Re-visiting the trans insertion model for complexin clamping
Figures
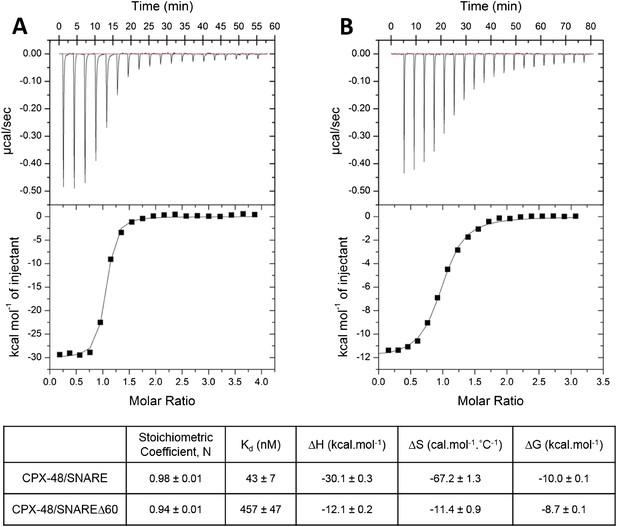
Characterization of interaction of complexin (CPX)-48 with pre- and post-fusion SNARE complex by isothermal titration calorimetry.
Representative thermograms of CPX-48 titrated into post-fusion SNARE (A) or pre-fusion SNARE∆60 complex (B). The solid lines represent the best fit to the corresponding data points using non-linear least squares fit with the one-set-of-sites model. The results of the fit from 2–3 independent trials are shown in Table 1.
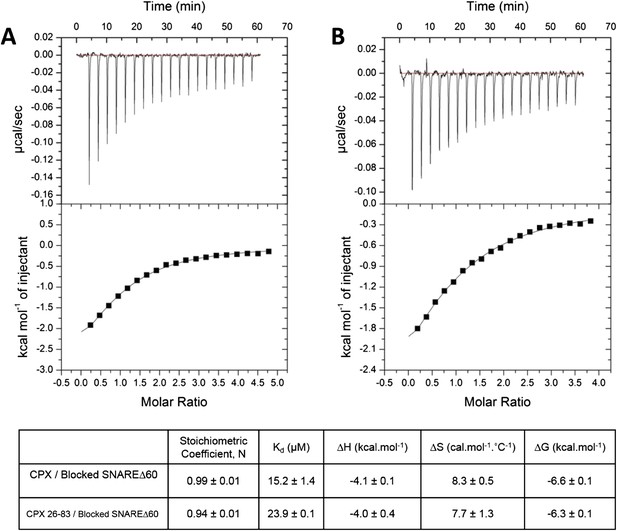
Interaction of complexin accessory helix (CPXacc) with the t-SNARE groove for full-length and truncated (residue 26–83) CPX characterized by isothermal titration calorimetry.
Full-length (A) or CPX26–83 (B) were titrated into pre-fusion SNAREΔ60 complex with the CPX central helix (CPXcen) binding site blocked with CPX-48 to exclusively measure the CPXacc–t-SNARE clamping interaction. The solid lines represent the best fit to the corresponding data points using non-linear least squares fit with one-set-of-sites-model and results of the fit are shown in Table 1. All experiments were conducted in triplicate and a representative thermogram is shown.
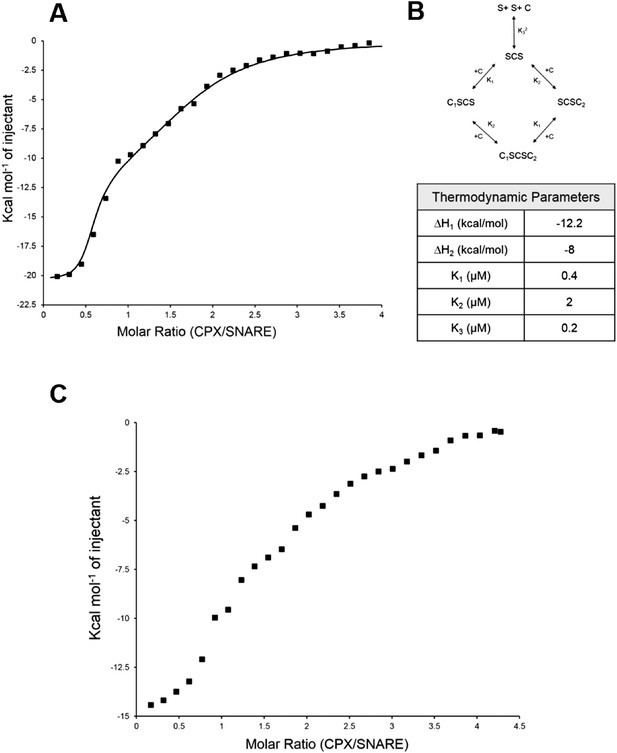
Isothermal titration calorimetry indicates multivalent interactions between SNAREΔ60 and CPX.
(A) Calorimetric titration of super-clamp complexin (scCPX; residues 1–134, with D27L, E34F, R37A mutations) into pre-fusion SNAREΔ60 complex describes a multi-site interaction of CPX. The solid lines represent the predicted binding thermogram assuming that both scCPX and truncated SNAREΔ60 are bivalent with well-defined independent thermodynamic parameters describing CPX central helix and CPX accessory helix binding (B). (C) Representative thermogram of full-length wild-type CPX (residues 1–134) titrated into unblocked SNAREΔ60.
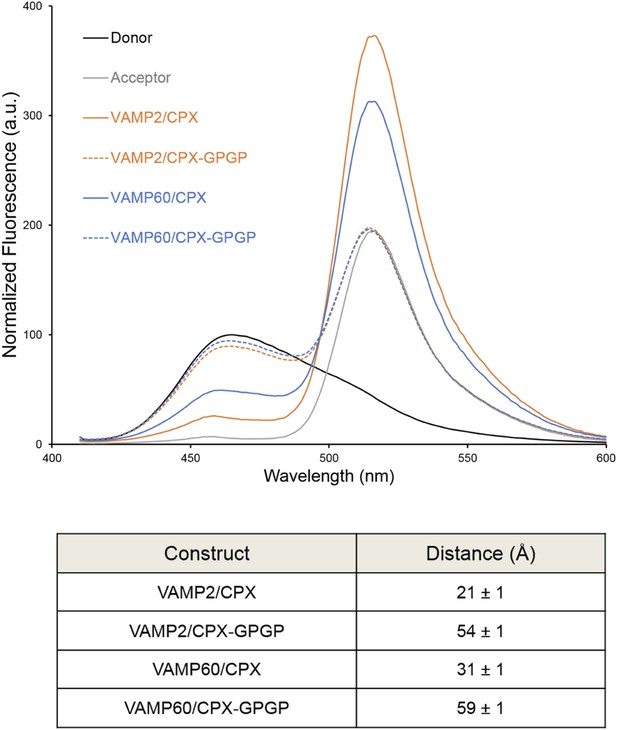
Bimane–Oregon green fluorescence resonance energy transfer (FRET) experiments probing the effect of flexibility on complexin (CPX) orientation in pre- and post-fusion CPX–SNARE complexes.
The FRET labeling positions were residue 193 on SNAP25 (Bimane) and residue 38 on CPX (Oregon green). Fluorescence emission spectra of Bimane only (black), Oregon green only (grey), and Bimane–Oregon green labeled CPX–SNARE complexes containing VAMP2 (residues 25–96, orange), VAMP60 (residues 25–60, blue) confirms the angled (low FRET) configuration of CPX accessory helix (CPXacc) in the pre-fusion SNARE complex (VAMP60). The near complete loss of FRET for the flexible CPX mutant (helix breaking GPGP insertion between CPXacc and CPC central helix (CPXcen); CPX–GPGP, dashed lines) compared with the wild-type (WT, solid lines) in both pre- and post-fusion SNARE complexes shows that the difference in FRET signal observed with WT CPX is not due to increased flexibility of the CPXacc in the pre-fusion complex. FRET distances in these CPX–SNARE complexes determined from the quenching of the donor (Bimane) fluorescence is shown (table) and standard deviations are reported from 2–3 independent experiments.
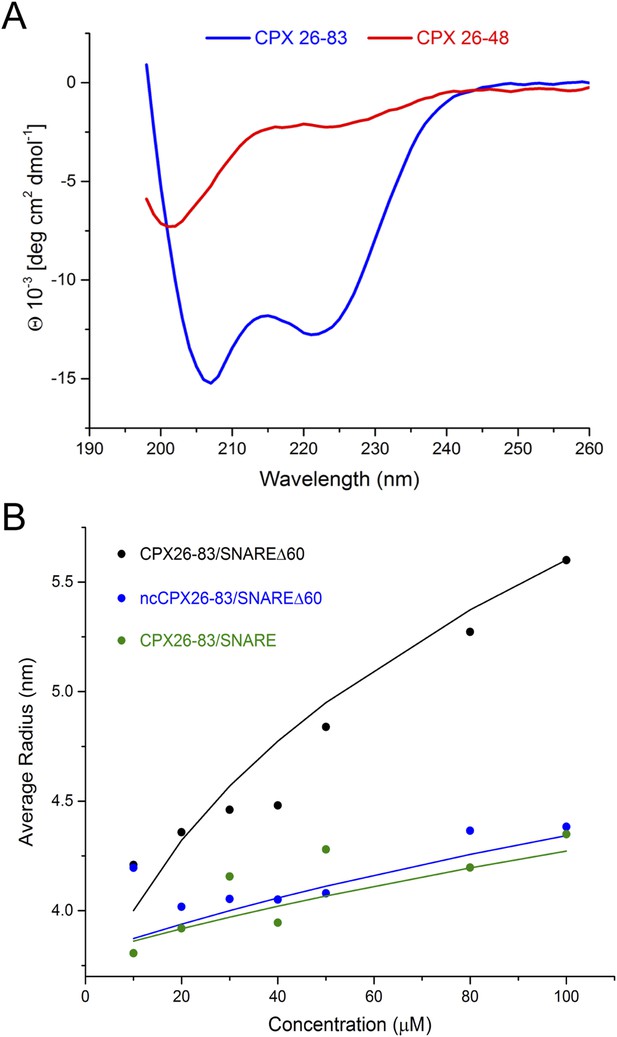
Circular dichroism (CD) and dynamic light scattering (DLS) experiments.
(A) CD spectra of the complexin (CPX) accessory domain (CPX26–48) and the CPX minimal functional domain (CPX26–83). The continuous CPX construct (26–83, blue) with uninterrupted accessory and central domain shows the characteristics of an α-helical protein. In contrast, the isolated CPX accessory domain (26–48) shows little secondary structure and appears mostly unfolded. This may explain why no interaction between the CPX accessory region and SNAREΔ60 was observed by NMR. (B) DLS analysis showing the formation of the high-order oligomers of CPX–SNAREΔ60 in the concentration range used in the NMR experiments in Trimbuch et al. (2014). Experimental average particle radius of pre-formed CPX26–83/SNAREΔ60 (black dots), ncCPX26–83/SNAREΔ60 (blue dots), and CPX26–83/SNARE (green dots) at varying concentration is shown. The solid lines (same color scheme) represent the average gyration radius of the oligomers calculated from the semi-quantitative model described in ‘Materials and methods’.
Tables
Affinity constants (Kd) for complexin (CPX) binding to SNARE complexes measured by isothermal titration calorimetry
Complexin | Binding partner | Binding affinity (Kd) | Reference |
---|---|---|---|
CPX1–134 | Ternary SNARE | 19 nM | Pabst et al. (2002) |
CPX48–134 | Ternary SNARE | 43 ± 7 nM | This study |
CPX48–134 | SNAREΔ60 | 457 ± 47 nM | This study |
CPX1–134 | Blocked SNAREΔ60 (1.5-fold excess of CPX48–134) | 16 µM | Kümmel et al. (2011) |
Super-clamp CPX1–134 (D27L E34F R37A) | Blocked SNAREΔ60 (1.5-fold excess of CPX48–134) | 2 µM | Kümmel et al. (2011) |
CPX1–134 | Blocked SNAREΔ60 (3-fold excess of CPX48–134) | 15.2 ± 1.4 µM | Current study |
CPX26–83 | Blocked SNAREΔ60 (3-fold excess of CPX48–134) | 23.9 ± 0.1 µM | Current study |