Cancer: How the immune system spots tumors
The immune system is best known for its role in defending the body against microbial infections. People carrying mutations that affect the immune system are, therefore, highly susceptible to infectious diseases and usually require continuous care to avoid infections. However, it has also been proposed that the immune system can eliminate cancer cells. This idea, known as immune surveillance, is several decades old, but there is little experimental evidence to support it. In large part, this is because the mechanisms used by the body to recognize cancer cells remain poorly understood.
A key feature of the immune system is its ability to distinguish between pathogens (‘non-self’) and the body's own cells (‘self’). Immune cells detect certain components that are found in many microorganisms, such as the complex carbohydrates that make up bacterial and fungal cell walls. Because these components are unique to microbes, detecting them allows the innate immune response—the body's first line of defence—to identify the cell they came from as ‘non-self’ and potentially harmful, and then act to neutralize the threat.
The carbohydrates are recognized by the so-called pattern-recognition receptors, such as Toll-like receptors and C-type lectin receptors, which are found in two types of immune cell—dendritic cells and macrophages (Takeuchi and Akira, 2010). Pathogens cannot easily evade detection because the components that are recognized by the receptors are essential for microbial fitness and survival.
This approach cannot be used to detect tumors because they develop from the host's own cells. Rather, in order to perform immune surveillance the immune system has to be able to distinguish tumor cells from normal cells. One way that this would be possible is if cancer cells produce signals that can be detected by the immune system. Clearly, if such signals exist, they would be the first feature to be lost by the cancer cells as they evolve under the selection pressure imposed by immune surveillance. Unless, that is, getting rid of these signals comes with a significant cost to the cancer cell.
Now, in eLife, Tadatsugu Taniguchi of the University of Tokyo and co-workers—including Shiho Chiba, Hiroaki Ikushima and Hiroshi Ueki as joint first authors—report evidence that immune surveillance does indeed rely on this strategy. They report that the pattern-recognition receptor Dectin-1 plays a crucial role in recognizing a process called tumor cell-associated glycosylation and in initiating an anti-tumor innate immune response (Chiba et al., 2014).
Glycosylation is a reaction where a carbohydrate molecule called a glycan is added to a protein. Altered glycosylation is a universal feature of tumor cells (Varki et al., 2009). By increasing where and how much glycosylation occurs on their surface, many tumor cells develop an advantage over other cells when migrating to and spreading through other organs.
Chiba et al. found that two proteins play crucial roles in suppressing the spread of cancer: Dectin-1 and IRF5 (Figure 1). Dectin-1 recognizes molecules called β-glucans that are found on fungal cell walls (Brown, 2006). When Dectin-1 recognizes its target, the transcription factor IRF5 activates innate immunity in response (del Fresno et al., 2013). Chiba et al. now show that Dectin-1 also recognizes particular glycosylation associated structures called N-glycan structures, which are highly expressed in several tumor cell lines—but not in non-cancerous cells. When N-glycan structures are detected, Dectin-1 triggers an anti-tumor immune response.
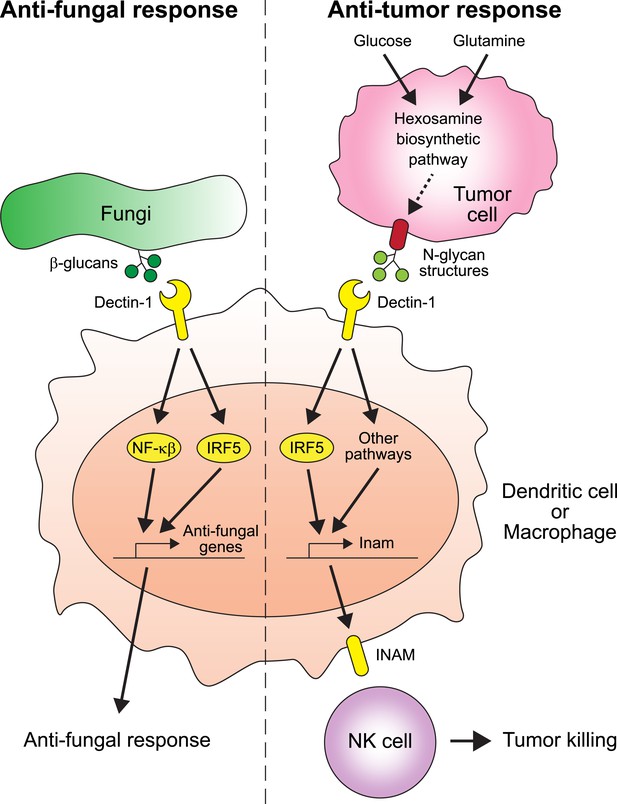
The receptor protein Dectin-1 plays an important role in the anti-fungal and anti-tumor responses of the innate immune system.
Left: Dectin-1 is expressed on dendritic cells and macrophages, and recognizes molecules called β-glucans (dark green circles) on fungal cell walls. This recognition triggers the signaling pathways that activate transcription factors including IRF5 and NF-κβ (del Fresno et al., 2013), which cause anti-fungal genes to be expressed. Right: Chiba et al. found that Dectin-1 can also recognize N-glycan structures expressed on certain tumor cell lines. Recognizing these tumor cell signals activates IRF5 and perhaps other unidentified pathways, which causes tumoricidal action by natural killer (NK) cells. At least part of the mechanism for natural killer cell mediated tumor killing involves increasing the expression of the Inam gene. It would be interesting in the future to determine if Dectin-1 recognizes N-glycan structures as a proxy for the increased activity of the hexosamine biosynthetic pathway in tumor cells.
Natural killer (NK) cells are lymphocytes (or white blood cells) that can kill cancer cells and so could play an important role in immune surveillance (Vivier et al., 2012). Chiba et al. found in their experimental model that natural killer cells are indeed required for the elimination of tumor cells. Furthermore, natural killer cells rely on Dectin-1 expressed on dendritic cells and macrophages in order to kill tumor cells; the findings therefore reveal a new functional link between dendritic cells, macrophages and natural killer cells.
Chiba et al. have taken an important step towards understanding how the anti-tumor immune response works. As is normal for a brand-new finding, this work raises a number of important questions. The level and/or the specific structural patterns of glycosylation on tumor cells appears to be important for the fitness of cancer cells. Therefore, an immune surveillance system based on cell surface glycan recognition may have evolved to prevent cancer cells themselves evolving in a way that enables them to evade the anti-tumor immune response. In this regard, it would be important to know whether Dectin-1 is activated by either an altered structure or increased level of N-glycans on tumor cells.
It is possible that the total level or specific structural variants of cell surface N-glycans may reflect the metabolic state of the cell. For example, the hexosamine biosynthetic pathway—which is used by cells to produce a particular type of sugar—is more active than normal in some cancers and can affect the glycosylation patterns of cell surface proteins (Wellen et al., 2010). It would be very interesting to investigate whether Dectin-1 (and other C-type lectins) evolved to detect the increased activity of the hexosamine biosynthetic pathway through its effect on cell surface N-glycans, as a proxy for cancer cells (Figure 1).
References
-
Dectin-1: a signalling non-TLR pattern-recognition receptorNature Reviews Immunology 6:33–43.https://doi.org/10.1038/nri1745
-
Targeting natural killer cells and natural killer T cells in cancerNature Reviews Immunology 12:239–252.https://doi.org/10.1038/nri3174
Article and author information
Author details
Publication history
Copyright
© 2014, Okabe and Medzhitov
This article is distributed under the terms of the Creative Commons Attribution License, which permits unrestricted use and redistribution provided that the original author and source are credited.
Metrics
-
- 5,509
- views
-
- 433
- downloads
-
- 6
- citations
Views, downloads and citations are aggregated across all versions of this paper published by eLife.
Download links
Downloads (link to download the article as PDF)
Open citations (links to open the citations from this article in various online reference manager services)
Cite this article (links to download the citations from this article in formats compatible with various reference manager tools)
Further reading
-
- Cancer Biology
- Immunology and Inflammation
The immunosuppressive microenvironment in pancreatic ductal adenocarcinoma (PDAC) prevents tumor control and strategies to restore anti-cancer immunity (i.e. by increasing CD8 T-cell activity) have had limited success. Here, we demonstrate how inducing localized physical damage using ionizing radiation (IR) unmasks the benefit of immunotherapy by increasing tissue-resident natural killer (trNK) cells that support CD8 T activity. Our data confirms that targeting mouse orthotopic PDAC tumors with IR together with CCR5 inhibition and PD1 blockade reduces E-cadherin positive tumor cells by recruiting a hypoactive NKG2D-ve NK population, phenotypically reminiscent of trNK cells, that supports CD8 T-cell involvement. We show an equivalent population in human single-cell RNA sequencing (scRNA-seq) PDAC cohorts that represents immunomodulatory trNK cells that could similarly support CD8 T-cell levels in a cDC1-dependent manner. Importantly, a trNK signature associates with survival in PDAC and other solid malignancies revealing a potential beneficial role for trNK in improving adaptive anti-tumor responses and supporting CCR5 inhibitor (CCR5i)/αPD1 and IR-induced damage as a novel therapeutic approach.
-
- Immunology and Inflammation
During thymic development, most γδ T cells acquire innate-like characteristics that are critical for their function in tumor surveillance, infectious disease, and tissue repair. The mechanisms, however, that regulate γδ T cell developmental programming remain unclear. Recently, we demonstrated that the SLAM/SAP signaling pathway regulates the development and function of multiple innate-like γδ T cell subsets. Here, we used a single-cell proteogenomics approach to identify SAP-dependent developmental checkpoints and to define the SAP-dependent γδ TCR repertoire in mice. SAP deficiency resulted in both a significant loss of an immature Gzma+Blk+Etv5+Tox2+ γδT17 precursor population and a significant increase in Cd4+Cd8+Rorc+Ptcra+Rag1+ thymic γδ T cells. SAP-dependent diversion of embryonic day 17 thymic γδ T cell clonotypes into the αβ T cell developmental pathway was associated with a decreased frequency of mature clonotypes in neonatal thymus, and an altered γδ TCR repertoire in the periphery. Finally, we identify TRGV4/TRAV13-4(DV7)-expressing T cells as a novel, SAP-dependent Vγ4 γδT1 subset. Together, the data support a model in which SAP-dependent γδ/αβ T cell lineage commitment regulates γδ T cell developmental programming and shapes the γδ TCR repertoire.