 deficiency in mammalian neurons.
(A) Schematic depictions of the different constructs used in this study. ‘pLL3.7 control’ is a control for EGFP expression. ‘scrambled (Scr) control’ is a control for the shRNA system activation with a scrambled Sh3 sequence and Sh1-Sh3 are three plasmids expressing different TIMM50 targeting shRNA sequences. (B) Neuronal cultures were transduced to express the indicated constructs, lysed, and analyzed by immunoblot with the indicated antibodies. Tubulin was used as the loading control. ‘Untreated’ controls are cells that were not transduced. Full results and original blots are found in Figure 2—source data 1; Figure 2—source data 2. (C) Band density analysis of the blots presented in B (and in Figure 2—source data 1; Figure 2—source data 2) shows a significant decrease in levels of TIMM50, TIMM23, and TIMM17A/B, but not in TIMM21, presequence translocase-associated motor (PAM) subunits, TOM subunits, and matrix TIM23 complex substrates. Band signals were normalized to the loading control and compared to the Scr control, taken as 100%. Data are shown as means ± SEM, n=14 biological repeats for TIMM50 antibody, n=3–4 biological repeats for all other antibodies, **p-value <0.01, ****p-value <0.0001, unpaired Student’s t-test.
-
Figure 2—source data 1
PDF file containing original immunoblots for Figure 2, indicating the relevant bands and samples running order.
- https://cdn.elifesciences.org/articles/99914/elife-99914-fig2-data1-v1.zip
-
Figure 2—source data 2
Original files for immunoblot analysis are displayed in Figure 2.
- https://cdn.elifesciences.org/articles/99914/elife-99914-fig2-data2-v1.zip
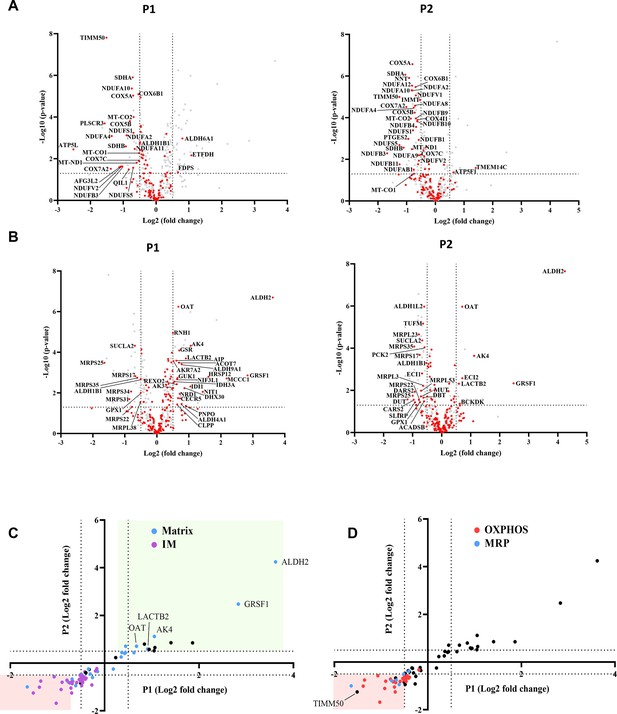
TIMM50 deficiency leads to a specific reduction in the oxidative phosphorylation (OXPHOS) and mitochondrial ribosomal proteins (MRP) machineries but does not affect the majority of mitochondrial inner membrane (MIM) and mitochondrial matrix proteome.
Untargeted mass spectrometry analysis of fibroblasts from both patients (P1, P2), as compared to the healthy control. In the volcano plots displayed in (A, B) and in all subsequent figures, the y-axis cut-off of >1.301 corresponds to –log (0.05) or p-value = 0.05, while the x-axis cut-off of <−0.5 and >0.5 corresponds to a ±1.414 fold change. Each dot in the graph represents a protein. Proteins depicted on the right side of the x-axis cut-off and above the y-axis cut-off were considered to be increased in amount, while proteins depicted on the left side of the x-axis cut-off and above the y-axis cut off were considered to be decreased in amount. All relevant proteins that were increased/decreased in amount are identified by their gene name, next to the dot. Statistical analysis was performed using Student’s t-test and a p-value <0.05 was considered statistically significant. n=9 per group (three biological repeats in triplicate), P1 and P2 results were compared to healthy control (HC) results. Full list of differentially expressed proteins in fibroblasts is found in Files 1-2Source data 1 and 2. (A) The steady-state levels of a majority of MIM proteins detected in patient fibroblasts were not affected. MIM proteins are colored red, while other detected mitochondrial proteins are colored gray. (B) The steady-state levels of a majority of matrix proteins detected in patient fibroblasts were not affected. Matrix proteins are colored red, while other detected mitochondrial proteins are colored gray. (C) Correlation plot comparing the log-fold-change of P1 to that of P2 showing that out of the proteins that were significantly changed for both patients, MIM proteins are consistently downregulated, while matrix proteins are both upregulated and downregulated. (D) Correlation plot comparing the log-fold-change of P1 to that of P2 showing that OXPHOS and MRP proteins comprise nearly all the proteins that were downregulated in both patients.
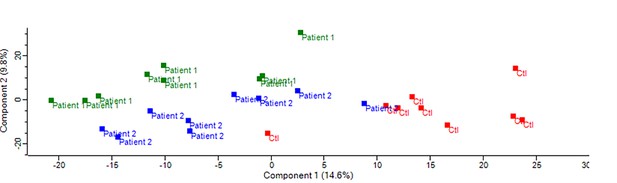
Principal component analysis (PCA) analysis for fibroblasts proteomics replicates.
PCA analysis shows that the proteomics P1 and P2 replicates are consistently different than the healthy control (HC) replicates.

Immunoblot confirmation of mass spectrometry-based proteomics findings for ALDH2 and GRSF1.
Verification of the measured increase in the levels of ALDH2 and GRSF1 in fibroblasts. Three biological repeats were performed for each antibody. Full results and original blots are found in Figure 3—figure supplement 2—source data 1;Figure 3—figure supplement 2—source data 2.
-
Figure 3—figure supplement 2—source data 1
PDF file containing original immunoblots for Figure 3—figure supplement 2, indicating the relevant bands and samples running order.
- https://cdn.elifesciences.org/articles/99914/elife-99914-fig3-figsupp2-data1-v1.zip
-
Figure 3—figure supplement 2—source data 2
Original files for immunoblot analysis are displayed in Figure 3—figure supplement 2.
- https://cdn.elifesciences.org/articles/99914/elife-99914-fig3-figsupp2-data2-v1.zip
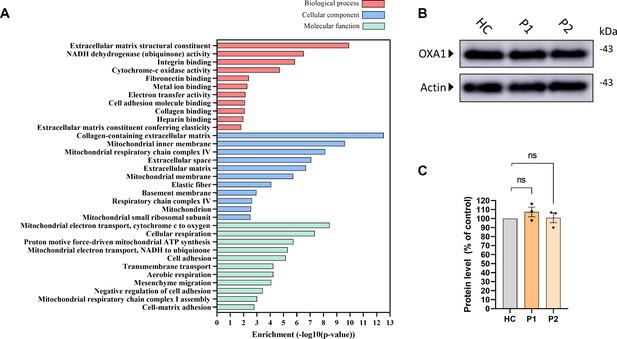
Go-term analysis of all significantly decreased fibroblast proteins and OXA1 immunoblot.
(A) Go-term analysis confirms the enrichment of inner mitochondrial membrane proteins, oxidative phosphorylation (OXPHOS)-related, processes and mitochondrial ribosome subunits. (B) Healthy control- and patients-derived primary fibroblasts were lysed and analyzed by immunoblot with OXA1 antibody. Actin was used as the loading control. (C) Band density analysis shows no significant change in the levels of OXA1. Band signals were normalized to the loading control and compared to the level measured in the healthy control sample, taken as 100%. Data are shown as means ± SEM, n=3 biological repeats. Full results and original blots are found in Figure 3—figure supplement 3—source data 1 and 2.
-
Figure 3—figure supplement 3—source data 1
PDF file containing original immunoblots for Figure 3—figure supplement 3, indicating the relevant bands and samples running order.
- https://cdn.elifesciences.org/articles/99914/elife-99914-fig3-figsupp3-data1-v1.zip
-
Figure 3—figure supplement 3—source data 2
Original files for immunoblot analysis are displayed in Figure 3—figure supplement 3.
- https://cdn.elifesciences.org/articles/99914/elife-99914-fig3-figsupp3-data2-v1.zip
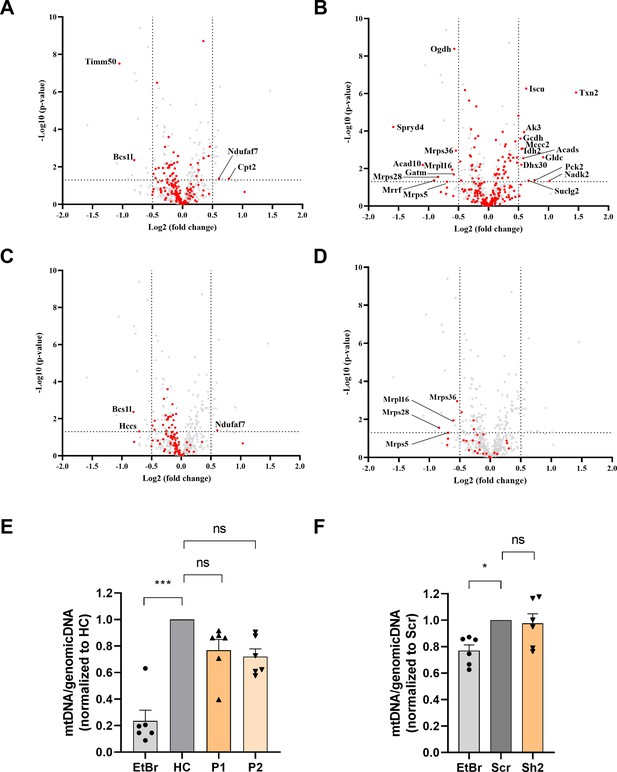
The impact of TIMM50 deficiency on the mitochondrial proteome in neurons.
Untargeted mass spectrometry analysis of mice primary neurons transduced with either Sh2 or the scrambled (Scr) control. n=9 per group (three biological repeats in triplicates). Full list of differentially expressed proteins in neurons is found in Source data 3. (A) The steady-state levels of a majority of mitochondrial inner membrane (MIM) proteins detected in TIMM50 knockdown (KD) neuronal cells were not affected. MIM proteins are colored red, while other detected mitochondrial proteins are colored gray. (B) The steady-state levels of a majority of matrix proteins detected in TIMM50 KD neuronal cells were not affected. Matrix proteins are colored red, while other detected mitochondrial proteins are colored gray. (C) Most OXPHOS subunits are observed to be in decreased amounts in TIMM50 KD neuronal cells, albeit at a lower extend from what was observed in patient fibroblasts. OXPHOS proteins are colored red, while other detected mitochondrial proteins are colored gray. (D) Decreased steady-state levels of multiple mitochondrial ribosomal protein (MRP) subunits were observed in TIMM50 KD neuronal cells. MRP proteins are colored red, while other detected mitochondrial proteins are colored gray. (E, F) Mitochondrial DNA content in fibroblasts (E) and neurons (F) was not affected in TIMM50 deficient cells compared to control cells. Mitochondrial DNA content was estimated by measuring the ratio between mitochondrial and nuclear DNA. Dloop1 expression was measured by qPCR relative to hypoxanthine guanine phosphoribosyl transferase (HPRT); TERT served as a control of a nuclear-encoded gene. Ethidium bromide (EtBr; 100 ng/ml) was used as the positive control. Data are shown as means ± SEM, n=6 (samples from three biological replicates, each performed twice), *p-value <0.05, ***p-value <0.001, Kruskal-Wallis test.
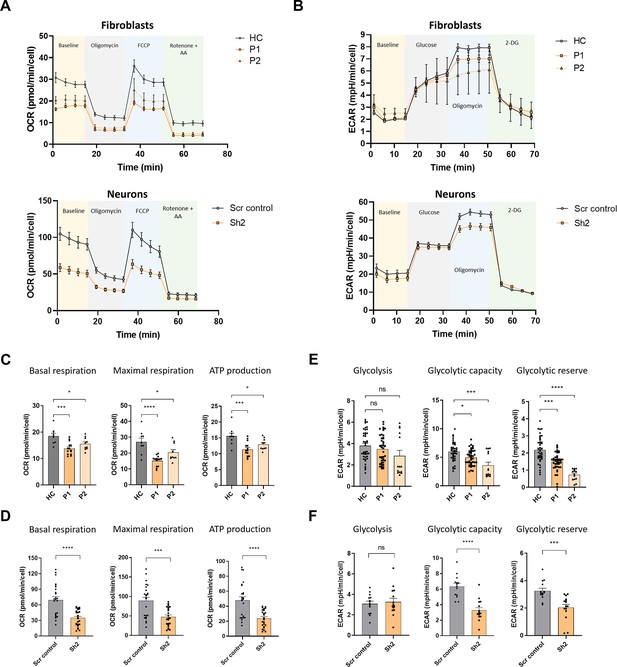
TIMM50 deficiency negatively impacts oxidative phosphorylation (OXPHOS) machinery and glycolysis functions.
(A) A Seahorse XF cell mito-stress assay was used to measure mitochondrial oxygen consumption rates at basal levels and in response to the indicated effectors in fibroblasts (upper panel) and neuronal cells (lower panel). (B) A Seahorse XF cell glycolysis stress assay was used to measure glycolysis at basal levels and in response to the indicated effectors in fibroblasts (upper panel), and neuronal cells (lower panel). (C) Basal respiration, maximal respiration, and ATP-linked respiration were reduced in patient fibroblast cells compared to healthy control (HC) cells. Data are shown as means ± SEM, *p-value <0.05, ***p-value <0.001, ****p-value <0.0001, Ordinary one-way ANOVA. (D) Basal respiration, maximal respiration and ATP-linked respiration were reduced in TIMM50 KD neuronal cells compared to scrambled (Scr) control-transduced neuronal cells. Data are shown as means ± SEM, ***p-value <0.001, ****p-value <0.0001, unpaired Student’s t-test. (E) Basal glycolysis remained similar, while glycolytic capacity and glycolytic reserves were reduced in patient fibroblast cells compared to HC cells. Data are shown as means ± SEM, *p-value <0.05, ***p-value <0.001, ****p-value <0.0001, Kruskal-Wallis test. (F) Basal glycolysis remained similar, while glycolytic capacity and glycolytic reserves were reduced in TIMM50 KD neuronal cells compared to Scr control-transduced neuronal cells. Data are shown as means ± SEM, ***p-value <0.001, ****p-value <0.0001, unpaired Student’s t-test. For all the experiments shown in (A–F) n=4–6 biological repeats of three to six technical repeats each.
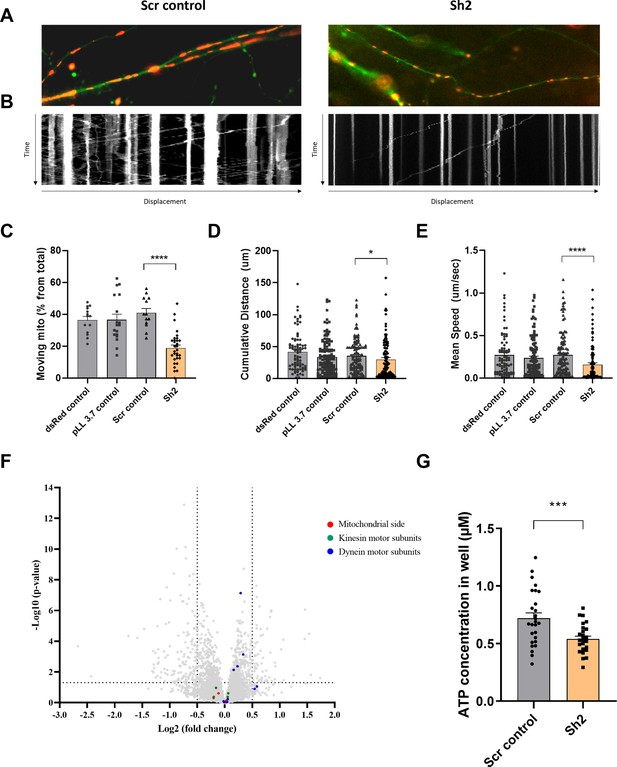
TIMM50 deficiency leads to defective mitochondrial trafficking along neuronal processes.
(A) Representative images of neuronal processes in neuronal cultures that were co-transfected with a dsRed-mito plasmid and either a scrambled (Scr) control plasmid (left panel) or a TIMM50 knockdown (KD) plasmid (right panel). (B) Kymographs of the same processes in A, shows the displacement of mitochondria over time. The y-axis length is 5 min, and x-axis length is about 100 μm. (C) Lower percentage of moving mitochondria was observed in TIMM50 KD neuronal processes compared to controls. Each dot in the graph represents the percentage of moving mitochondria out of the total observed mitochondria in a single neurite. Data are shown as means ± SEM, n=13–31 neurites per condition, analyzed from three biological repeats, ****p-value <0.0001, Ordinary one-way ANOVA. (D) Decreased mitochondrial cumulative travelling distance was observed in TIMM50 KD neuronal cells compared to controls. Each dot in the graph represents a single moving mitochondrion. n=84–130 mitochondria per condition, analyzed from three biological repeats, *p-value <0.05, Kruskal-Wallis test. (E) Slower mitochondrial movement was observed in TIMM50 KD neuronal cells compared to controls. Each dot in the graph represents a single moving mitochondrion. n=84–130 mitochondria per condition, analyzed from three biological repeats, ****p-value <0.0001, Kruskal-Wallis test. (F) No change in mitochondrial trafficking proteins was observed in TIMM50 KD neuronal cells. The y-axis cut-off of >1.301 corresponds to –log (0.05) or p-value = 0.05, while the x-axis cut-off of <−0.5 and >0.5 corresponds to a ±1.414 fold change. Each dot in the graph represents a protein. Proteins depicted on the right side of the x-axis cut-off and above the y-axis cut-off were considered to be increased in amount, while proteins depicted on the left side of the x-axis cut-off and above the y-axis cut-off were considered to be decreased in amount. Statistical analysis was performed using Student’s t-test and a p-value <0.05 was considered statistically significant. n=9 per group (three biological repeats in triplicate). Full list of differentially expressed proteins in neurons is found in Source data 3. (G) Lower cellular ATP levels were observed in TIMM50 KD neuronal cells compared to Scr control-transduced cells. Data are shown as mean ± SEM, n=27 quantified wells (each containing 5 × 104 cells) per condition, from three biological repeats with nine technical repeats each, ***p-value <0.001, unpaired Student’s t-test.
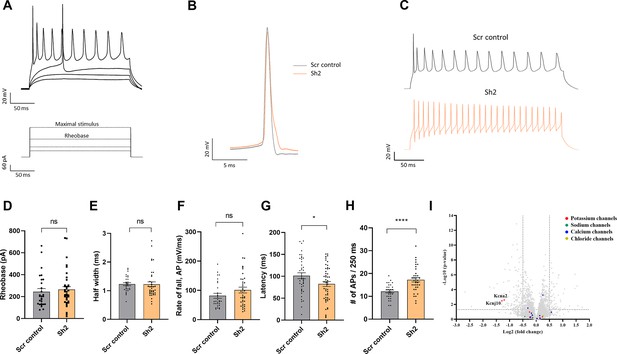
TIMM50 deficiency leads to a significant decrease in the levels of KCNA2 and KCNJ10 potassium channels and an increased electrical activity.
(A) Example of voltage traces in response to increased depolarization of TIMM50 knockdown (KD) neurons. The bottom panel shows the protocol applied, while the top panel shows the typical response pattern that was measured. (B) Representative traces of a single action potential, received at rheobase level, from a TIMM50 KD neuron, as compared to Scr control-transduced neuron. (C) Representative traces of the maximal stimulus for each condition, showing the maximal amount of action potentials measured for each group. (D) No change in rheobase was observed in TIMM50 KD neuronal cells, as compared to scrambled (Scr) control-transduced cells. Rheobase was measured as the first current step that caused the firing of an action potential. Data are shown as means ± SEM, n=28/37 cells (for Scr control-/-Sh2-transduced cells, respectively) from three biological repeats, Mann-Whitney test. (E) No change in the action potential half-width was observed in TIMM50 KD neuronal cells, as compared to Scr control-transduced cells. Half-width was measured as the time between the rising and falling phases of the action potential, at the half-point between the tip of the peak and the bottom of the voltage rising curve. Data are shown as means ± SEM, n=28 / 37 cells (for Scr control-/ Sh2-transduced cells, respectively) from three biological repeats, Mann-Whitney test. (F) No change in the action potential rate of fall was observed in TIMM50 KD neuronal cells, as compared to Scr control-transduced cells. Rate of fall was measured as the time between the action potential peak and the baseline following the action potential, divided by the change in voltage between the same two points (ΔX/ΔY). Data are shown as means ± SEM, n=28/37 cells (for Scr control/Sh2, respectively) from three biological repeats, Mann-Whitney test. (G) Decreased action potential latency was observed in TIMM50 KD neuronal cells compared to Scr control-transduced cells. Latency was measured as the time difference between the beginning of the pulse to the peak of the action potential. Each dot in the graph represents the average latency of the first five consecutive action potentials appearing after the rheobase level. Data are shown as means ± SEM, n=40/50 cells (for Scr control-/Sh2-transduced cells, respectively) from four biological repeats, *p-value <0.05, unpaired Student’s t-test. (H) An increase in the maximal number of action potentials fired in a single stimulus was observed in TIMM50 KD neuronal cells, as compared to Scr control-transduced cells. Data are shown as means ± SEM, n=28/37 cells (for Scr control-/Sh2-transduced cells, respectively) from three biological repeats, ****p-value <0.0001, unpaired Student’s t-test. (I) Amongst the detected ion channel proteins, a specific decrease in KCNA2 and KCNJ10 potassium channels was observed in TIMM50 KD neuronal cells. The y-axis cut-off of >1.301 corresponds to –log (0.05) or p-value = 0.05, while the x-axis cut off of <−0.5 and >0.5 corresponds to a ±1.414 fold change. Each dot in the graph represents a protein. Proteins depicted on the right side of the x-axis cut-off and above the y-axis cut-off were considered to be increased in amount, while proteins depicted on the left side of the x-axis cut-off and above the y-axis cut-off were considered to be decreased in amount. Statistical analysis was performed using Student’s t-test and a p-value <0.05 was considered statistically significant. n=9 per group (three biological repeats in triplicate). Full list of differentially expressed proteins in neurons is found in Source data 3.
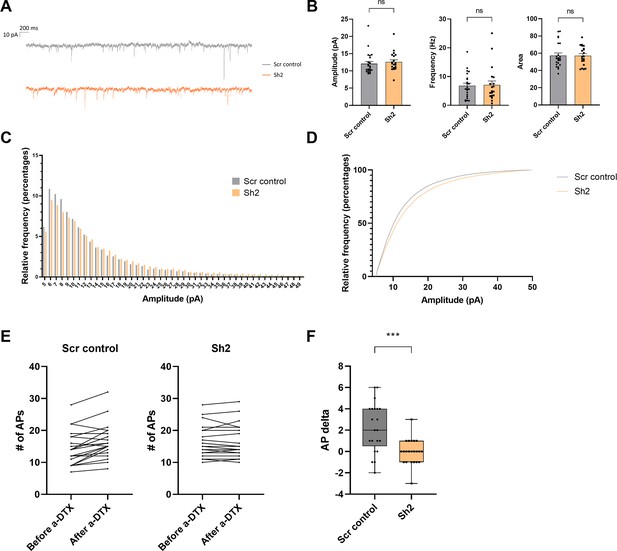
Spontaneous excitatory activity recordings and the effect of α-dendrotoxin (α-DTX) on TIMM50 knockdown (KD) neuronal cells.
(A) Representative traces of miniature excitatory post-synaptic currents (mEPSCs) measurements taken in the presence of 1 μM tetrodotoxin (TTX). (B) No change in the average mEPSC amplitude, frequency, and area under the peak was observed in TIMM50 KD neuronal cells compared to scrambled (Scr) control-transduced cells. Data are shown as means ± SEM, n=23/20 cells (for Scr control-/Sh2-transduced cells, respectively) from three biological repeats, Mann-Whitney test. (C) Histogram of the relative frequency distribution of the mEPSC amplitude measurements. (D) Cumulative distribution function of the mEPSC amplitude measurements. (E) Measurements of the number of action potentials fired before and after treatment with 100 nM α-DTX for Scr control- and Sh2-transduced neuronal cells. (F) A higher difference in the number of action potentials fired after and before α-DTX application was observed in Scr control-transduced cells compared to TIMM50 KD neuronal cells. n=21/20 cells (for Scr control-/Sh2-transduced cells, respectively) from three biological repeats, ***p-value <0.001, unpaired Student’s t-test.
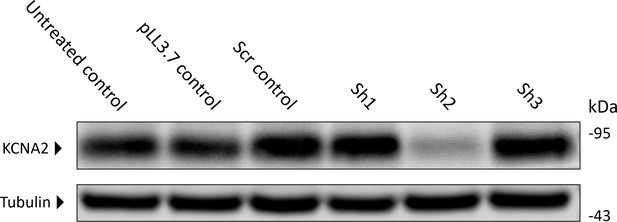
Immunoblot confirmation of mass spectrometry-based proteomics findings for KCNA2.
Verification of the measured decrease in the levels of the KCNA2 potassium channel in neuronal cultures. Three biological repeats were performed. Full results and original blots are found in Figure 7—figure supplement 2—source data 1;Figure 7—figure supplement 2—source data 2.
-
Figure 7—figure supplement 2—source data 1
PDF file containing original immunoblots for Figure 7—figure supplement 2, indicating the relevant bands and samples running order.
- https://cdn.elifesciences.org/articles/99914/elife-99914-fig7-figsupp2-data1-v1.zip
-
Figure 7—figure supplement 2—source data 2
Original files for immunoblot analysis are displayed in Figure 7—figure supplement 2.
- https://cdn.elifesciences.org/articles/99914/elife-99914-fig7-figsupp2-data2-v1.zip
Additional files
-
Supplementary file 1
Oligonucleotides were used in this study.
- https://cdn.elifesciences.org/articles/99914/elife-99914-supp1-v1.docx
-
Supplementary file 2
Reagents and tools used in this study.
- https://cdn.elifesciences.org/articles/99914/elife-99914-supp2-v1.docx
-
Supplementary file 3
Representative mitochondrial trafficking movies.
Scr control neurons and TIMM50 knockdown (KD) neurons co-transfected with the corresponding control/KD plasmid and mito-DsRed plasmid. The cells were imaged for 5 min with a 3 s interval between each image. The videos displayed are sped up by ~15x.
- https://cdn.elifesciences.org/articles/99914/elife-99914-supp3-v1.zip
-
MDAR checklist
- https://cdn.elifesciences.org/articles/99914/elife-99914-mdarchecklist1-v1.docx
-
Source data 1
Healthy control (HC) vs P1 all proteins fold change.
- https://cdn.elifesciences.org/articles/99914/elife-99914-data1-v1.xlsx
-
Source data 2
Healthy control (HC) vs P2 all proteins fold change.
- https://cdn.elifesciences.org/articles/99914/elife-99914-data2-v1.xlsx
-
Source data 3
Scrambled (Scr) vs Sh2 all proteins fold change.
- https://cdn.elifesciences.org/articles/99914/elife-99914-data3-v1.xlsx