Dynamic subcellular localization of a respiratory complex controls bacterial respiration
Figures
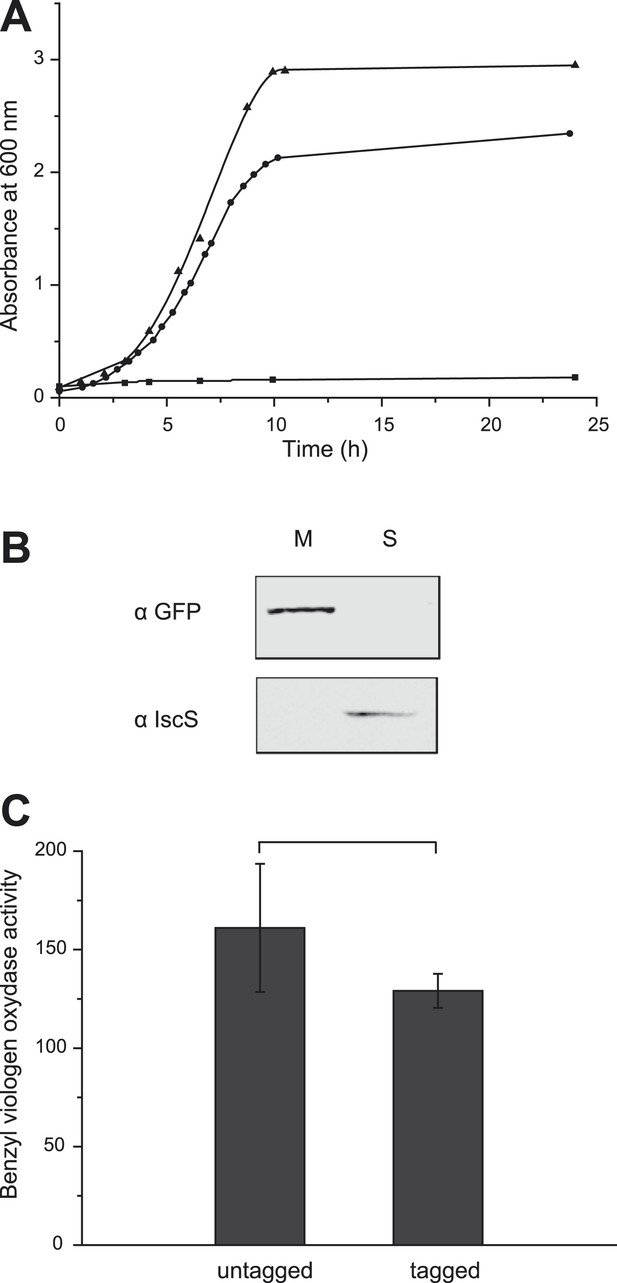
The GFP-labeled nitrate reductase complex is active and fully assembled
.(A) Growth curves of E. coli strains expressing untagged or tagged-NarGHI under nitrate-respiring conditions. Cells were grown anaerobically in a minimal medium using glycerol as sole carbon source and nitrate as terminal electron acceptor. JCB4011 strain expresses the untagged NarGHI complex (▲), whereas the LCB3635 strain expresses the GFP-tagged NarGHI complex (●). As a negative control, the nitrate reductase-deficient strain JCB4023 shows no growth under these conditions (■). The estimated generation time is about 80 ± 10 min for JCB4011 and 110 ± 10 min for LCB3635. (B) The GFP-tagged NarGHI complex is correctly localized to the membrane. Western blots were performed with antibodies raised against eGFP or IscS as a marker of soluble proteins on soluble (S) or membrane (M) fractions prepared from nitrate-respiring cells. (C) The activity of the NarGH catalytic module is unaffected by the eGFP fusion. Benzyl viologen:nitrate oxidoreductase activity assays were performed on membranes prepared from JCB4011 (untagged version) or LCB3635 (GFP-tagged version) cells grown under nitrate-respiring conditions and expressed in µmoles of nitrite produced min−1 mg−1 of nitrate reductase.
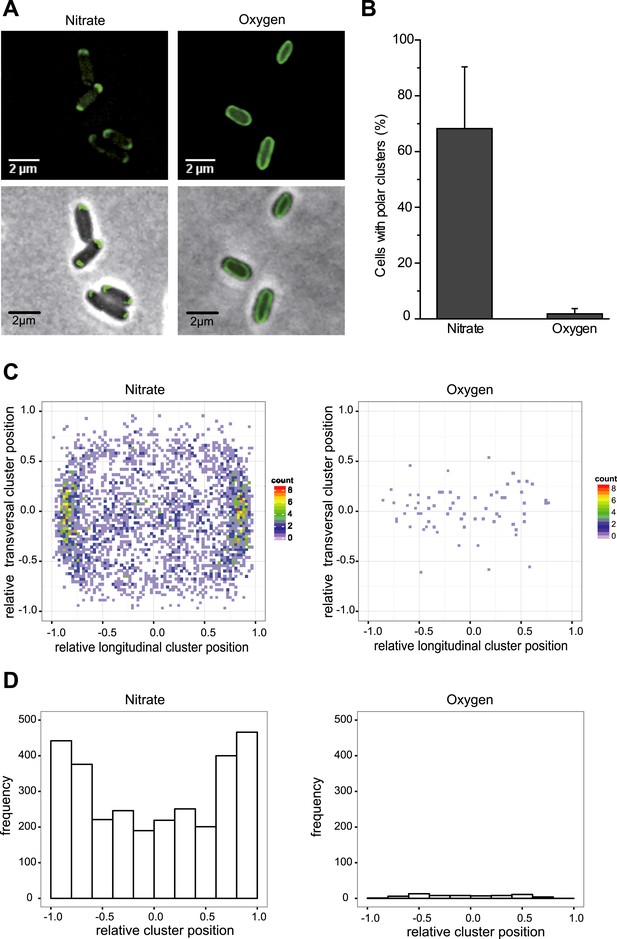
The GFP-labeled nitrate reductase complex concentrates at the cell poles under nitrate-respiring conditions.
(A) Fluorescence images (top) and overlays of fluorescence and phase contrast images (bottom) are shown for nitrate-respiring and oxygen-respiring cells. The deconvolved image of the fluorescence signal is shown in green, and the cell outline is shown by phase contrast. (B) Mean frequency of cells displaying clusters at the cell poles for nitrate-respiring or oxygen-respiring cells. (C) A density map was built from a two-dimensional histogram (relative transversal cluster position vs relative longitudinal cluster position) of the fluorescence signal observed in nitrate-respiring or oxygen-respiring LCB3635 cells. The color map shows the interpolated density of clusters positions. The dots represent the individual clusters (a little jittering was added to avoid overlapping of the dots). (D) The histogram of the fluorescence signal clusters across the transversal axis of nitrate-respiring or oxygen-respiring LCB3635 cells is shown. In both conditions, more than 500 cells were analyzed. Under nitrate-respiring conditions, a strong enrichment of the clusters is observed at the cell poles. Under oxygen-respiring conditions, only few cells exhibit clusters which have an even distribution along the cell axis.
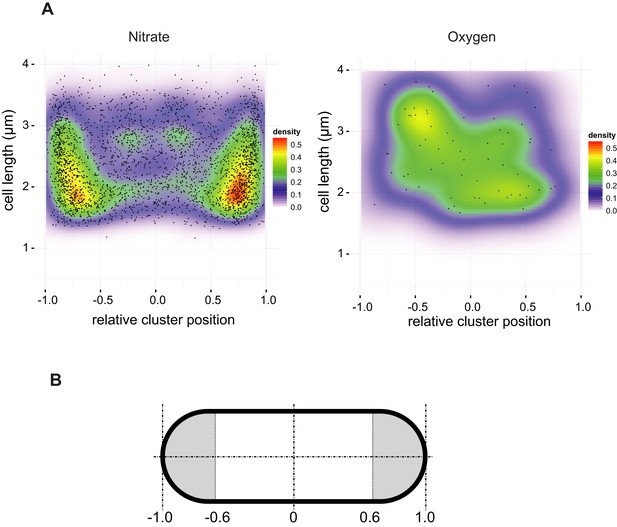
Spatial distribution of clusters.
(A) A density map was built from a two-dimensional histogram (cell length vs relative cluster position) of the fluorescence signal observed in nitrate-respiring or oxygen-respiring LCB3635 cells. The color map shows the interpolated density of clusters positions. The dots represent the individual clusters (a little jittering was added to avoid overlapping of the dots). (B) The ‘poles’ were defined according to the distribution of clusters along the cell axis in Figure 2D. The limit was set to ± 0.6 and used subsequently throughout the article.
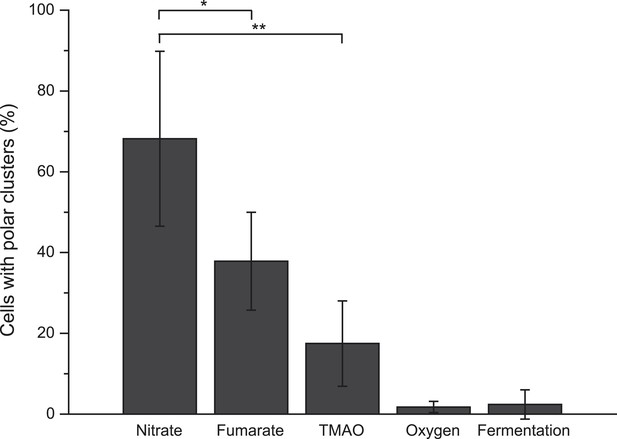
Metabolism-dependent localization of the nitrate reductase OXPHOS complex.
Mean frequency of LCB3635 cells displaying clusters at the cell poles for various metabolic conditions (anaerobic respiration with either nitrate, fumarate or TMAO as terminal electron acceptor, aerobic respiration and anoxic fermenting conditions). Non-fermentable glycerol was used as sole carbon and electron source in all cases with the exception of fermenting conditions where it was replaced for glucose. Polar localization is only observed under anaerobic respiration whatever the terminal electron acceptor used.
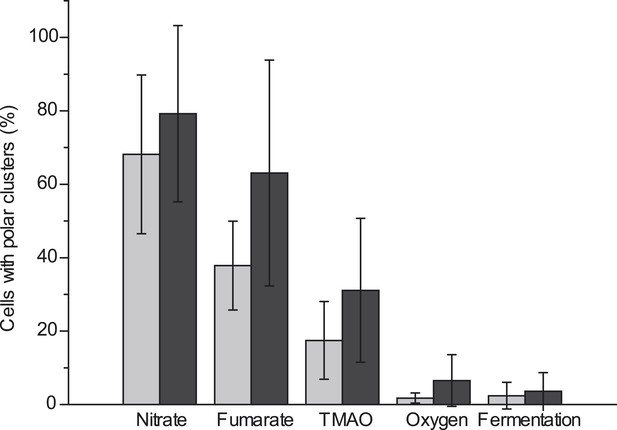
Comparative analysis of cells displaying clusters at the cell poles vs cells displaying clusters along the cell body upon varying metabolic conditions.
(Gray bars) Mean frequency of LCB3635 cells displaying clusters of the fluorescence signal at the cell poles. (Black bars) Mean frequency of LCB3635 cells displaying clusters of the fluorescence signal along the entire cell body for various metabolic conditions. The conditions tested were: anaerobic respiration with either nitrate, fumarate or TMAO as terminal electron acceptor, aerobic respiration, and anoxic fermenting conditions.
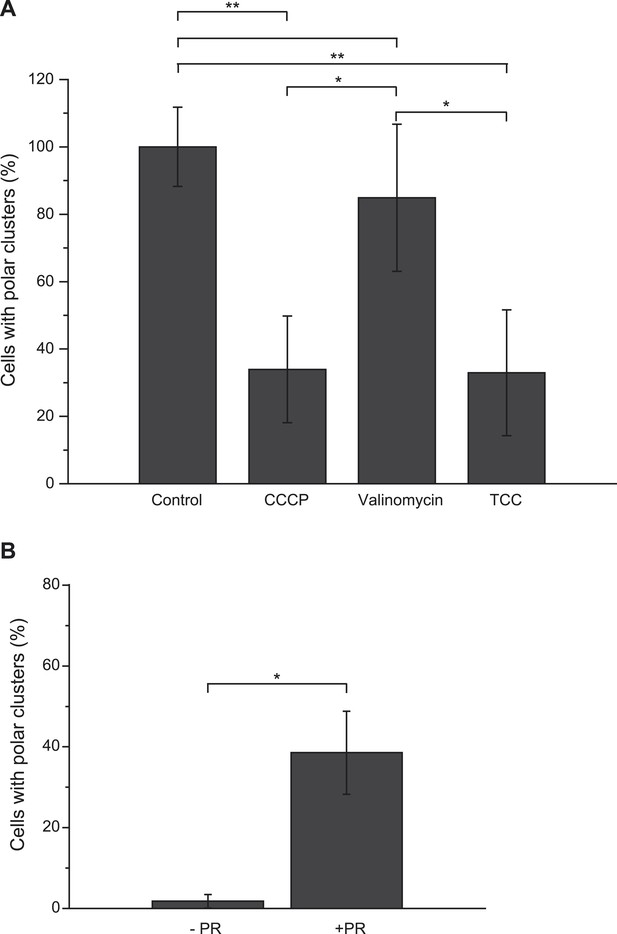
Proton gradient is a cue for polar localization of the anaerobic OXPHOS complex.
(A) The pmf is important for polar localization of the nitrate reductase complex. Shown is the mean frequency of nitrate-respiring LCB3635 cells displaying clusters at the cell poles upon addition of the indicated ionophores. Each value was normalized with respect to that of the untreated cells (labeled as control) and expressed in % of the control. Images were taken 15 min after the treatment with the ionophore. (B) Establishment of an artificial proton concentration gradient in anoxic fermenting growing cells is sufficient to restore the polar localization. Shown is the mean frequency of fermenting growing LCB3635 cells displaying clusters at the cell poles in the absence (-PR) or presence of the proteorhodopsin (+PR).
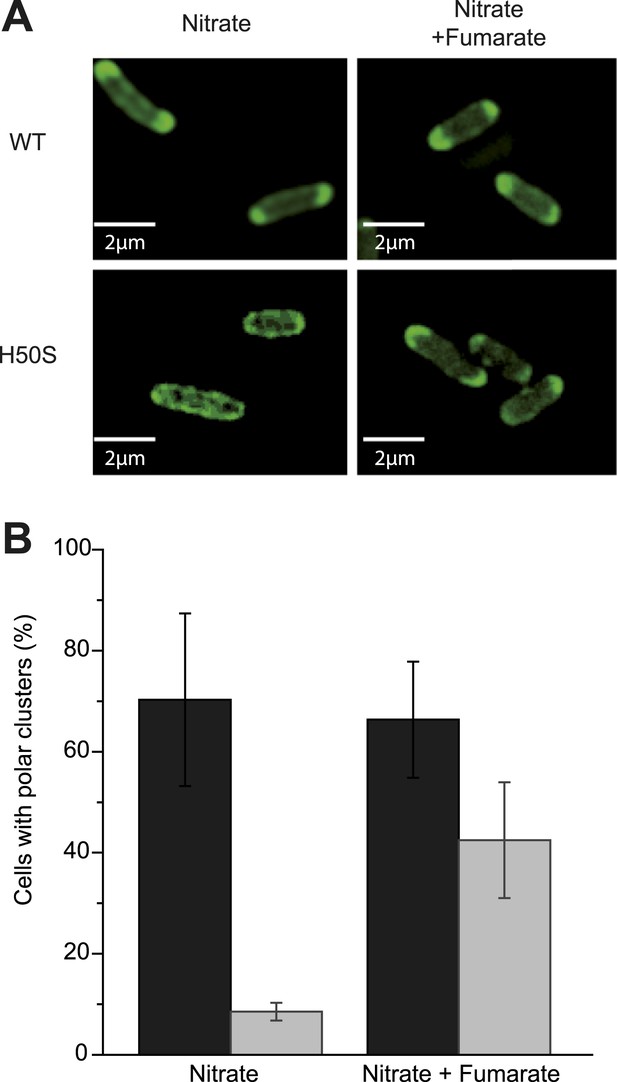
Activity of the nitrate reductase OXPHOS complex is not essential for polar positioning unless a ΔpH is established.
(A) Fluorescence images of JCB4023/pVA70GFP cells expressing active (top) or inactive NarG-H50S variant of the GFP-labeled nitrate reductase (bottom) are shown. Cells were grown anaerobically either in glycerol-nitrate (nitrate) or glycerol-nitrate-fumarate (nitrate + fumarate) medium. (B) Mean frequency of cells displaying clusters at the cell poles under nitrate or nitrate–fumarate conditions. (Black bars) JCB4023/pVA70GFP cells expressing the active complex. (Gray bars) JCB4023/pVA70GFPH50S cells expressing the inactive variant.
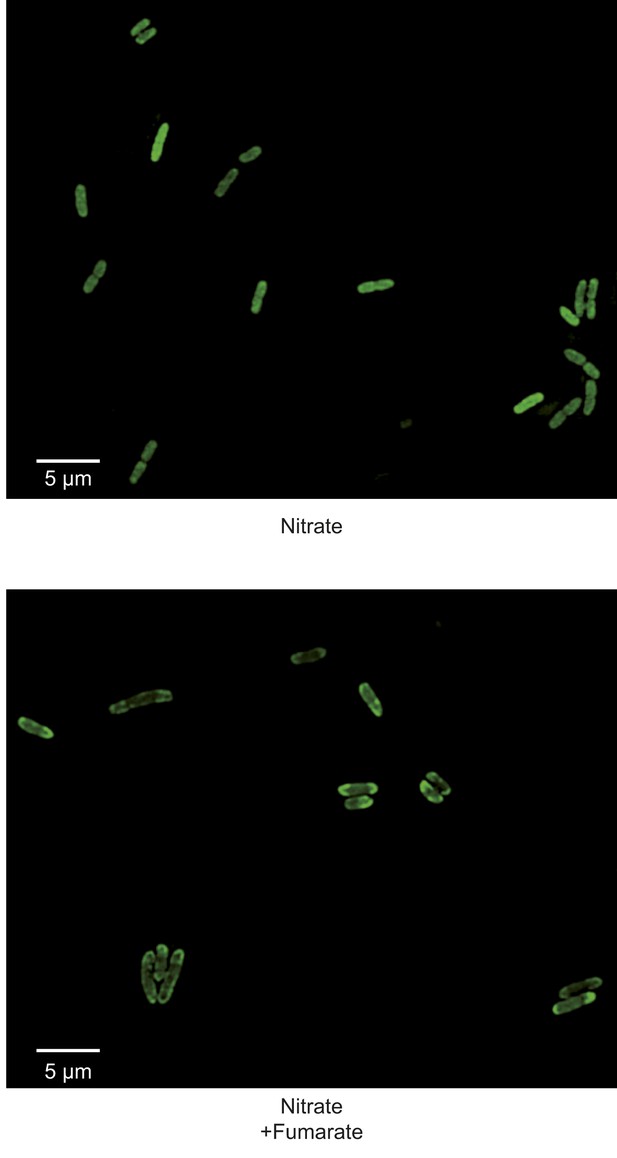
Activity of the nitrate reductase OXPHOS complex is not essential for polar positioning unless a ΔpH is established.
Fluorescence images of JCB4023/pVA70GFP cells expressing inactive NarG-H50S variant of the GFP-labeled nitrate reductase and grown anaerobically either in glycerol-nitrate (nitrate) or glycerol-nitrate-fumarate (nitrate + fumarate) medium.
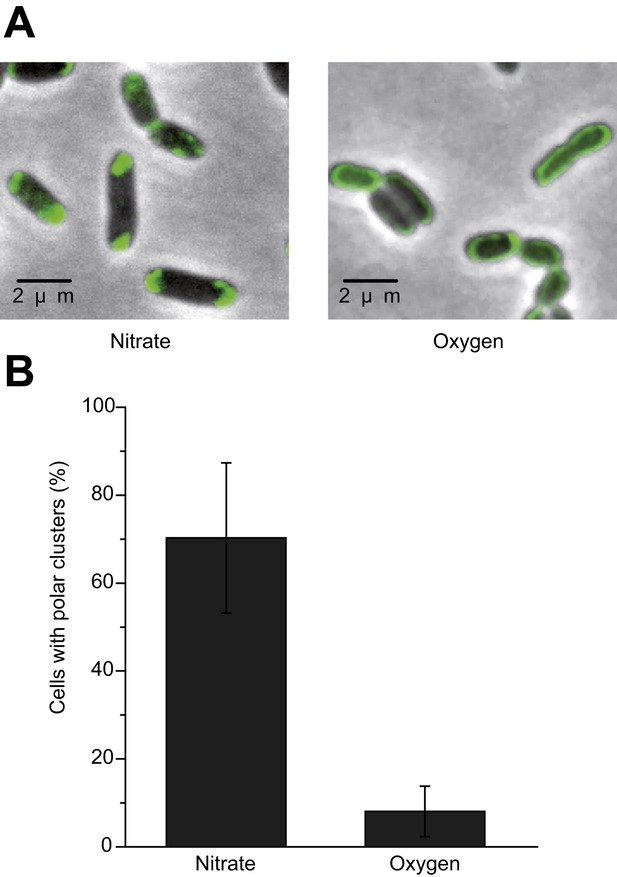
Cells expressing the GFP-labeled nitrate reductase complex from the pVA70GFP plasmid show identical metabolism-dependent localization of the complex.
(A) Overlays of fluorescence and phase contrast images are shown for nitrate-respiring and oxygen-respiring JCB4023 cells expressing the GFP-tagged NarGHI complex from the pVA70GFP plasmid. (B) Mean frequency of cells displaying clusters at the cell poles for nitrate-respiring or oxygen-respiring cells.
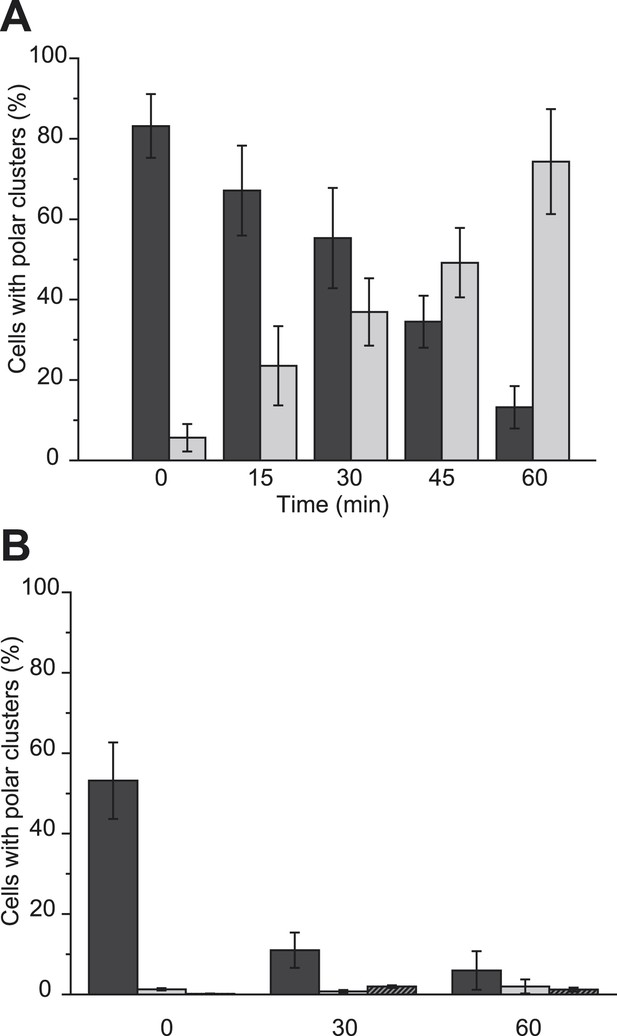
Metabolism-dependent localization changes occur in a timeframe of several tens of minutes.
(A) (Black bars) Nitrate-respiring JCB4023/pVA70GFP cells were submitted to strong aeration and the fluorescence pattern was evaluated at 15-min intervals. (Gray bars) Oxygen-respiring JCB4023/pVA70GFP cells were shifted to anoxic conditions in presence of nitrate. In both cases, shown is the mean frequency of cells displaying clusters at the cell poles. (B) (Black bars) Upon chloramphenicol (Cm) addition, nitrate-respiring 3635/pBAD24 cells were submitted to strong aeration and the fluorescence pattern was evaluated at 30-min intervals. (Gray bars) Upon Cm addition, oxygen-respiring 3635/pBAD24 cells were shifted to anoxic conditions in presence of nitrate. (Shaded bars) Upon Cm addition, oxygen-respiring 3635/pPR cells were shifted to anoxic conditions in presence of nitrate. In all cases, shown is the mean frequency of cells displaying clusters at the cell poles.
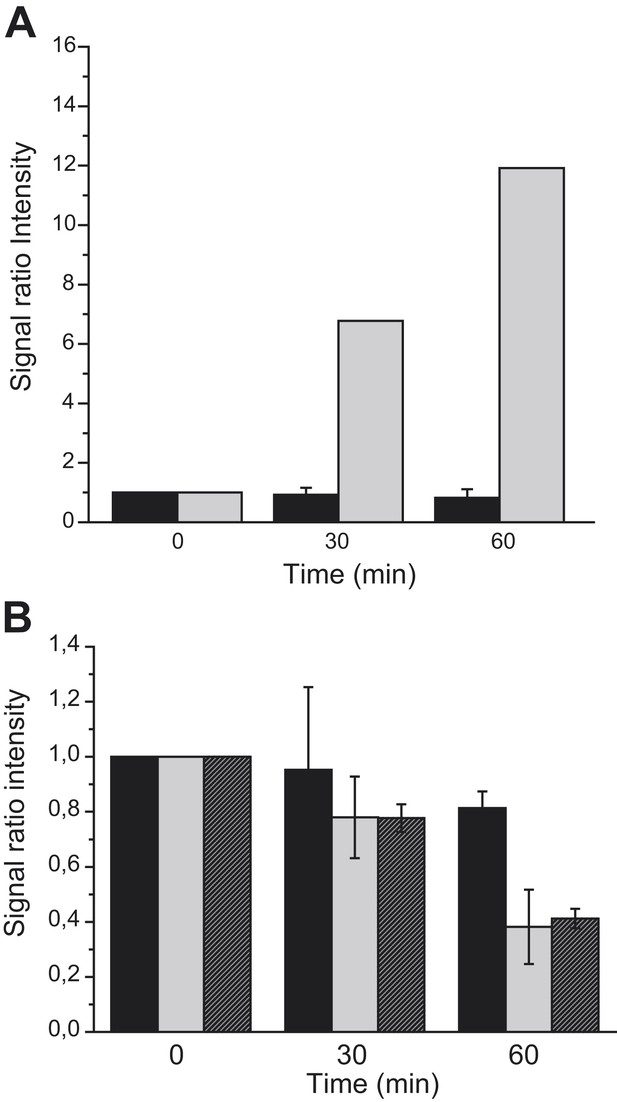
Metabolism-dependent localization changes occur in a timeframe of several tens of minutes.
(A) Changes in GFP-labeled nitrate reductase content as estimated by immunoblotting. (Black bars) anoxic–oxic transition. (Gray bars) oxic–anoxic transition. (B) Changes in GFP-labeled nitrate reductase content as estimated by immunoblotting (Black bars) anoxic–oxic transition of 3635/pBAD24 cells. (Gray bars) oxic–anoxic transition 3635/pBAD24 cells. (Shaded bars) oxic–anoxic transition 3635/pPR cells. The mean GFP-labeled nitrate reductase content was normalized using IscS as internal control and expressed as signal ratio intensity.
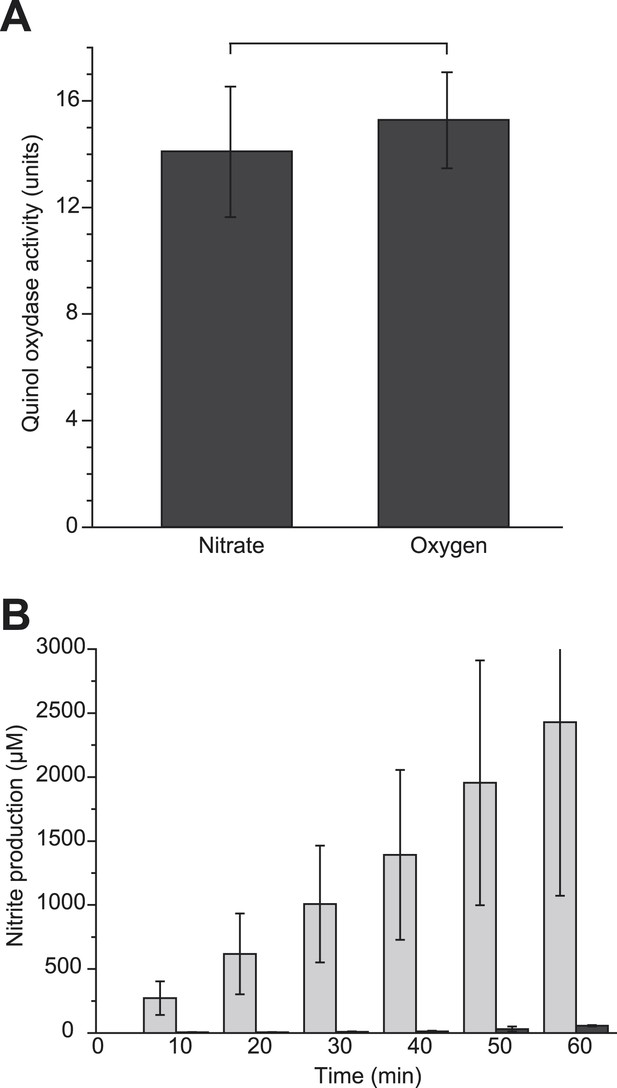
Polar localization determines integration of the nitrate reductase complex in anaerobic respiratory chains.
(A) Constant activity of the GFP-tagged complex upon distinct subcellular localization. Quinol:nitrate oxidoreductase activity was measured on membranes prepared from oxygen-respiring or nitrate-respiring JCB4023 cells expressing the GFP-tagged NarGHI complex from the pVA70GFP plasmid. Activities are expressed in µmoles of nitrite produced min−1 mg−1 of nitrate reductase. (B) Enhanced nitrite production in cells with enforced polar localization of the GFP-NarGHI complex. At time 0, nitrate (100 mM final concentration) was added to anoxic fermenting growing LCB3635 cells expressing (+PR, light grey) or not expressing (−PR, black) the proteorhodopsin. Subsequently, nitrite production was detected in the cell culture over time using the Griess reaction. The indicated values were derived from raw data using a suitable standard curve and represented the means of three distinct experiments.
Additional files
-
Supplementary file 1
List of strains, plasmids, and oligonucleotides.
- https://doi.org/10.7554/eLife.05357.015