MOF-associated complexes ensure stem cell identity and Xist repression
Figures
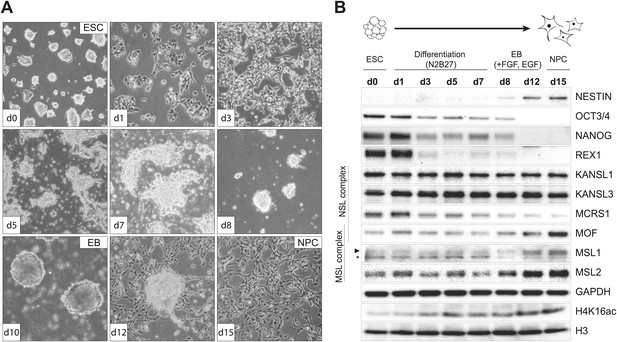
Distinct dynamics of MOF, MSL and NSL complexes during differentiation from ESCs to NPCs.
(A) We monitored the cell morphology during differentiation of mouse embryonic stem cells into neuronal progenitor cells (NPC) via embryoid body formation (EB) with bright field microscopy. The day of differentiation is indicated in white boxes. (B) Western blot analysis for ESC to NPC differentiation. Stages of differentiation together with the day of differentiation (d0–d15) are indicated on top. GAPDH and histone 3 (H3) were used as loading controls. For expression analysis see Figure 1—figure supplement 1.
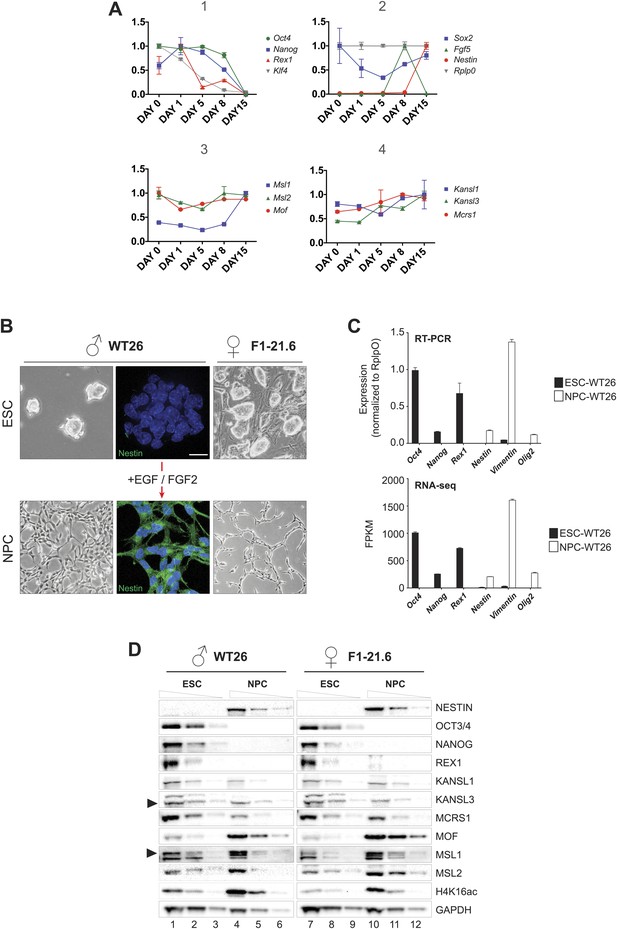
Monitoring RNA and protein levels in ESCs and NPCs.
(A) We monitored the expression dynamics during ESC differentiation for markers of pluripotency (Oct4, Nanog, Rex1, Klf4), embryoid body formation (Fgf5), differentiation (Sox2), and NPC (Nestin). Panels 3 and 4 contain the expression profiles for members of the MSL complex (Msl1, Msl2), Mof, and the NSL complex (Kansl1, Kansl3, Mcrs1), respectively. All results are represented as relative values individually normalized to Rplp0 expression levels (panel 2) on a given day and to the highest expression level of a given gene during the entire differentiation process (highest expression level of each gene = 1). The x-axes show days of differentiation. All results are expressed as means ± SD for technical replicates. For primers see Supplementary file 3C. (B) Bright field images illustrate the cell morphology before and after the process of differentiation. The immunofluorescence analysis indicates the specific staining for the NESTIN (green) in neuronal progenitors (NPC); DNA is counterstained with DAPI (blue). (C) Expression changes for selected ESC-specific and NPC-specific markers before and after differentiation of wild-type WT26 cells using RT-PCR analysis and RNA-seq. (D) Western blots for proteins from two ES cell lines and their NPC derivatives. Different dilutions were loaded (100%, 30%, 10%) with the order indicated on top of the blots. Anti-GAPDH was used as loading control; arrows indicate the protein of interest.
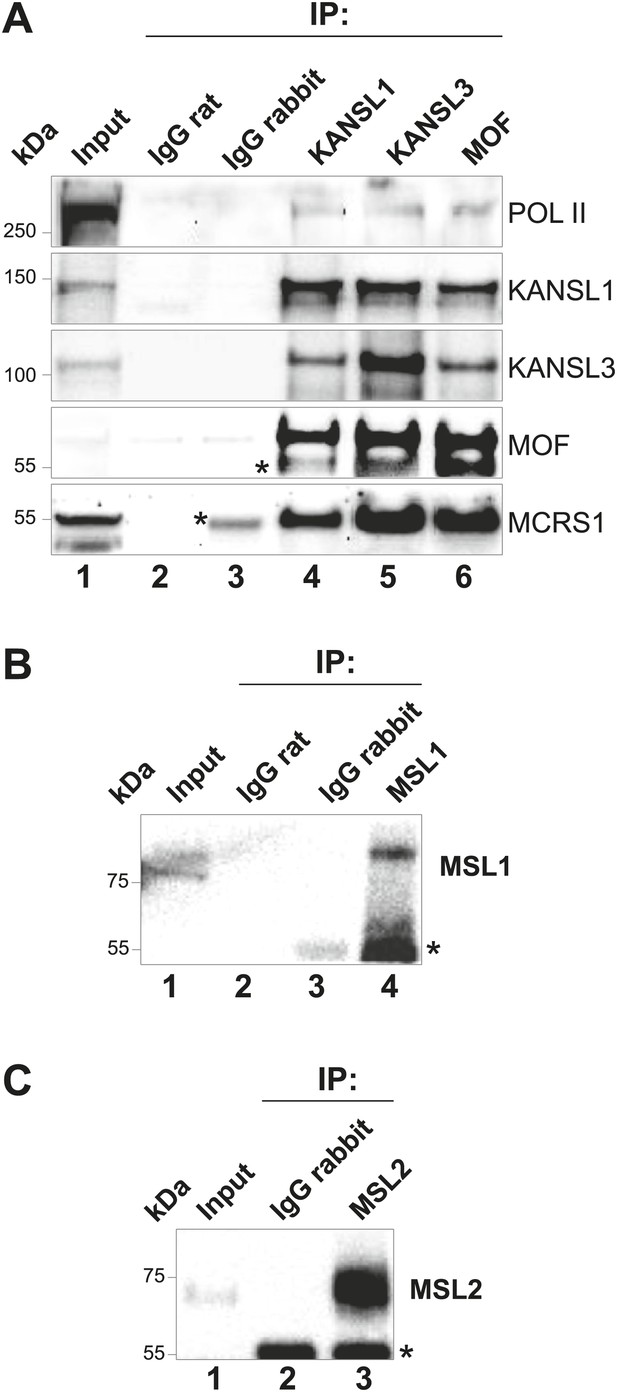
Verification of antibodies used in this study.
(A) Immunoprecipitations from ESC nuclear extracts with antibodies specific for KANSL1, KANSL3 or MOF, and rabbit or rat antisera. The blot was probed with indicated antibodies showing the co-immunoprecipitation of several NSL complex members. Pol II = RNA Polymerase II. (B) and (C) same as (A) except that immunoprecipitations were performed with antibodies specific to MSL1 (B) and MSL2 (C). Asterisks represent the IgG signal.
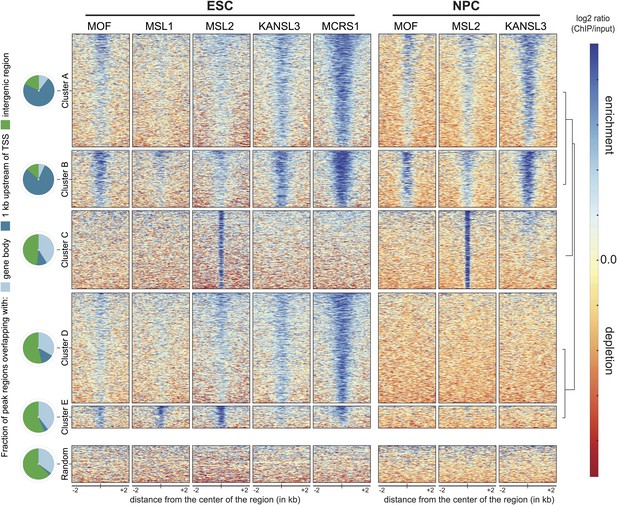
Distinct and shared binding sites of MOF and its complexes in mouse ESCs and NPCs.
We applied unsupervised clustering on the union of peaks from all ChIP-seq samples and thereby identified five distinct groups of binding for MOF, MSL and NSL proteins in ESCs and NPCs. Shown here are the input-normalized ChIP signals for each cluster of peaks including a size-matched control set of random genomic regions. The order of the regions is the same for all columns. The pie charts on the left indicate the number of regions from each cluster that overlap with gene bodies, the region 1 kb upstream of genes' TSS or intergenic regions.
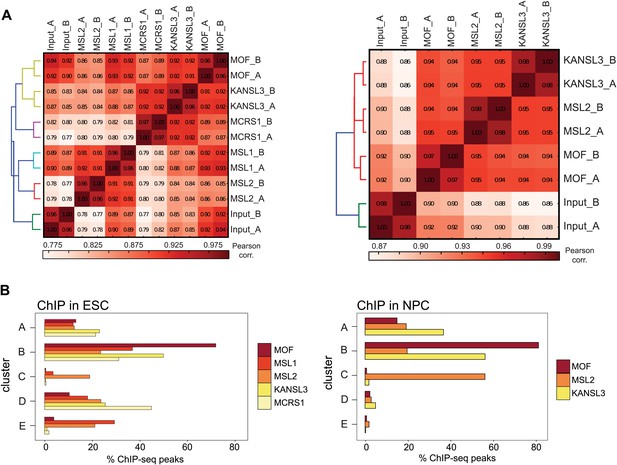
ChIP-seq quality measures.
(A) Correlation plot for all individual ChIP-seq and input samples from ESCs (left) and NPCs. The genome was sampled in windows of 10 kb length; the numbers of reads per bin were counted for each ChIP sample and correlated using Pearson correlation. The calculation and heatmap visualization were done with the bamCorrelate module from the deepTools suite (Ramirez et al., 2014). (B) The bar chart depicts the fraction of ChIP-seq peaks for each protein that reside within each cluster shown in Figure 2, that is approximately 30% of MSL1 peaks in ESCs locate in cluster E. Note that the absolute numbers of peaks differ between the samples (see Supplementary file 1B for absolute peak numbers and ‘Materials and methods’ for peak calling details).
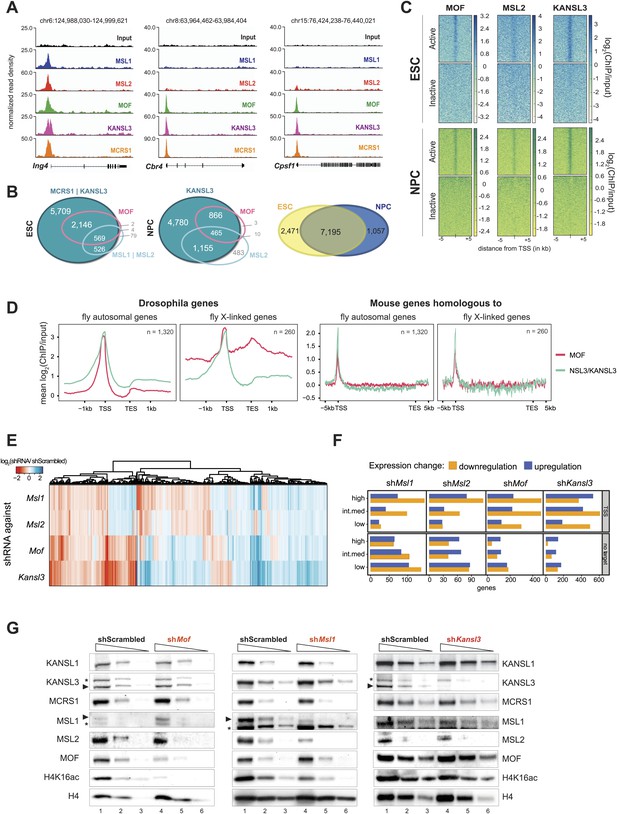
Both MOF-complexes bind to the TSS of broadly expressed genes in mouse ESCs and NPCs.
(A) Genome browser snapshots of genes targeted by MSL and NSL complexes or by the NSL complex only. Signals were sequencing-depth-normalized and from ESCs. For ChIP-qPCR-based validation of the signals see Figure 3—figure supplement 4B. (B) Venn diagrams of genes whose promoter regions (TSS ± 500 bp) overlapped with ChIP-seq peaks of NSL complex members (KANSL3 and/or MCRS1), MOF and MSL complex members (MSL1 and/or MSL2). The right-most panel depicts the overlap of genes bound by at least one factor in ESCs and NPCs. (C) The heatmaps display the input-normalized ChIP enrichments of MOF, MSL2 and KANSL3 around the TSS of genes that were active in ESCs as well as NPCs based on RNA-seq data that we generated for both cell types. (D) Summary plots of genes bound by the NSL complex in D. melanogaster for which mouse homologues were found. The input-normalized ChIP-seq signals around the TSS reveal markedly increased binding of MOF for male X-linked fly genes (left panels) that was not recapitulated in the mouse (right panels; ChIP-seq signals from ESCs). Fly genes were scaled to 1.2 kb and values were extracted from published data sets, mouse genes were scaled to 30 kb. (E) Heatmap depicting results of RNA-seq experiments from different shRNA-treated cells. The colors correspond to log2 fold changes (shRNA-treated cells/scrambled control) for genes whose expression was significantly affected in all knockdown conditions. Values were ordered using hierarchical clustering. (F) Bar plot of gene counts for different gene classes. We determined significantly up- and downregulated genes for each knockdown condition and binned them according to their expression strength in wild-type ESCs (high, intermediate, low). Then, for each gene, information about the TSS-targeting was extracted from the corresponding ChIP-seq sample. Non-target genes are neither bound at the promoter nor the gene body and were not predicted to be regulated via TSS-distal binding sites in any of the 5 ChIP-seq ESC samples. For details on the target classification see ‘Materials and methods’. (G) Western blot analysis of MSL and NSL complex members and H4K16 acetylation in scrambled-, Mof-, Msl1-, and Kansl3-shRNA-treated male ESCs. Three concentrations (100%, 30%, 10%) of RIPA extract were loaded per sample. Asterisks mark the position of unspecific bands; triangles indicate the protein of interest.
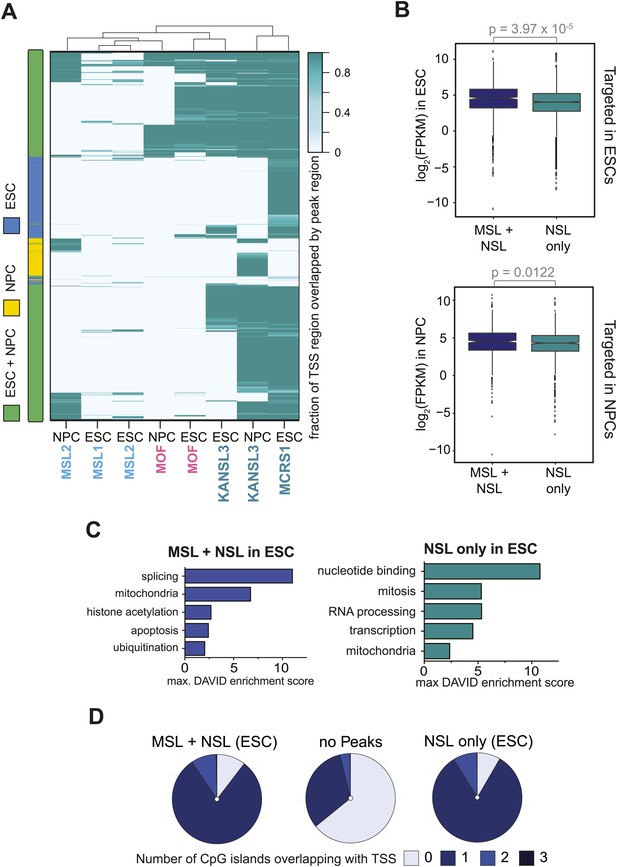
MSL and NSL complexes target promoters of broadly expressed genes in ESCs and NPCs.
(A) The heatmap is related to Figure 3B as it is based on all genes that are bound by at least 1 ChIPed factor in ESCs or NPCs. The intensity of the color depicts the fraction of the 1 kb TSS-region that was covered by a binding site of MOF, MSL1, MSL2, KANSL3 or MCRS1. Rows and columns were sorted using hierarchical clustering on the Euclidean distances of the overlap fractions using R. The left color bar indicates which genes are targeted in 1 or both cell types. (B) Distribution of expression values from RNA-seq data in ESCs and NPCs for genes targeted by MSL and NSL complex members together or by the NSL complex only. p-values were calculated using Welch t test. (C) Results of the GO term analysis using DAVID (Huang da et al., 2009) on genes that were bound at the TSS in ESCs by NSL complex members only or both MSL and NSL complexes. (D) The pie charts depict how many times annotated TSSs overlapped with a CpG island. The vast majority of genes that were bound in ESCs by MSL and NSL together or by NSL complex members alone overlapped with at least 1 CpG island (dark and medium blue) while approximately 2/3 of the non-target-TSS did not overlap with any CpG island (light blue for 0 CpG islands within the queried regions).
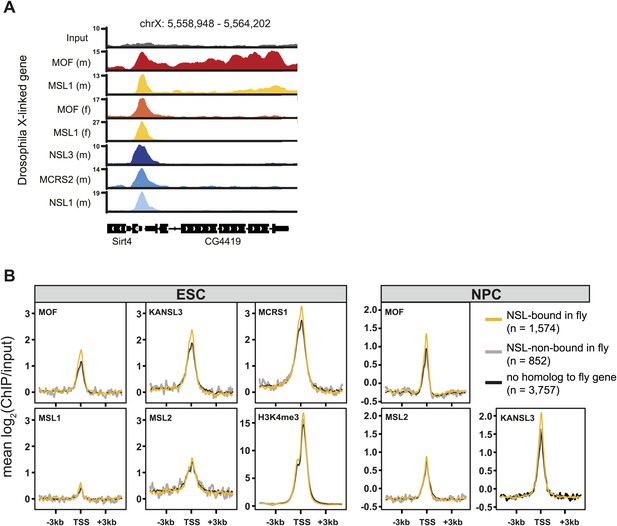
The NSL-, but not the MSL-binding mode of D. melanogaster is present in mammalian cells.
(A) Exemplary genome browser snapshots of the X-linked fly gene CG4419. Shown here are the sequencing-depth normalized profiles for ChIP and corresponding input samples, clearly showing a broad enrichment of MOF and MSL1 along the entire gene body in male (m) D. melanogaster while all other marks show sharp enrichments around the TSS (including MSL1 and MOF in female (F) D. melanogaster) which are similar to those seen for both complexes in mouse cells (Figure 3A,D). (B) Comparison of expressed (FPKM >4) mouse genes whose homologous genes are either bound or not bound by MOF and its complexes in the fly. We extracted the input-normalized ChIP-seq values for 6 kb regions around the TSS using the computeMatrix module of deepTools (Ramirez et al., 2014). H3K4me3 signal is from a published data set, see Supplementary file 2 for the corresponding accession number.
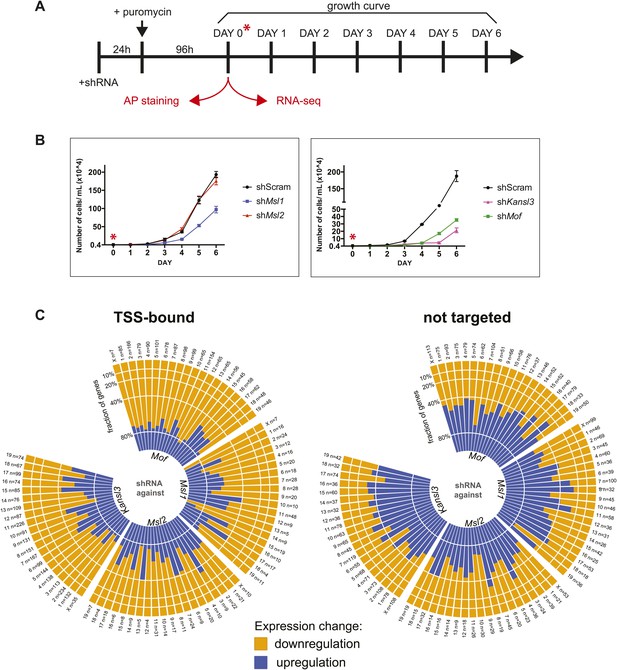
Effects of shRNA-mediated depletion of MOF, MSL1, MSL2 and KANSL3.
(A) Time course of knockdown experiments. For experimental details see ‘Materials and methods’. Samples for RNA-sequencing and AP staining (Figure 4—figure supplement 4) were extracted 4 days after puromycin selection of shRNA-treated cells. (B) Proliferation assay for shRNA-treated cells, starting at day 4 after puromycin selection (Figure 3—figure supplement 3A). (C) Bar plots depicting the fractions of genes (per chromosome) that were significantly up- or downregulated in RNA-seq experiments from shRNA-treated cells. The left plot contains genes which were defined as TSS-targets in the respective ChIP-seq samples, the right plot contains genes that were neither classified as TSS- nor as TSS-distal targets. The labels on each bar indicate the chromosome name and the total number of genes that fulfilled the criteria for this chromosome (significantly affected, TSS-bound or non-targeted). See ‘Materials and methods’ for details of the classification.
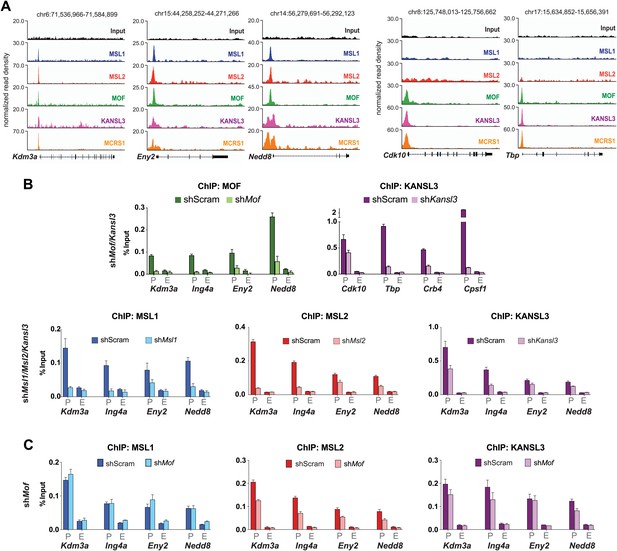
Assessment of ChIP signals around the TSSs of putative target genes as determined by ChIP-seq.
(A) Genome Browser snapshots of several MSL/NSL (first three from the left) or NSL-only target genes and respective sequencing-depth-normalized ChIP-seq and input signals from ESCs. The exact genomic coordinates are indicated on top of each panel. Gene names are indicated on the bottom. (B) ChIP-qPCR validation for MOF (green), KANSL3 (purple), MSL1 (blue) and MSL2 (red) signals. Immunoprecipitated DNA was amplified by qPCR with primer sets positioned at the promoter (P) and end (E) of the coding sequence (Supplementary file 3A). Results are expressed as mean ± SD of three biological replicates; cells were harvested for experiments on day 4 (Kansl3, Msl1, Msl2) or 5 (Mof) of knockdown. (C) ChIP-qPCR for MSL1 (blue), MSL2 (red) and KANSL3 (purple) in ESCs treated with sh-RNA (scrambled or shMof). Signals on genes were evaluated using primers at the promoter (P), and end (E) of the coding sequence. Results are expressed as mean ± SD of three biological replicates; cells were harvested for experiments on day 5 of Mof knockdown.
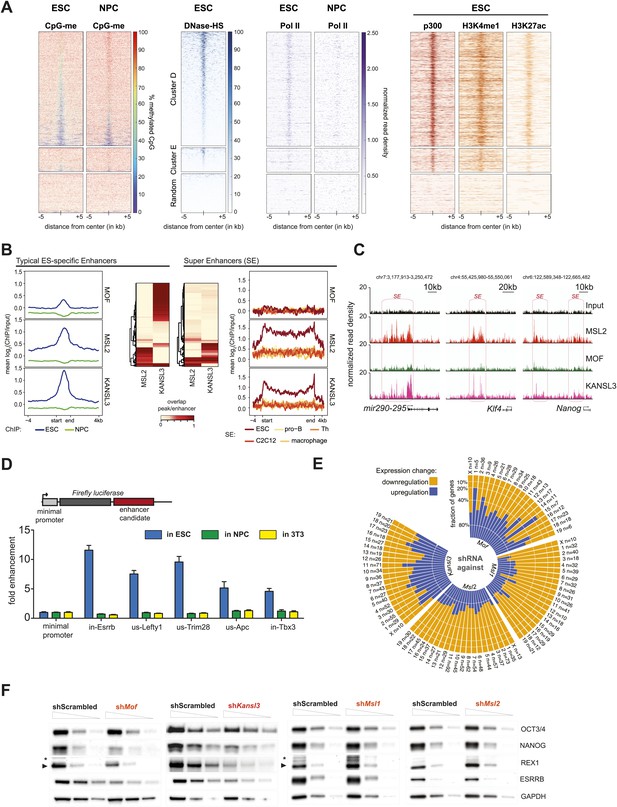
MSL and NSL complex members are enriched at regions with enhancer marks in ESCs.
(A) Shown here are the fractions of methylated cytosines and ChIP-seq read densities of enhancer markers for regions of ESC-specific enrichments of our proteins of interest. We downloaded the different data from public repositories (see Supplementary file 3A for details) and calculated the values for the regions of the ESC-specific clusters D and E and random genomic loci. Most data sets used here were from mouse ESC except one RNA Polymerase II (Pol II) sample from NPC. All heatmaps were sorted according to the DNase hypersensitivity values except for CpG methylation heatmaps which were sorted according to their own values. (B) Summary plots of input-normalized ChIP-seq signals along typical (TE) and super enhancers (SE) (Whyte et al., 2013). Note that we show the ESC-specific TE only while on the right-hand side we show the signal for SE regions from several cell types. Enhancer regions were scaled to 30 kb (SE) and circa 700 bp (TE). The heatmaps between the summary plots depict how much of each enhancer region overlaps with ChIP-seq peaks of MSL2 or KANSL3. ESC = embryonic stem cells (n = 232), pro-B = progenitor B cells (n = 396), Th = T helper cells (n = 437), C2C12 = myotube cells (n = 536). (C) Exemplary genome browser snapshots of annotated super enhancers (SE, pink boxes) for three pluripotency factors displaying the sequencing-depth normalized ESC ChIP-seq signals of MSL2, MOF and KANSL3. See Figure 4—figure supplement 4C for additional examples. (D) Luciferase assays demonstrate the biological activity of regions bound by MOF-associated proteins in ESCs (‘in’ stands for intronic region, ‘us’ indicates that the cloned region is upstream of the gene). The firefly luciferase gene was cloned under a minimal promoter together with the putative enhancer region in ESCs, NPCs, and 3T3 cells. The graphs represent at least three independent experiments performed in technical triplicates; error bars represent SEM. (E) Bar plots depicting the fraction of significantly up- and downregulated genes per chromosome in the different shRNA-treated cells compared to shScrambled controls (total number of significantly affected genes per sample and chromosome labels are indicated). All genes counted here were classified as TSS-distal target genes in the respective ChIP-seq experiments. See ‘Materials and methods’ for details of the classifications. (F) Western blot analyses of the pluripotency factors in scrambled-, Mof-, Kansl3-, Msl1-, and Msl2-shRNA-treated male ESCs. For additional analyses in female ESCs see Figure 6C. The respective dilution (100%, 30%, 10%) of loaded RIPA extract is indicated above each panel. Asterisks mark the position of unspecific bands; triangles indicate the protein of interest. GAPDH was used as the loading control. For antibodies see ‘Materials and methods’.
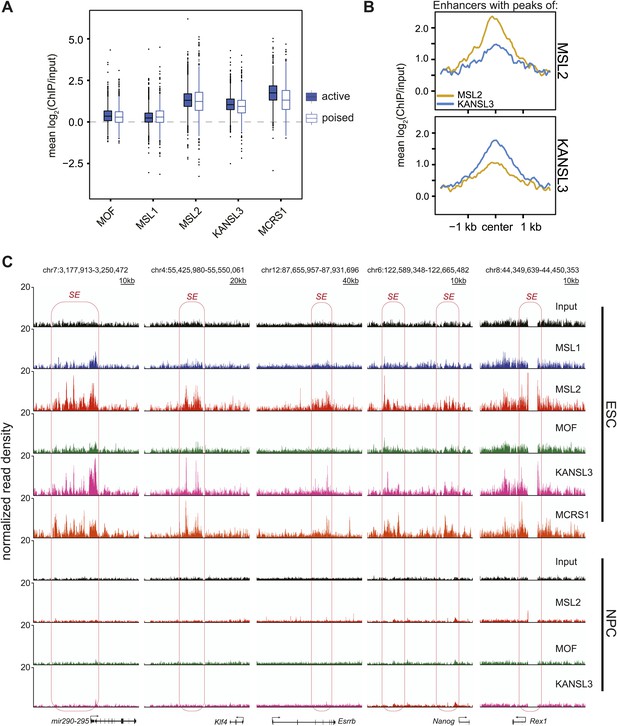
MSL2 and KANSL3 show strong enrichments at typical and super enhancers in ESCs.
(A) Boxplots demonstrating the distribution of mean ChIP enrichments for enhancer regions defined by H3K4me1 and H3K27ac marks in ESCs (see Creyghton et al., 2010 for details) that overlap with the clusters of binding defined by our ChIP-seq samples. Mean values were extracting using the UCSCtool bigWigAverageOverBed. (B) Summary plots for typical enhancer regions (Whyte et al., 2013) that overlapped with either MSL2 (top) or KANSL3 (bottom) peaks. Different colors indicate different ChIP-seq signals. Related to the heatmaps of Figure 4B. (C) Genome browser snapshots of sequencing-depth normalized ChIP-seq and input profiles for super enhancers of key pluripotency factors.
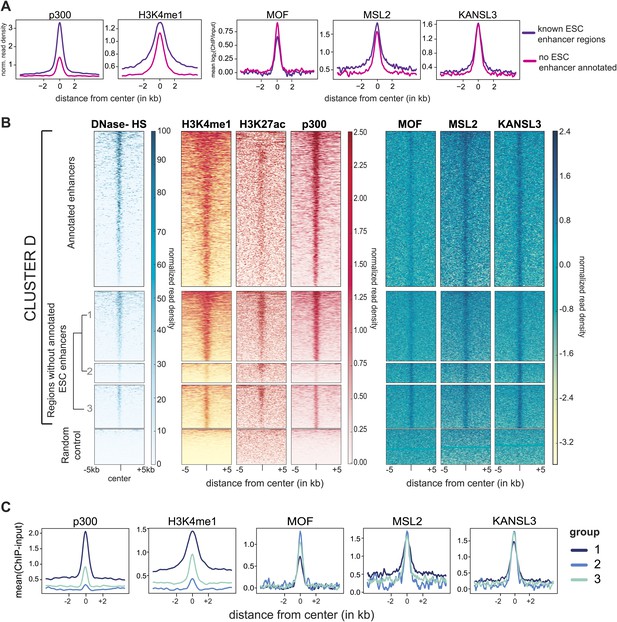
MOF is moderately enriched at non-canonical enhancers.
(A) Summary plots of ChIP-seq values for binding sites belonging to cluster D. The regions were divided based on the presence or absence of annotated ESC enhancers (Creyghton et al., 2010; Whyte et al., 2013). (B) Heatmaps of ChIP-seq read densities of known enhancer markers for the ESC-specific binding sites of our proteins of interest (cluster D, see Figure 2) and random genomic regions. The binding sites of cluster D (excluding regions with TSSs) were divided into two basic groups based on the presence or absence of known ESC enhancers (Creyghton et al., 2010; Whyte et al., 2013). The latter group was further divided into three (arbitrarily numbered) sub-clusters based on hierarchical clustering of the values from DNase hypersensitivity sites, p300, H3K4me1 and our MOF sample (in ESCs). Heatmaps of the ESC-enhancer-containing regions were sorted according to p300, those of the sub-clustered regions were sorted according to the MOF signal. (C) Related to (B), shown here are the corresponding summary plots of ChIP-seq values for cluster D binding sites that do not overlap with annotated enhancer regions (bottom part of the heatmaps in the figure above). The three indicated groups are based on the hierarchical clustering that was performed on p300, H3K4me1 and MOF values (‘Regions without annotated ESC enhancers’ in (B)).
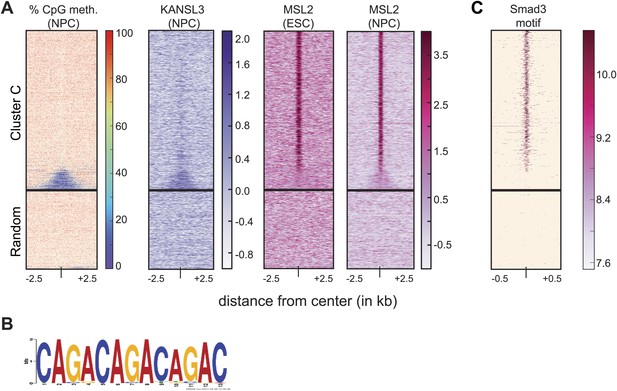
MSL2 has intergenic binding sites in DNA-hypomethylated regions that are enriched for SMAD3 binding sites.
(A) We extracted the percentage of methylated CpGs and the input-normalized ChIP-seq values from KANSL3 and MSL2 and 5 kb surrounding the center of the regions belonging to cluster C (Figure 2) and random genomic control regions. All heatmaps were sorted according to the percentages of methylated CpGs (Stadler et al., 2011). (B) Motif obtained by MEME analysis on the top 200 MSL2 peaks within cluster C. (C) Same as for (A), except that the score was the motif hit score for SMAD3 for 1 kb. See ‘Materials and methods’ for details.
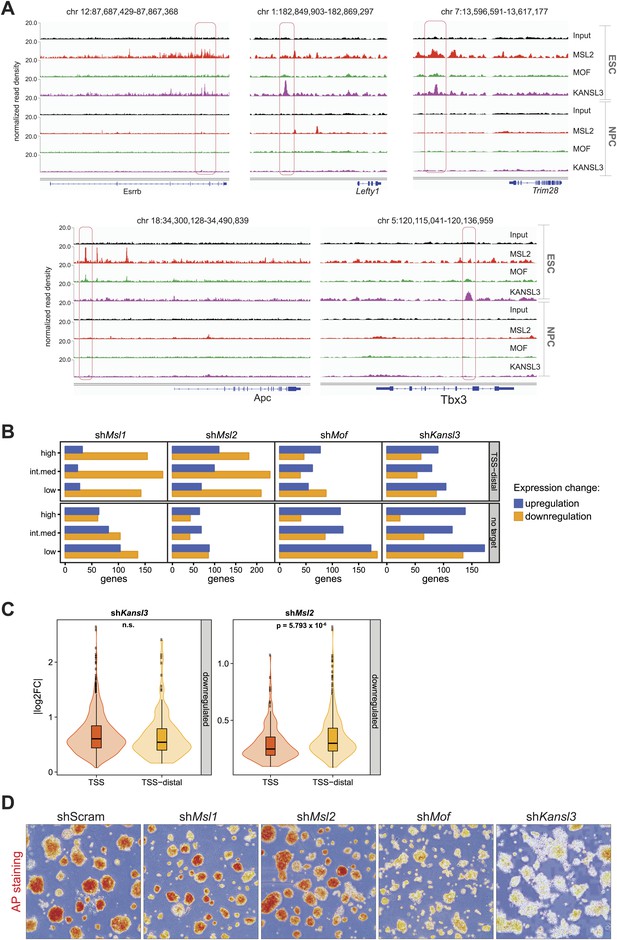
Biological significance of the TSS-distal binding sites of the investigated proteins.
(A) Genome browser snapshots of sequencing-depth normalized ChIP-seq and input profiles. Pink boxes mark the regions cloned and transfected into ESCs and NPCs for luciferase assays (Figure 4D). (B) Genes that were significantly up- or downregulated in the respective shRNA-treatments compared to shScrambled were classified according to ChIP-seq peak overlaps (TSS-distal, no target) and expression strength in wild-type ESCs (high, intermediate, low). See ‘Materials and methods’ for details of the classifications. (C) Distribution of absolute log2 fold changes (shKansl3 or shMsl2 compared to shScrambled) for significantly downregulated genes. Different shades of orange indicate different target classes based on ChIP-seq experiments for KANSL3 or MSL2, respectively. p-values were calculated with Welch t test. (D) Alkaline phosphatase staining and morphology of ESC colonies in indicated knockdowns after 4 days growth under puromycin selection (Figure 3—figure supplement 3A). MOF- and KANSL3-depleted cells demonstrate reduced alkaline phosphatase positive colonies with increased differentiation compared with MSL1- and MSL2-depleted cells and scrambled control.
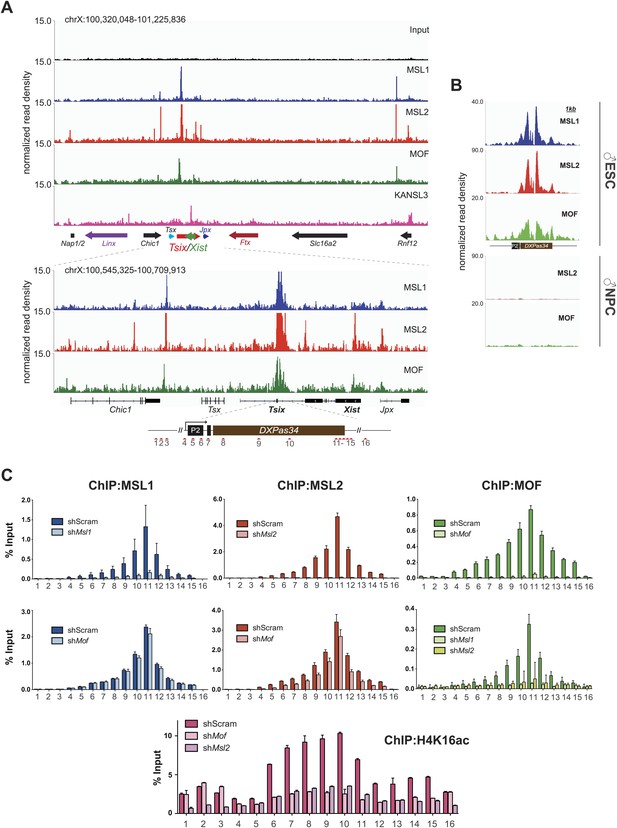
The MSL complex binds multiple loci within the X inactivation center including the Tsix DXPas34 minisatellite enhancer.
(A) Genome browser snapshots of the mouse X inactivation center (approximately 0.9 Mb) (upper panel) plus enlargement of the 164 kb region between Chic1 and Jpx/Enox (lower panel). The signals shown are the sequencing-depth normalized profiles for ChIP-seq from ESCs (for corresponding profiles in NPCs see Figure 5—figure supplement 1A); colored arrows indicate genes of lncRNAs. The schematic representation of the DXPas34 locus depicts the locations of the primer pairs that were used for ChIP-qPCR analyses (Supplementary file 3B). (B) Genome browser snapshots of the DXPas34 minisatellite of sequencing-depth normalized ChIP-seq profiles in ESCs and NPCs. (C) ChIP-qPCR analyses of MSL1 (blue), MSL2 (red), MOF (green), and H4K16 acetylation (purple) across the Tsix major promoter (P2) and the DXPas34 enhancer in male ESCs treated with the indicated shRNAs. For corresponding ChIP-qPCR in female ESCs see Figure 5—figure supplement 1C. Panels in the middle show the effects of MOF depletion on the recruitment of MSL1 and MSL2 to DXPas34 and vice versa. The bottom panel shows effects of depletion of control (dark pink), MOF (light pink) and MSL2 (purple) on the H4K16 acetylation signal. The labels of the x axes correspond to the arrowheads in (A). Results are expressed as mean ± SD of three biological replicates; cells were harvested on day 4 (Msl1, Msl2) or 5 (Mof) after shRNA treatment. For primer pairs see Supplementary file 3C.
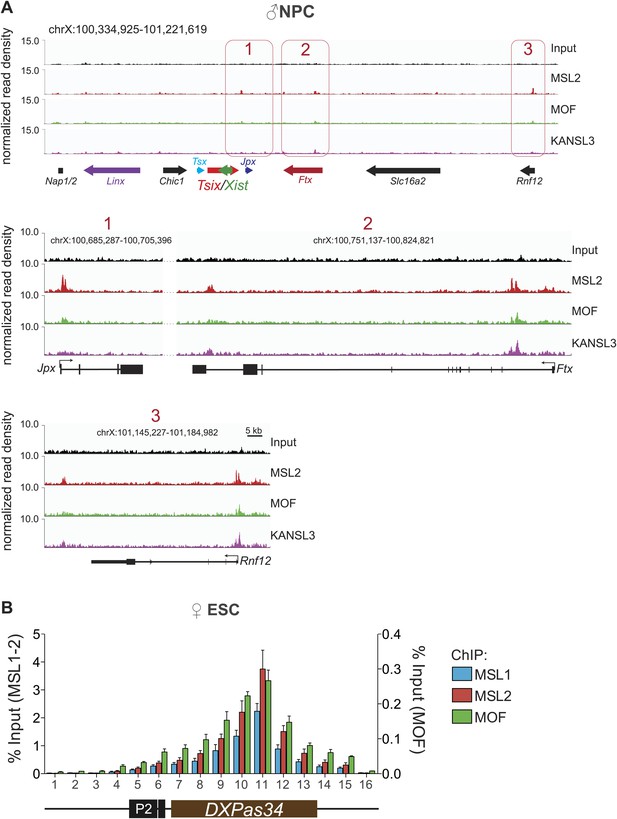
The MSL proteins bind to multiple loci within the X inactivation center (XIC).
(A) Genome browser snapshots of the mouse XIC (top panel) with three enlargements on Jpx, Ftx and Rnf12 genes (lower panels). Red boxes with corresponding numbers mark the enlarged regions presented in the lower panels. The exact genomic coordinates are indicated on top of each panel, arrows represent genes. The signals shown are the sequencing-depth normalized ChIP-seq profiles in NPCs. (B) ChIP analysis of MSL1, MSL2 and MOF across the DXPas34 minisatellite in female ESCs. The x-axis labels indicate the genomic coordinates corresponding to the arrowheads in Figure 5A. The y-axes show the percentage of ChIP recovery for MSL1 and MSL2 (left-hand side) and MOF (right-hand side) normalized to input. For all ChIP experiments, three biological replicates were used; all results are expressed as mean ± SD.
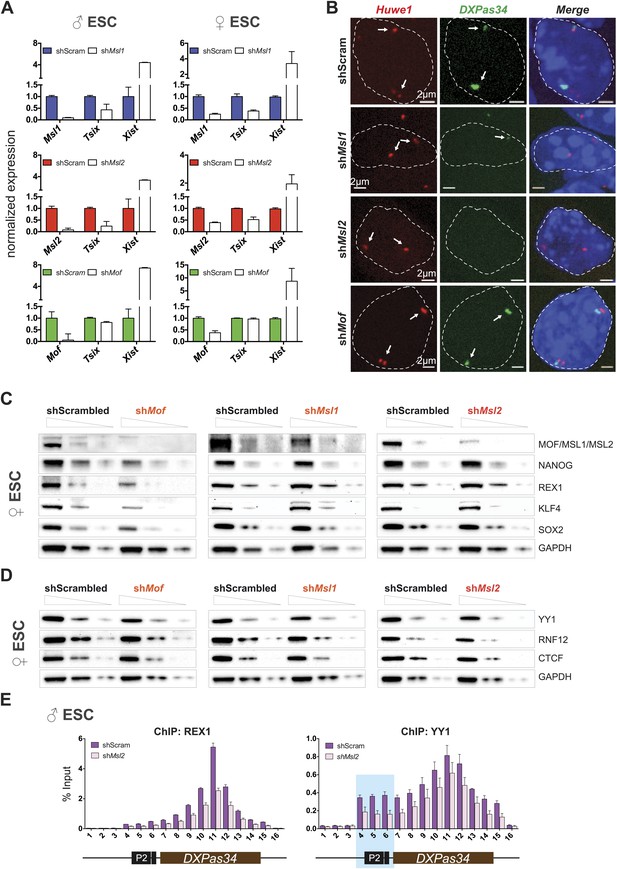
Depletion of MSL1 and MSL2 leads to downregulation of Tsix with concomitant upregulation of Xist.
(A) Gene expression analysis for the indicated genes in male and female ESCs treated with scrambled RNA (shScram) or shRNA against Msl1, Msl2, or Mof. All results are represented as relative values normalized to expression levels in shScram (normalized to Hprt) and expressed as means ± SD in three biological replicates. (B) RNA-FISH for Huwe1 (red) and DXPas34 (green) in: scrambled control, shMsl1-, shMsl2-, and shMof-treated female ESCs. Nuclei were counterstained with DAPI (blue). White arrows denote foci corresponding to Huwe1 or Tsix; dashed lines indicate nuclei borders. For additional images, phenotypes and quantifications see Figure 6—figure supplement 1A–C. For probe references see ‘Materials and methods’. (C) Western blot analyses of the pluripotency factors in scrambled-, Mof-, Msl1-, and Msl2-shRNA-treated female ESCs. For corresponding expression analyses see Figure 6—figure supplement 1D,E. The respective dilution (100%, 30%, 10%) of loaded RIPA extracts is shown above each panel. GAPDH was used as the loading control. For antibodies see ‘Materials and methods’. (D) Western blot analyses of the transcription factors involved in regulation of the XIC in scrambled-, Mof-, Msl1-, and Msl2-shRNA-treated female ESCs. The respective dilution (100%, 30%, 10%) of loaded RIPA extracts is shown above each panel. GAPDH was used as the loading control. (E) ChIP-qPCR analysis of REX1 (left panel) and YY1 (right panel) across the Tsix major promoter (P2) and DXPas34 in male ESCs treated with the indicated shRNAs. The labels of the x axes correspond to the arrowheads in Figure 5A. For all ChIP experiments, three biological replicates were used; results are expressed as mean ± SD; cells were harvested on day 4 (Msl2) or 5 (Mof) after shRNA treatment.
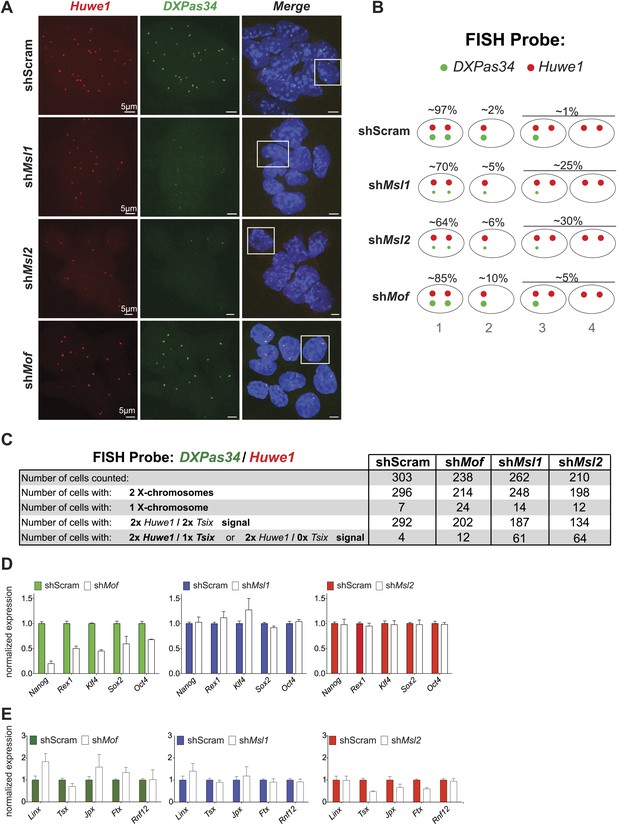
Cells depleted of MSL1 or MSL2, but not MOF show loss of DXPas34 foci.
(A) RNA-FISH for Huwe1 (red) and DXPas34 (green) in shScrambled-, shMsl1-, shMsl2-, and shMof-treated female ESCs. Shown here are examples of RNA-FISH signals for multicellular colonies and loss of DXPas34 signal in MSL1- and MSL2-depleted cells. White boxes indicate cells enlarged and resented in Figure 6B. For all experiments, nuclei were counterstained with DAPI (blue). (B) Summary of RNA-FISH for DXPas34 and Huwe1. Red dots indicate the number of X chromosomes and green dots, DXPas34 foci (smaller dot = reduced signal). Phenotypes that we observed in knockdowns are categorized into four groups containing cells with equal Huwe1/DXPas34 ratio and with DXPas34 loss. The percentages indicate how many cells per population showed the respective phenotype. (C) Corresponding to Figure 6B. Summary of total cell counts from RNA-FISH for (DXPas34) and Huwe1 in MSL1-, MSL2-, or MOF-depleted female ESCs. (D) Gene expression analysis for the indicated genes in female ESCs treated with scrambled RNA (shScram) or shRNA against Mof, Msl1 and Msl2. All results are represented as relative values normalized to expression levels in shScram (normalized to Hprt) and expressed as means ± SD in three biological replicates. (E) Gene expression analysis for genes of the XIC in female ESCs treated with scrambled RNA or shRNA against Msl1, Msl2 or Mof. All results are represented as relative values normalized to expression levels in shScrambled (normalized to Hprt) and expressed as means ± SD for three biological replicates.
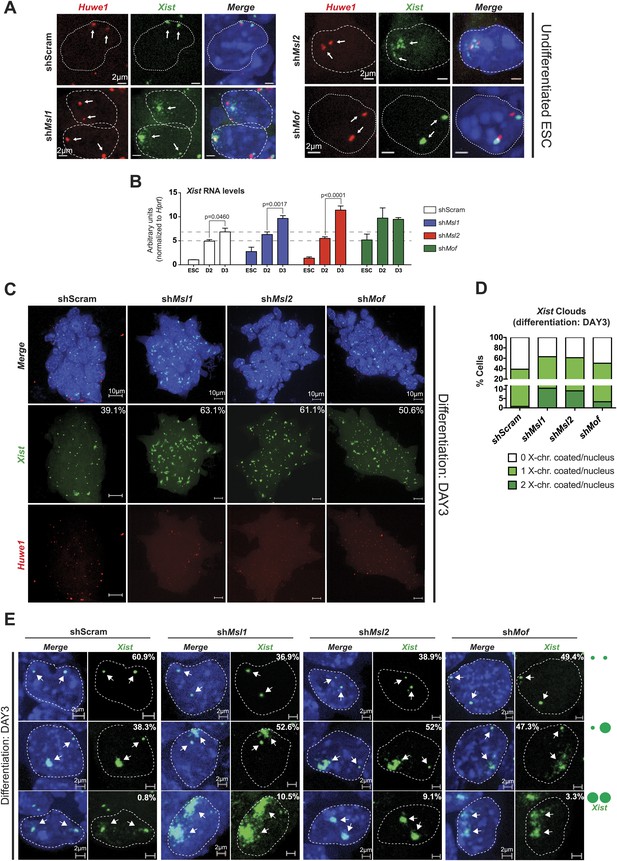
MSL1 and MSL2 depletion leads to enhanced and chaotic Xist accumulation in early differentiation.
(A) RNA-FISH for Huwe1 (red) and Xist (green) in: scrambled control, shMsl1-, shMsl2-, and shMof-treated female ESCs. Nuclei were counterstained with DAPI (blue). White arrows denote foci corresponding to Huwe1 or Xist; dashed lines indicate nuclei borders. For additional images, phenotypes and quantifications see Figure 7—figure supplement 1B–D. For probe references see ‘Materials and methods’. (B) Expression analysis for Xist in undifferentiated, day 2 (D2) and day 3 (D3) differentiating female ESCs treated with scrambled RNA (shScram) or shRNA against Mof, Msl1, and Msl2. All results are represented as arbitrary units (Xist expression in undifferentiated ESCs = 1) normalized to expression levels in shScram (normalized to Hprt) and expressed as means ± SD in three biological replicates. p-values for D2-to-D3 expression change were obtained using unpaired t test. (C) RNA-FISH for Huwe1 (red) and Xist (green) in: scrambled control, shMsl1-, shMsl2-, and shMof-treated differentiating female ESCs. Nuclei were counterstained with DAPI (blue). RNA-FISH was performed on the sixth day of knockdown (after 72 hr of differentiation). Percentages indicate number of cells with at least one Xist cloud for each of the knockdowns. For additional images of multicellular colonies see Figure 7—figure supplement 2A. (D) Bar plot summarizing the percentage of Xist clouds for individual knockdowns in differentiating (DAY3) female ESCs for individual knockdowns. Cells were divided into three categories: cells carrying no Xist clouds (white), single Xist cloud (light green), or two Xist clouds (dark green). For quantifications, see Figure 7—figure supplement 2B. (E) RNA-FISH for Xist (green) in: scrambled control, shMsl1-, shMsl2-, and shMof-treated differentiating (DAY3) female ESCs. Here, we show examples of individual nuclei carrying different patterns of Xist accumulation. Percentages correspond to the frequency of the shown Xist pattern within the population of cells. White arrows denote Xist foci; dashed lines indicate nuclei borders. For quantifications see Figure 7—figure supplement 2B.
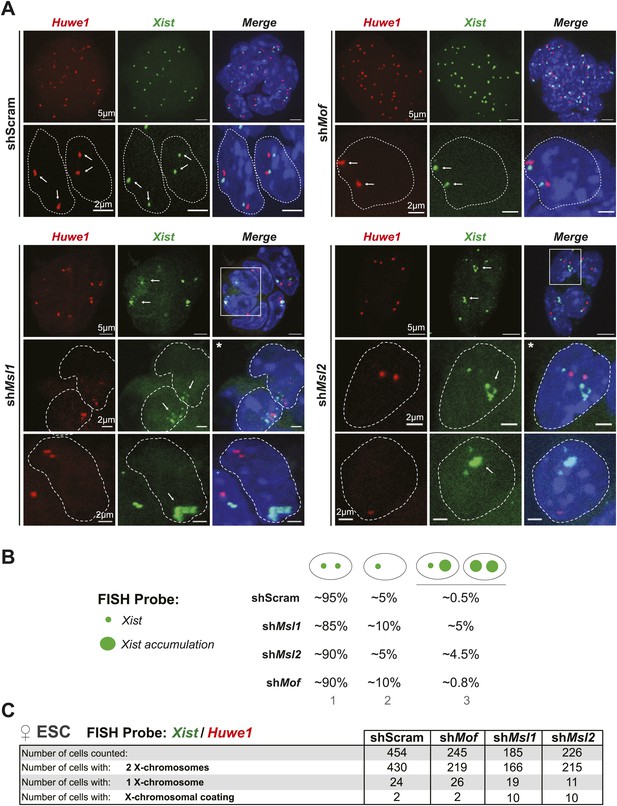
Depletion of MSL1 and MSL2 leads to occasional accumulation and spreading of Xist in undifferentiated ESCs.
(A) RNA-FISH for Huwe1 (red) and Xist (green) in shScrambled- (top left) and shMof- (top right), shMsl1- (bottom left) and shMsl2-treated (bottom right) female ESCs. Shown here are additional examples of RNA-FISH for multicellular colonies and individual cells exhibiting Xist-mediated coating (Figure 7A). White boxes indicate cells enlarged in Figure 7A. White arrows denote Huwe1 and Xist foci. Dashed lines indicate nuclei borders. For all experiments, nuclei were counterstained with DAPI (blue). (B) Summary of RNA-FISH for Xist and Huwe1. The number of green dots indicates the number of X chromosomes within the cell while the larger dot indicates Xist accumulation. Cells were classified into three phenotypic groups with cells showing sharp, localized Xist signals (once or twice) or Xist ‘clouds’. The percentages indicate how many cells per population showed the respective phenotype. (C) Corresponding to Figure 7A. Summary of the total cell counts from Xist and Huwe1 RNA-FISH in indicated knockdowns.
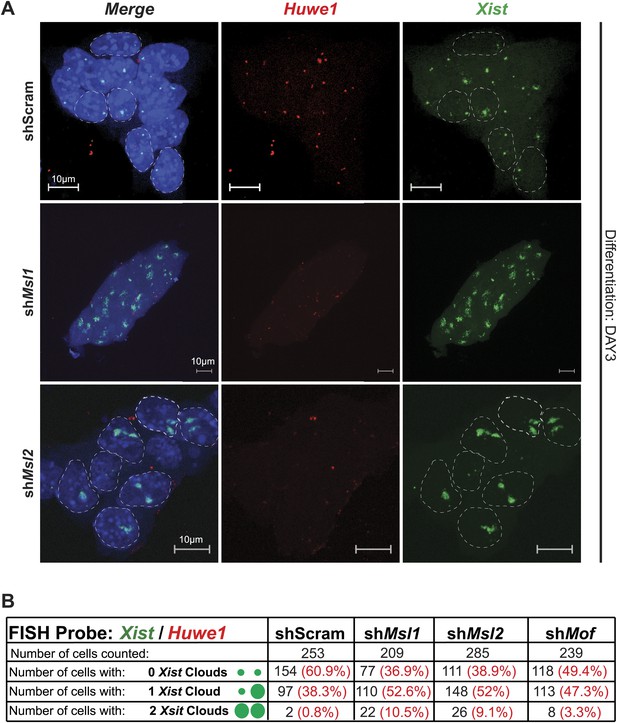
Depletion of MSL1 and MSL2 lead to enhanced Xist accumulation in differentiating ESCs.
(A) RNA-FISH for Huwe1 (red) and Xist (green) in shScrambled-, shMsl1-, and shMsl2-treated differentiating (DAY3) female ESCs. Shown here are additional examples of RNA-FISH for multicellular colonies (Figure 7C). Dashed lines indicate nuclei borders. For all experiments, nuclei were counterstained with DAPI (blue). (B) Corresponding to Figure 7C–E. Summary of the total cell counts from Xist RNA-FISH in indicated knockdowns. Percentage of cells with respective phenotype indicated in red.
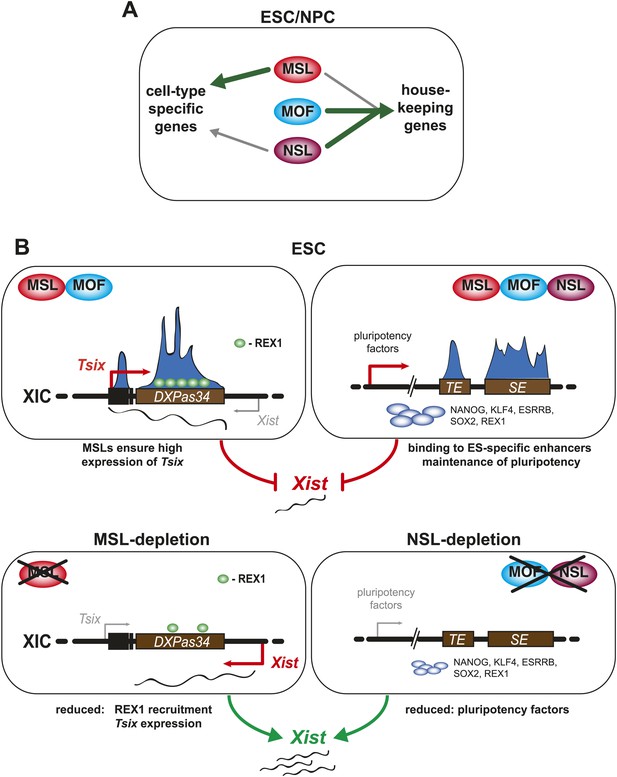
A summary model. Shared and distinct pathways by which MOF, MSLs and NSLs regulate gene expression, pluripotency, and the X inactivation center.
(A) In this study, we have identified several modes of concurrent and independent binding of mammalian MOF, MSL and NSL proteins. We find that all complexes bind to promoters of housekeeping genes in ESCs and NPCs with NSL complex members occupying the majority of the target genes, while MOF and MSL proteins bind NSL-bound genes in a more restricted manner. Furthermore, we observe that upon differentiation, KANSL3 and MSL2 additionally occupy TSSs of different sets of cell-type-specific genes in the absence of MOF. (B) When we studied the functions of MSL and NSL complexes at the murine X inactivation center, we determined two basic mechanisms by which the different proteins affect the maintenance of two active X chromosomes in ESCs. (1) MSLs bind to the promoter and enhancer of Tsix whose transcription represses Xist expression. Upon depletion of MSLs, Tsix expression is compromised, so is REX1 recruitment to the Tsix locus. Consequently, Xist is increasingly transcribed and can occasionally accumulate. (2) In addition, MOF, MSLs, and NSLs bind to typical enhancers (TE) and super enhancers (SE) in ESCs, and notably those of pluripotency factors. In WT ESCs, the high expression of pluripotency factors is another layer of Xist repression. The depletions of MOF or KANSL3, but not of MSL1 or MSL2 reduce the expression of pluripotency factors involved in Xist repression causing a Tsix-independent increase of Xist expression.
Additional files
-
Supplementary file 1
MOF/MSL/NSL ChIP-seq statistics.
- https://doi.org/10.7554/eLife.02024.026
-
Supplementary file 2
Detailed information about publicly available genome-wide resources used in this study.
- https://doi.org/10.7554/eLife.02024.027
-
Supplementary file 3
Lists of primer pairs used in ChIP-qPCR and RT-PCR analyses.
- https://doi.org/10.7554/eLife.02024.028