Prion propagation can occur in a prokaryote and requires the ClpB chaperone
Figures
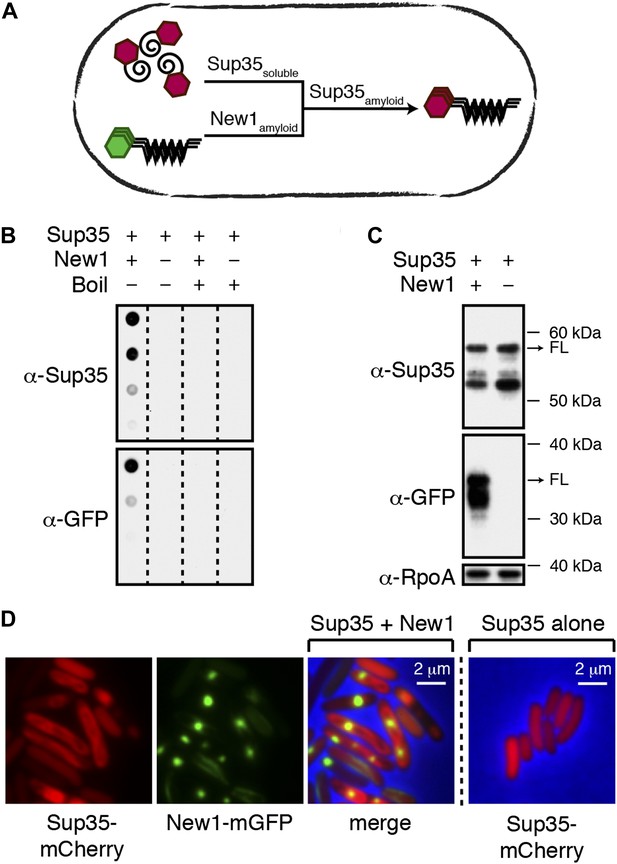
Conversion of Sup35 NM to its prion form in E. coli requires New1.
(A) Cartoon representation of how conversion of soluble Sup35 NM (Sup35soluble) to its amyloid conformation (Sup35amyloid) depends on the presence of New1 in its amyloid conformation (New1amyloid). Sup35 NM and New1 (black) are depicted as fusions to mCherry (red) and mGFP (green), respectively. (B) SDS-stable Sup35 NM aggregates are detected only in cells producing SDS-stable New1 aggregates as assessed by filter retention analysis. For each sample, undiluted lysate and three twofold dilutions are shown (see ‘Materials and methods’). Sup35 NM and New1 aggregates are no longer detected once boiled. The α-Sup35 antibody recognizes the Sup35 NM-mCherry-His6X fusion protein, and the α-GFP antibody detects the New1-mGFP fusion protein. (C) Intracellular full-length (FL) Sup35 NM fusion protein levels are comparable in the presence and absence of New1 as assessed by Western blot analysis. The α-RpoA antibody recognizes the α subunit of E. coli RNA polymerase. (D) Fluorescence images of representative cells containing Sup35 NM and New1 or Sup35 NM alone. For cells containing both fusion proteins, the mCherry channel, GFP channel, and merged images are shown.
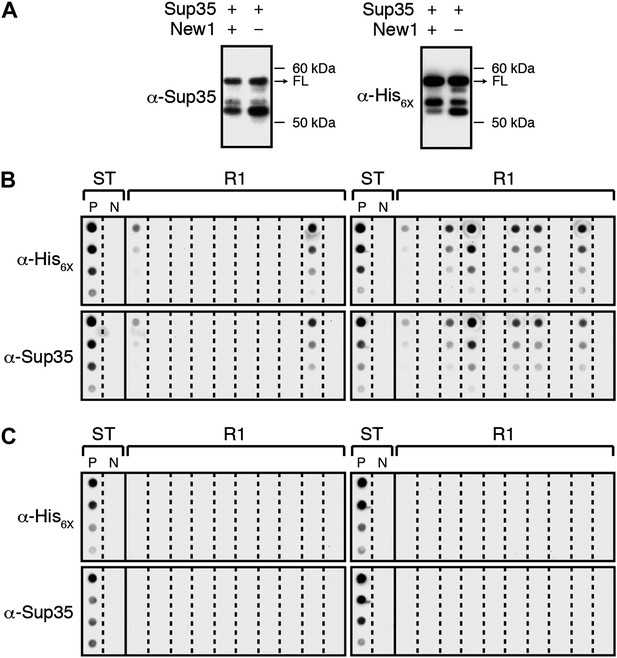
α-Sup35 and α-His6X antibodies are interchangeable for detecting the Sup35 NM-mCherry-His6X fusion protein.
(A) Probing nitrocellulose membranes with either α-Sup35 antibody (Figure 1C) or α-His6X antibody results in the detection of similar intracellular Sup35 NM fusion protein products in the presence or absence of New1 as assessed by Western blot analysis. Full-length (FL) Sup35 NM fusion proteins are indicated by arrows. (B) Probing cellulose acetate membranes with either α-His6X antibody (Figure 3B) or α-Sup35 antibody results in the detection of similar aggregate-positive and aggregate-negative samples in starter cultures (ST) and Round 1 (R1) experimental clones as assessed by filter retention analysis. For each sample, undiluted lysate and three twofold dilutions are shown. Starter cultures of cells containing Sup35 NM and New1 and cells containing Sup35 NM alone serve as positive (P) and negative (N) controls, respectively. (C) Probing cellulose acetate membranes with either α-His6X antibody (Figure 3D) or α-Sup35 antibody results in the detection of similar aggregate-positive and aggregate-negative samples in starter cultures (ST) and R1 control clones as assessed by filter retention analysis.
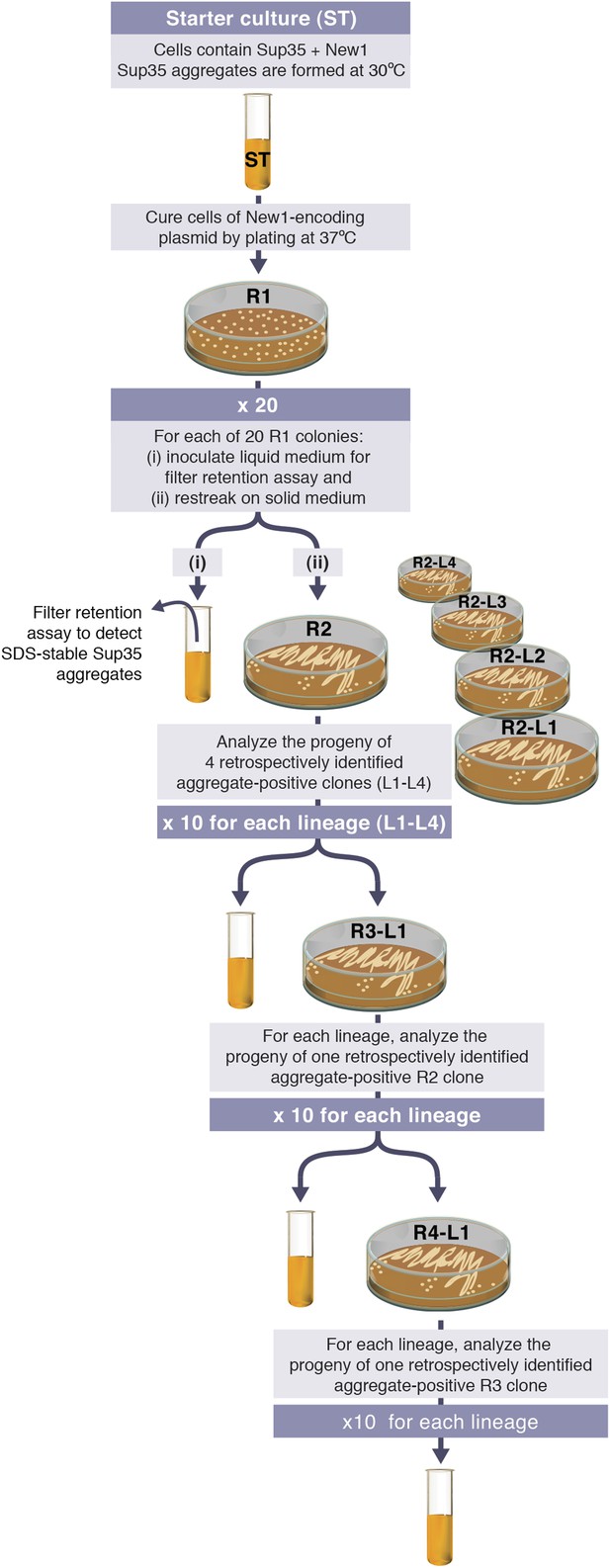
Experimental protocol for assessing the ability of E. coli cells to propagate SDS-stable Sup35 NM aggregates.
Experiments are initiated with either a starter culture (ST) of cells containing Sup35 NM and New1 (shown) or a starter culture of cells containing Sup35 NM alone (not shown). For each of the 4 lineages (L1–L4), the total number of generations over which Sup35 NM prion propagation is monitored corresponds to the number of cell divisions that occur in the absence of New1 during 4 rounds (R1–R4) of growth on solid medium and an additional round of growth in liquid medium. Growth in the absence of New1 begins at the time the starter culture cells are plated at 37°C (R1). Single R1 colonies were found to contain ∼950,000 colony forming units (CFUs), and the liquid cultures contain ∼108 CFUs per μl. Thus, prion propagation is monitored over 98.7 or ∼100 generations. We note that the presence of SDS-stable Sup35 NM aggregates in experimental starter culture cells does not represent prion propagation because the presence of New1 (i.e., [PIN+]) enables the continuing de novo conversion of newly synthesized Sup35 NM to the prion form.
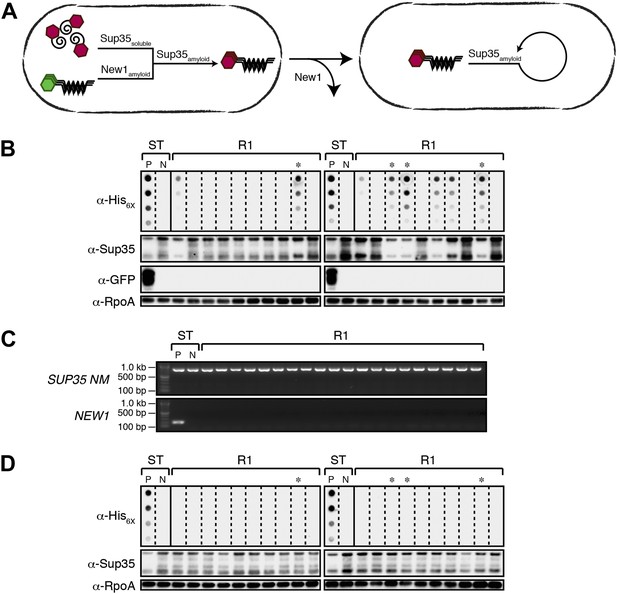
Converted Sup35 NM can remain in its prion conformation in E. coli cells lacking New1.
(A) Cartoon representation of how Sup35 NM can convert to its prion form in the presence of New1 and remain in the prion conformation after cells have been cured of New1-encoding DNA. Sup35 NM and New1 (black) are depicted as fusions to mCherry (red) and mGFP (green), respectively. (B) SDS-stable Sup35 NM aggregates are detected in 8 of 20 Round 1 (R1) experimental clones derived from a starter culture (ST) of cells containing Sup35 NM and New1 as assessed by filter retention analysis. Starter cultures of cells containing Sup35 NM and New1 and cells containing Sup35 NM alone serve as positive (P) and negative (N) controls, respectively. The four aggregate-positive clones selected to establish the four experimental lineages are indicated by asterisks. In all 20 R1 experimental samples, intracellular Sup35 NM fusion protein levels are comparable, and New1 fusion protein is not detectable as assessed by Western blot analysis. The α-His6X and α-Sup35 antibodies recognize the Sup35 NM-mCherry-His6X fusion protein (see Figure 1—figure supplement 1B,C), the α-GFP antibody detects the New1-mGFP fusion protein, and the α-RpoA antibody recognizes the α subunit of E. coli RNA polymerase. (C) In all 20 R1 experimental samples, DNA encoding Sup35 NM is detectable whereas DNA encoding the prionogenic module of New1 is not detectable by PCR. (D) SDS-stable Sup35 NM aggregates are not detected in any of the 20 R1 control clones derived from a starter culture of cells containing Sup35 NM alone as assessed by filter retention analysis. The four aggregate-negative clones selected to establish the four control lineages are indicated by asterisks. Intracellular Sup35 NM fusion protein levels are comparable in all 20 R1 control samples.
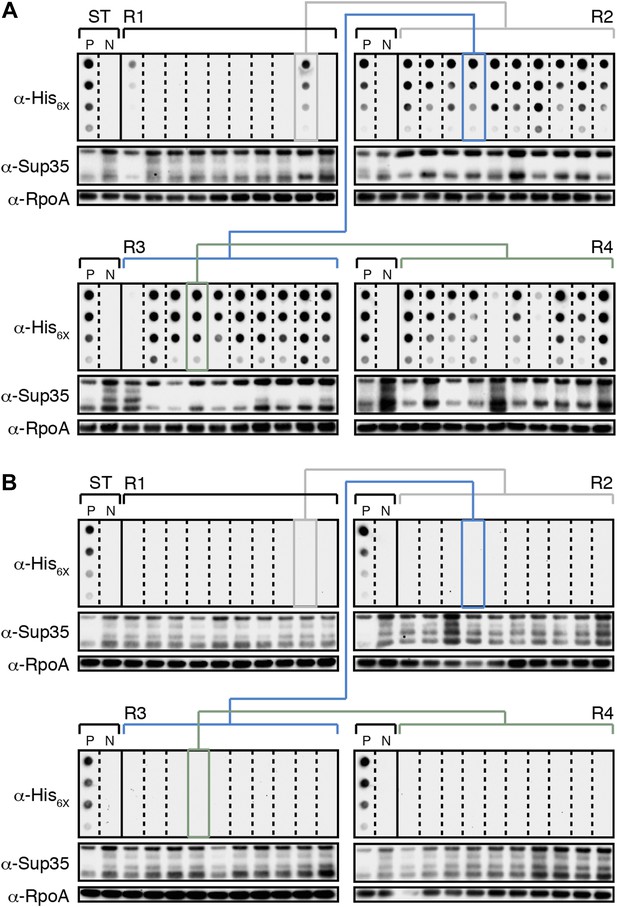
E. coli can propagate the Sup35 NM prion over ∼100 generations.
(A) Experimental Lineage 1 (L1E). An aggregate-positive Round 1 (R1) experimental clone (gray box) derived from a starter culture (ST) of cells containing Sup35 NM and New1 is identified and restreaked to yield progeny Round 2 (R2) clones (gray bracket). All 10 R2 clones analyzed contain detectable SDS-stable Sup35 NM aggregates. An aggregate-positive R2 clone (blue box) is identified and restreaked to yield progeny Round 3 (R3) clones (blue bracket). Again, all 10 R3 clones analyzed contain SDS-stable Sup35 NM aggregates. An aggregate-positive R3 clone (green box) is identified and restreaked to yield progeny Round 4 (R4) clones (green bracket). 9 of 10 R4 clones analyzed contain SDS-stable Sup35 NM aggregates. The filter retention assay is used to detect SDS-stable Sup35 NM aggregates. Intracellular Sup35 NM fusion protein levels are comparable in all 40 samples as assessed by Western blot analysis. Starter cultures of cells containing Sup35 NM and New1 and cells containing Sup35 NM alone serve as positive (P) and negative (N) controls, respectively. The α-His6X and α-Sup35 antibodies recognize the Sup35 NM-mCherry-His6X fusion protein, and the α-RpoA antibody recognizes the α subunit of E. coli RNA polymerase. (B) Control Lineage 1 (L1C). An aggregate-negative R1 control clone (gray box) derived from a starter culture of cells containing Sup35 NM alone is identified and restreaked to yield progeny R2 clones (gray bracket), an aggregate-negative R2 clone (blue box) is identified and restreaked to yield progeny R3 clones (blue bracket), and an aggregate-negative R3 clone (green box) is identified and restreaked to yield progeny R4 clones (green bracket). No SDS-stable Sup35 NM aggregates are detectable in any sample. Intracellular Sup35 NM fusion protein levels are comparable in all 40 samples as assessed by Western blot analysis.
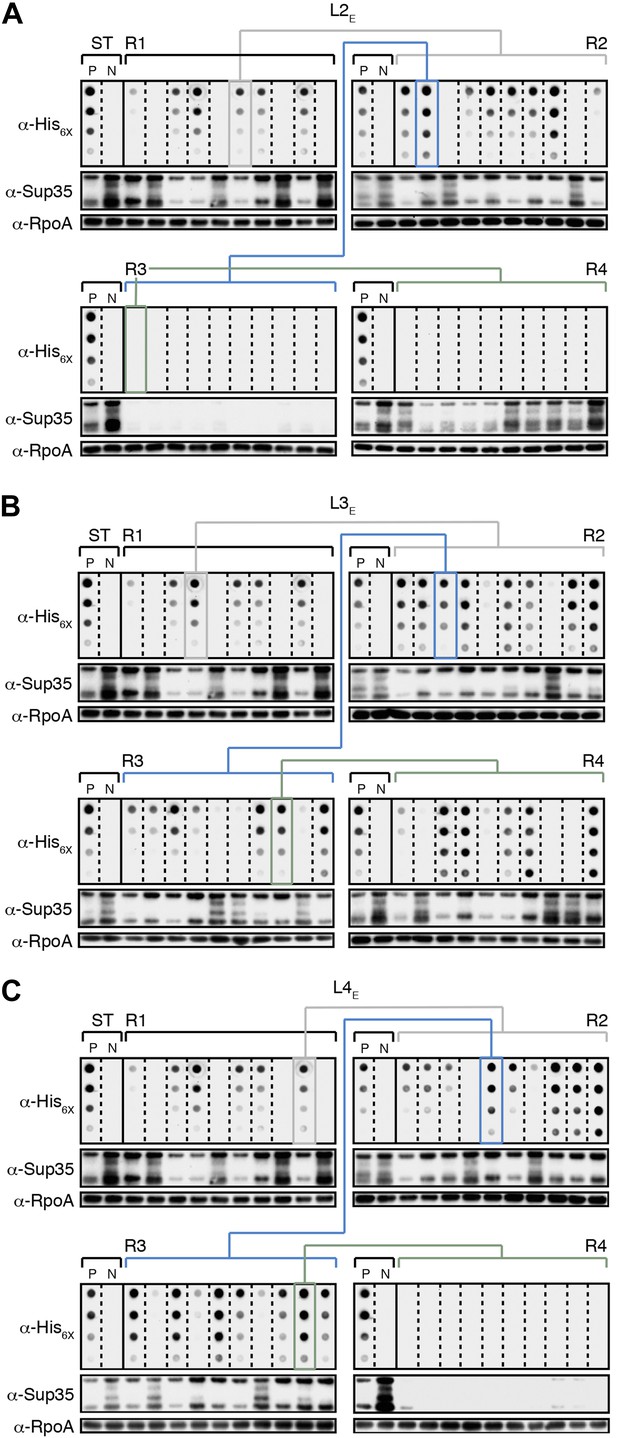
The fate of Sup35 NM in experimental Lineages 2-4.
(A) Experimental Lineage 2 (L2E). An aggregate-positive Round 1 (R1) clone (gray box) derived from a starter culture (ST) of cells containing Sup35 NM and New1 is identified and restreaked to yield progeny Round 2 (R2) clones (gray bracket). 8 of 10 R2 clones analyzed contain detectable SDS-stable Sup35 NM aggregates as assessed by filter retention analysis. An aggregate-positive R2 clone (blue box) is restreaked to yield progeny Round 3 (R3) clones (blue bracket). 0 of 10 R3 clones analyzed contain detectable SDS-stable Sup35 NM aggregates. The apparent loss of aggregates coincides with the loss of detectable Sup35 NM fusion protein as assessed by Western blot analysis. Assaying the 10 Round 4 (R4) progeny clones derived from an aggregate-negative R3 clone (green box) reveals that fusion protein levels can be restored without the recovery of SDS-stable Sup35 NM aggregates (green bracket). Starter cultures of cells containing Sup35 NM and New1 and cells containing Sup35 NM alone serve as positive (P) and negative (N) controls, respectively. The α-His6X and α-Sup35 antibodies recognize the Sup35 NM-mCherry-His6X fusion protein, and the α-RpoA antibody recognizes the α subunit of E. coli RNA polymerase. (B) Experimental Lineage 3 (L3E). 9 of 10 R2 clones analyzed contain detectable SDS-stable Sup35 NM aggregates, which are retained in 8 of 10 R3 progeny clones and 7 of 10 R4 progeny clones. (C) Experimental Lineage 4 (L4E). 8 of 10 R2 clones analyzed contain detectable SDS-stable Sup35 NM aggregates, which are retained in 9 of 10 R3 progeny clones. 0 of 10 R4 clones analyzed contain detectable SDS-stable Sup35 NM aggregates. As in R3 of L2E (A), the apparent loss of aggregates coincides with a dramatic drop in Sup35 NM fusion protein levels.
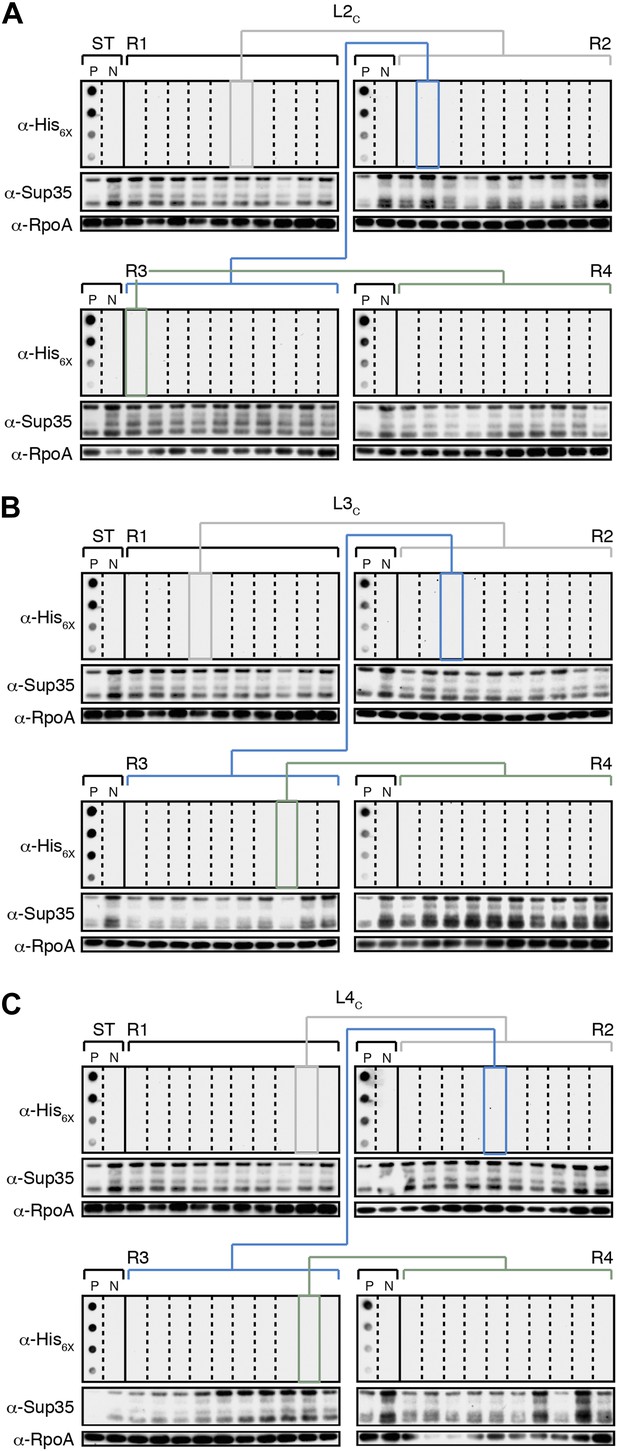
The fate of Sup35 NM in control Lineages 2–4.
(A) Control Lineage 2 (L2C). An aggregate-negative Round 1 (R1) clone (gray box) derived from a starter culture (ST) of cells containing Sup35 NM alone is identified and restreaked to yield progeny Round 2 (R2) clones (gray bracket). 0 of 10 R2 clones analyzed contain detectable SDS-stable Sup35 NM aggregates as assessed by filter retention analysis. An aggregate-negative R2 clone (blue box) is restreaked to yield progeny R3 clones (blue bracket). 0 of 10 R3 clones analyzed contain detectable SDS-stable Sup35 NM aggregates. An aggregate-negative R3 clone (green box) is restreaked to yield progeny R4 clones (green bracket). 0 of 10 R4 clones analyzed contain detectable SDS-stable Sup35 NM aggregates. Starter cultures of cells containing Sup35 NM and New1 and cells containing Sup35 NM alone serve as positive (P) and negative (N) controls, respectively. The α-His6X and α-Sup35 antibodies recognize the Sup35 NM-mCherry-His6X fusion protein, and the α-RpoA antibody recognizes the α subunit of E. coli RNA polymerase. (B) Control Lineage 3 (L3C). As in L2C (A), no SDS-stable Sup35 NM aggregates are detectable in progeny R2, R3, or R4 clones. (C) Control Lineage 4 (L4C). As in L2C (A) and L3C (B), no SDS-stable Sup35 NM aggregates are detectable in progeny R2, R3, or R4 clones.
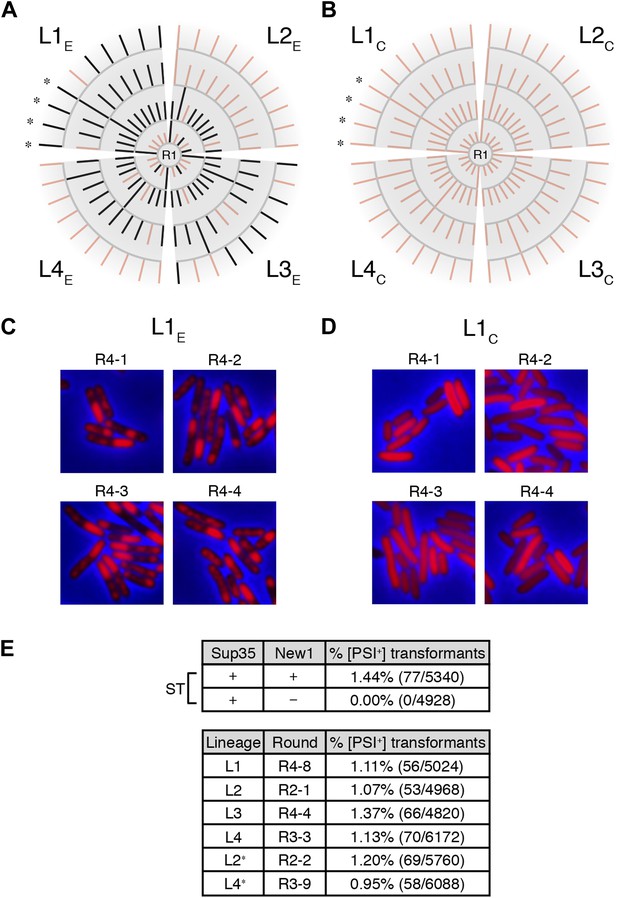
Genealogy of E. coli cell lineages propagating Sup35 NM in an infectious prion conformation.
(A) The fate of Sup35 NM in four experimental lineages (L1E-L4E) established from a starter culture of cells containing Sup35 NM and New1 is shown. Clones that maintain or lose the Sup35 NM prion are indicated by black or pink lines, respectively. Rounds 1–4 (R1–R4) are depicted as gray arcs, with R1 situated at the center of the tree. Clones are designated as aggregate-positive if they contain SDS-stable Sup35 NM aggregates that are detectable in the undiluted sample and at least 1 of the 3 two-fold serial dilutions, as analyzed by filter retention. L1E and L3E retain SDS-stable Sup35 NM aggregates for the duration of the experiment (Figure 4A, Figure 4—figure supplement 1B). L2E and L4E lose detectable SDS-stable Sup35 NM aggregates at R3 and R4, respectively. In both cases, the loss of SDS-stable aggregates coincides with a dramatic yet apparently reversible drop in fusion protein levels (Figure 4—figure supplement 1A,C; see ‘Discussion’). Cells from four aggregate-positive L1E-R4 clones visualized by fluorescence microscopy are indicated by asterisks. (B) The fate of Sup35 NM in four control lineages (L1C–L4C) established from a starter culture of cells containing Sup35 NM alone is shown. None of the 120 clones analyzed contain SDS-stable Sup35 NM aggregates (Figure 4B, Figure 4—figure supplement 2). Cells from four aggregate-negative L1C-R4 clones visualized by fluorescence microscopy are indicated by asterisks. (C) Fluorescence images of representative cells corresponding to the four aggregate-positive R4 clones indicated by asterisks in (A). (D) Fluorescence images of representative cells corresponding to the four aggregate-negative R4 clones indicated by asterisks in (B). (E) E. coli cell extracts containing propagated, SDS-stable Sup35 NM aggregates are infectious when transformed into S. cerevisiae [psi−] cells. A starter culture (ST) of cells containing Sup35 NM and New1 contain infectious SDS-stable Sup35 NM aggregates capable of converting [psi−] yeast cells to [PSI+]. In contrast, a starter culture of cells containing Sup35 NM alone lacks detectable infectivity. Progeny cell extracts transformed into yeast are identified as RX-Y, where X corresponds to a round number and Y corresponds to a clone number assigned sequentially and clockwise according to (A) and (B). Clones that gave rise to aggregate-negative progeny in the subsequent round are indicated by asterisks. Analysis of these data by Fisher's exact test indicates that the differences in the frequency of [PSI+] transformants observed with samples containing SDS-stable Sup35 NM aggregates compared with the sample containing soluble Sup35 NM are statistically significant (p < 0.0001). The percentages given refer to strong [PSI+] transformants; samples containing SDS-stable Sup35 NM aggregates (but not samples containing soluble Sup35 NM) also gave rise to weak [PSI+] transformants (Figure 5—figure supplement 1), but these were not quantified (‘Results’).
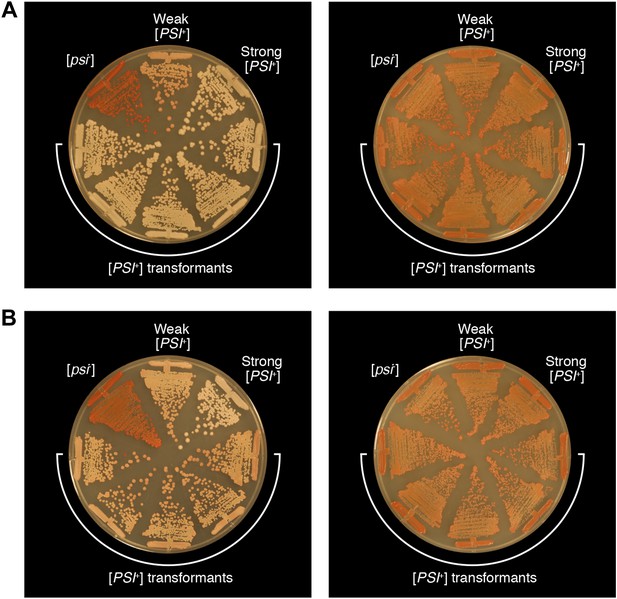
Bacterial cell extracts containing propagated, infectious Sup35 NM aggregates yield both strong and weak [PSI+] yeast transformants.
The phenotypes of five representative strong [PSI+] (A) and weak [PSI+] (B) strains obtained by transforming S. cerevisiae [psi−] cells with E. coli cell extracts containing propagated, SDS-stable Sup35 NM-aggregates on 1/4 YPD agar before (left) and after (right) passage on YPD agar supplemented with 3 mM GuHCl. For the purposes of comparison, untransformed [psi−], weak [PSI+], and strong [PSI+] yeast strains are shown.
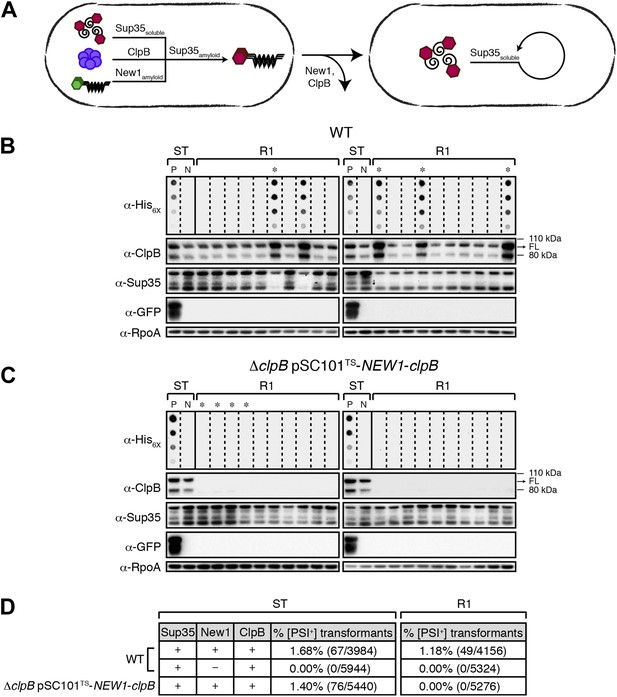
Sup35 NM prion propagation in E. coli requires ClpB.
(A) Cartoon representation of how Sup35 NM can convert to its prion form in the presence of New1 and ClpB but cannot propagate in the prion conformation after cells have been cured of New1- and ClpB-encoding DNA. Sup35 NM and New1 (black) are depicted as fusions to mCherry (red) and mGFP (green), respectively. ClpB is depicted as a purple hexamer. (B) SDS-stable Sup35 NM aggregates are detected in 5 of 20 Round 1 (R1) wild-type (WT) clones derived from a starter culture (ST) of wild-type cells containing Sup35 NM and New1 as assessed by filter retention analysis. In total, 17 of 60 R1 wild-type clones are aggregate-positive (Figure 6—figure supplement 2A). Starter cultures of cells containing Sup35 NM and New1 and cells containing Sup35 NM alone serve as positive (P) and negative (N) controls, respectively. In all 20 R1 wild-type clones shown, full-length (FL) ClpB is detectable, Sup35 NM fusion protein levels are comparable, and New1 fusion protein is not detectable as assessed by Western blot analysis. The α-His6X and α-Sup35 antibodies recognize the Sup35 NM-mCherry-His6X fusion protein, the α-GFP antibody recognizes the New1–mGFP fusion protein, the α-ClpB antibody recognizes the E. coli ClpB chaperone, and the α-RpoA antibody recognizes the α subunit of E. coli RNA polymerase. Cells from four aggregate-positive R1 wild-type clones visualized by fluorescence microscopy (Figure 6—figure supplement 2C) are indicated by asterisks. (C) SDS-stable Sup35 NM aggregates are not detectable in R1 ΔclpB clones derived from a starter culture of ΔclpB cells containing Sup35 NM, New1, and ectopically produced ClpB as assessed by filter retention analysis. In total, 0 of 60 R1 ΔclpB clones are aggregate-positive (Figure 6—figure supplement 2B). Starter cultures of wild-type cells containing Sup35 NM and New1 and wild-type cells containing Sup35 NM alone serve as positive (P) and negative (N) controls, respectively. In all 20 R1 ΔclpB clones shown, Sup35 NM fusion protein levels are comparable, and neither ClpB nor New1 fusion protein is detectable as assessed by Western blot analysis. Cells from four aggregate-negative R1 wild-type clones visualized by fluorescence microscopy (Figure 6—figure supplement 2D) are indicated by asterisks. (D) Extract prepared from cells lacking ClpB is not infectious when transformed into S. cerevisiae [psi–] cells. Starter cultures of wild-type cells transformed with pBR322-SUP35 NM and pSC101TS-NEW1 as well as ΔclpB cells transformed with pBR322-SUP35 NM and pSC101TS-NEW1-clpB contain infectious SDS-stable Sup35 NM aggregates capable of converting [psi−] yeast cells to [PSI+]. Wild-type starter culture cells containing Sup35 NM alone lack detectable infectivity. An aggregate-positive R1 wild-type clone retains infectious Sup35 NM aggregates. In contrast, an aggregate-negative R1 ΔclpB clone lacks detectable infectivity, as does an aggregate-negative R1 wild-type clone. Analysis of these data by Fisher's exact test indicates that the differences in the frequency of [PSI+] transformants observed with samples containing SDS-stable Sup35 NM aggregates compared with the samples containing soluble Sup35 NM are statistically significant (p < 0.0001).
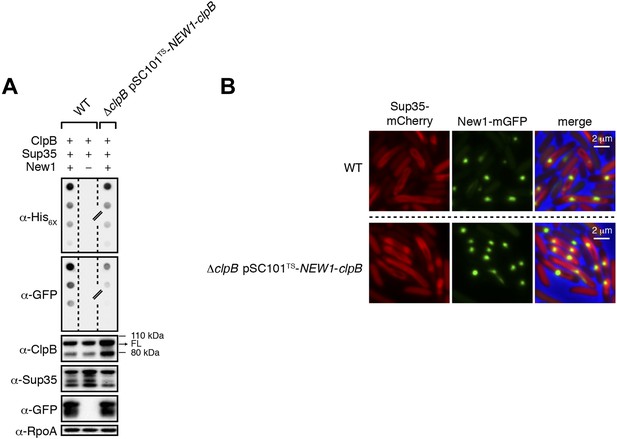
ΔclpB cells containing New1 and ectopically produced ClpB support the formation of SDS-stable Sup35 NM aggregates.
(A) SDS-stable Sup35 NM aggregates are detected in wild-type (WT) cells producing SDS-stable New1 aggregates as assessed by filter retention analysis. SDS-stable Sup35 NM aggregates are also detected in ΔclpB cells containing SDS-stable New1 aggregates and ectopically produced ClpB. The α-His6X antibody detects the Sup35 NM-mCherry-His6X fusion protein, and the α-GFP antibody detects the New1-mGFP fusion protein. A lane cropped from the same immunoblot is indicated by a hash mark. Intracellular levels of full-length (FL) ClpB, Sup35 NM fusion protein, and New1 fusion protein are comparable in the presence and absence of New1 and ectopically produced ClpB as assessed by Western blot analysis. The α-ClpB antibody recognizes the E. coli ClpB chaperone, the α-Sup35 antibody recognizes the Sup35 NM fusion protein, and the α-RpoA antibody recognizes the α subunit of E. coli RNA polymerase. (B) Fluorescence images of representative wild-type cells containing Sup35 NM and New1 and ΔclpB cells containing Sup35 NM, New1, and ectopically produced ClpB. The mCherry channel, GFP channel, and merged images are shown.
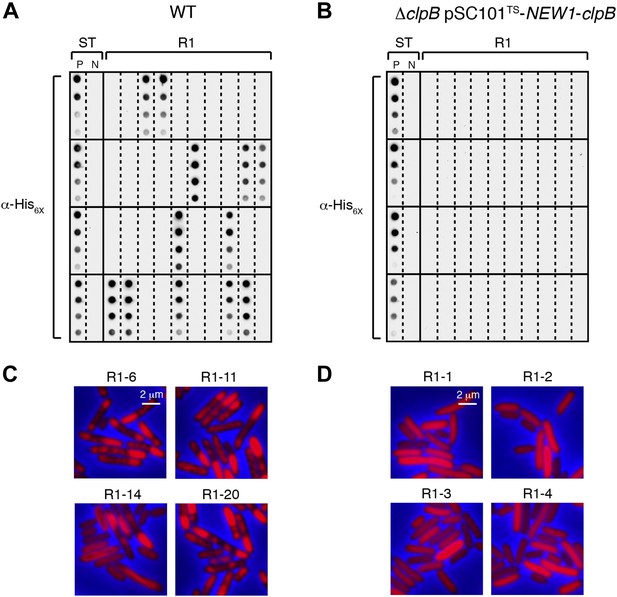
The fate of Sup35 NM in 40 wild-type R1 clones and 40 ΔclpB R1 clones.
(A) SDS-stable Sup35 NM aggregates are detected in 12 of 40 Round 1 (R1) wild-type (WT) clones derived from a starter culture (ST) of wild-type cells containing Sup35 NM and New1 as assessed by filter retention analysis. In total, 17 of 60 R1 wild-type clones are aggregate-positive (Figure 6B). Starter cultures of cells containing Sup35 NM and New1 and cells containing Sup35 NM alone serve as positive (P) and negative (N) controls, respectively. The α-His6X antibody detects the Sup35 NM-mCherry-His6X fusion protein. (B) In contrast, 0 of 40 R1 ΔclpB clones derived from a starter culture of ΔclpB cells transformed with pBR322-SUP35 NM and pSC101TS-NEW1-clpB contain detectable SDS-stable Sup35 NM aggregates. In total, 0 of 60 R1 ΔclpB clones are aggregate-positive (Figure 6C). The observed difference in the number of aggregate-positive clones of wild-type vs ΔclpB cells is statistically significant (p < 0.0001 as determined by Fisher's Exact Test). (C) Fluorescence images of representative cells corresponding to the four aggregate-positive R1 wild-type clones indicated by asterisks in Figure 6B. Notably, wild-type clone R1-14 exhibits twisted ring structures. (D) Fluorescence images of representative cells corresponding to the four aggregate-negative R1 ΔclpB clones indicated by asterisks in Figure 6C.
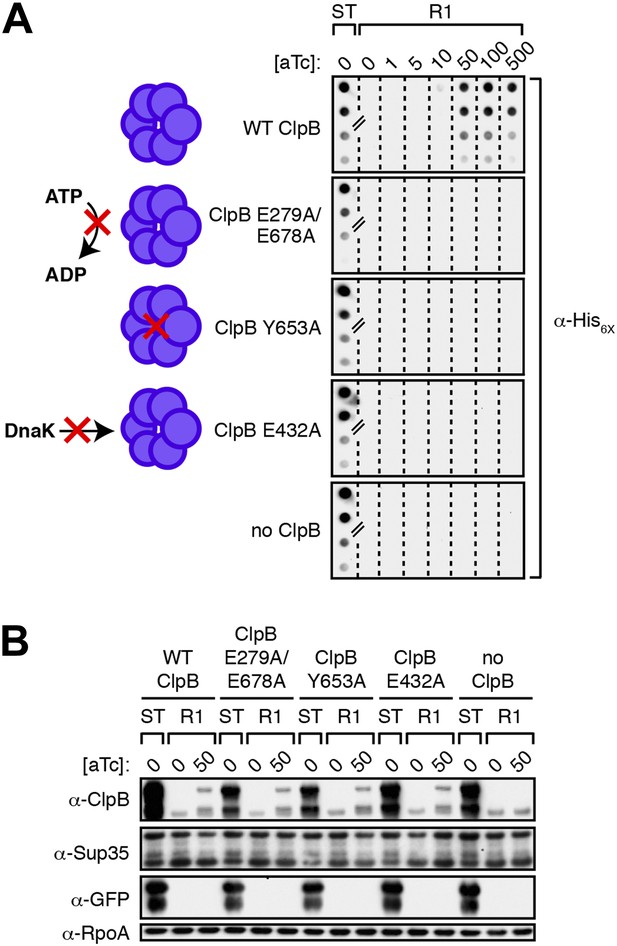
Propagation of SDS-stable Sup35 NM aggregates in E. coli requires ClpB disaggregase activity.
(A) SDS-stable Sup35 NM aggregates are detected in starter cultures (ST) of ΔclpB cells containing pBR322-SUP35 NM, pSC101TS-NEW1-clpB, and one of four aTc-inducible chromosomal clpB alleles. Wild-type (WT) ClpB is depicted as a purple hexamer. ClpB E279A/E678A is unable to hydrolyze ATP, ClpB Y653A is pore-deficient, and ClpB E432A is unable to collaborate with DnaK. Propagated Sup35 NM aggregates are detected in scraped cell suspensions as a function of increasing aTc concentration only for Round 1 (R1) clones producing wild-type ClpB. Sup35 NM aggregates are not detected at any aTc concentration in scraped cell suspensions of R1 clones producing ClpB disaggregase mutants or in R1 clones lacking ClpB. Lanes cropped from the same immunoblot are indicated by hash marks. The α-His6X antibody recognizes the Sup35 NM-mCherry-His6X fusion protein. (B) Wild-type and mutant ClpB levels along with Sup35 NM fusion protein levels are comparable in R1 clones grown on solid medium supplemented with 50 ng/ml aTc as assessed by Western blot analysis. The α-Sup35 antibody recognizes the Sup35 NM-mCherry-His6X fusion protein, the α-GFP antibody recognizes the New1-mGFP fusion protein, the α-ClpB antibody recognizes the E. coli ClpB chaperone, and the α-RpoA antibody recognizes the α subunit of E. coli RNA polymerase.
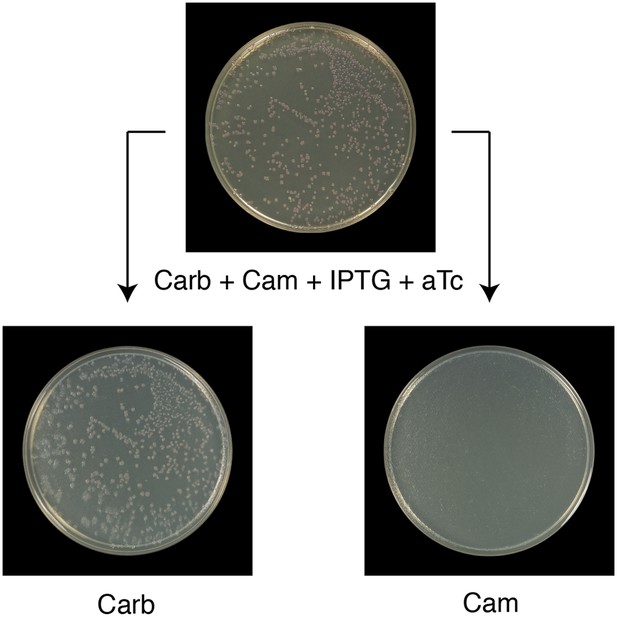
All Round 1 clones producing wild-type ClpB are cured of pSC101TS-NEW1-clpB.
All Round 1 (R1) clones derived from a starter culture of ΔclpB cells containing pBR322-NM SUP35, pSC101TS-NEW1-clpB, and chromosomal aTc-inducible wild-type clpB lose pSC101TS-NEW1-clpB as assessed by replica plating from solid medium supplemented with carbenicillin (Carb), chloramphenicol (Cam), IPTG, and 50 ng/ml aTc to solid medium containing either Carb or Cam. pSC101TS-NEW1-clpB confers Cam resistance.
Additional files
-
Supplementary file 1
Strains and Plasmids.
- https://doi.org/10.7554/eLife.02949.017