Pheromones: The taste of togetherness
A wide range of organisms, including insects, can communicate with other organisms of the same species using sounds, signs and chemicals. The chemical cues emitted by organisms are called pheromones, and they trigger important behaviors like courtship, aggression, aggregation and aversion. The behavior produced by a pheromone depends on the chemical released and the social context in which it is detected.
Understanding how pheromones drive such powerful behaviors can be studied in depth in the fruit fly Drosophila melanogaster because its relatively simple nervous system makes it easy to investigate the neural circuits that detect and respond to these chemical signals. Several important principles have emerged from extensive studies of pheromone signaling in adult fruit flies, including an understanding of how pheromones are detected and how they can influence behavior in male and female flies in different ways. However, most of this work has focused on a pheromone called cis-vaccenyl acetate (van der Goes van Naters and Carlson, 2007). Now in eLife, Joshua Mast and colleagues from the Janelia Farm Research Campus, ETH Zürich and the US Department of Agriculture have used the chemosensory system of fruit fly larvae to identify new pheromones and the neurons that detect them (Mast et al., 2014).
D. melanogaster larvae have chemosensory (chemical detection) systems that are much simpler than those found in adults. This, along with the simple behavior of the larvae, makes them very suitable for studying pheromones. D. melanogaster larvae gather together on food sources, suggesting that they may produce pheromones that cause them to aggregate (Durisko et al., 2014). However, the identity of these pheromones remained a mystery.
Mast and co-workers now show that D. melanogaster larvae are attracted to surfaces on which other larvae of the same species have crawled, and have found that this behavior is caused by a chemical cue deposited by the larvae. Detecting this cue requires two ion channels, called pickpocket23 and pickpocket29, that are expressed in the neurons of the terminal organ, which is the main taste organ of a larva (Figure 1). Taste neurons expressing these two ion channels are also known to detect sex pheromones in adult D. melanogaster flies (Lu et al., 2012; Thistle et al., 2012; Toda et al., 2012). The simplicity of the larval neural circuits allowed Mast et al. to narrow down the possible neurons that could detect pheromones to a single pair of neurons (R58F10+) in the terminal organ. This finding also provides a foundation for future investigations into the precise regions of the brain to which this pair of neurons sends information.
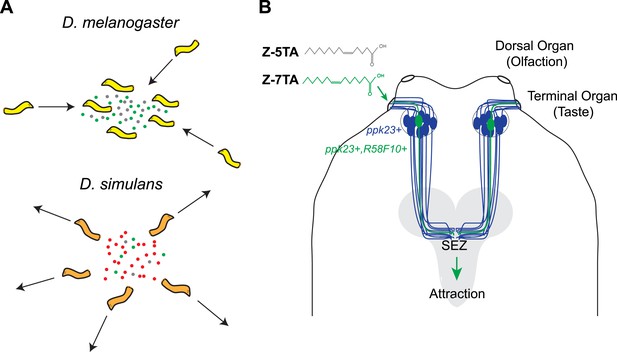
Different species of fruit fly larvae use pheromones to either find or avoid one another.
(A) Mast et al. reveal that the attractive pheromones (Z)-5-tetradecenoic acid (Z-5-TA; gray dots) and (Z)-7-tetradecenoic acid (Z-7-TA; green dots) are secreted by the larvae of D. melanogaster and cause other larvae to gather together (top). The larvae of D. simulans produce these pheromones at lower levels, and are thought to produce an additional compound (red dots) that repels other larvae (bottom). (B) Dorsal view of the ‘head’ of a D. melanogaster larva. A single pair (green) of the 10 pairs of pickpocket23-expressing neurons (blue) extends into the terminal organ and detects Z-5-TA and Z-7-TA. This information is then relayed to a collection of neurons in the subesophageal zone (SEZ) of the brain (grey).
Next, Mast et al. used gas chromatography to separate the attractive chemicals deposited by the larvae, and then used mass spectrometry to identify a small number of compounds. Examining how the larvae responded to synthetic versions of each compound identified two rare fatty acids—(Z)-5-tetradecenoic acid and (Z)-7-tetradecenoic acid—as being responsible for attracting the larvae to each other. The pair of pickpocket23-expressing neurons in the terminal organ is able to detect both of these chemicals.
During courtship, pheromones are often used to signal that both participants are of the same species (Savarit et al., 1999; Ferveur, 2005). Generally, members of different Drosophila species will not court flies from other species because, it is thought, each species has a characteristic pheromone profile (Coyne et al., 1994). This species-specific effect is also partially seen in response to the pheromones that cause the larvae to aggregate: D. melanogaster larvae will gather together to form aggregates, but the larvae of a closely related species, Drosophila simulans, will not (Durisko et al., 2014).
Mast et al. reasoned that these differences in behavior could have several underlying causes. D. simulans may have lost sensitivity to the pheromones that D. melanogaster larvae find attractive, or may produce different pheromones—or a combination of these possibilities. Tests revealed that the lack of aggregation in D. simulans larvae is likely the result of the reduced production of both of the attractive D. melanogaster pheromones detected by Mast et al., as well as the production of an additional unknown repellant compound.
The differences in pheromone signaling between species are of great interest in evolutionary biology, as they could be key for establishing new species by preventing separate species breeding with each other (Cobb and Hallon, 1990; Ferveur, 2010). The demonstration by Mast et al. that the larvae of different, closely related, fly species produce different pheromones suggests that pheromones are able to influence social behavior even at that early developmental stage. Exactly how these pheromones evolved along with their receptor systems and circuits, and how they participate in species-specific adaptations, remain open questions for future investigation.
References
-
Drosophila female courtship and mating behaviors: sensory signals, genes, neural structures and evolutionCurrent Opinion in Neurobiology 20:764–769.https://doi.org/10.1016/j.conb.2010.09.007
-
Genetic elimination of known pheromones reveals the fundamental chemical bases of mating and isolation in DrosophilaProceedings of the National Academy of Sciences of USA 96:9015–9020.https://doi.org/10.1073/pnas.96.16.9015
-
Receptors and neurons for fly odors in DrosophilaCurrent Biology 17:606–612.https://doi.org/10.1016/j.cub.2007.02.043
Article and author information
Author details
Publication history
Copyright
© 2014, Clark and Ray
This article is distributed under the terms of the Creative Commons Attribution License, which permits unrestricted use and redistribution provided that the original author and source are credited.
Metrics
-
- 1,391
- views
-
- 91
- downloads
-
- 0
- citations
Views, downloads and citations are aggregated across all versions of this paper published by eLife.
Download links
Downloads (link to download the article as PDF)
Open citations (links to open the citations from this article in various online reference manager services)
Cite this article (links to download the citations from this article in formats compatible with various reference manager tools)
Further reading
-
- Ecology
- Evolutionary Biology
Eurasia has undergone substantial tectonic, geological, and climatic changes throughout the Cenozoic, primarily associated with tectonic plate collisions and a global cooling trend. The evolution of present-day biodiversity unfolded in this dynamic environment, characterised by intricate interactions of abiotic factors. However, comprehensive, large-scale reconstructions illustrating the extent of these influences are lacking. We reconstructed the evolutionary history of the freshwater fish family Nemacheilidae across Eurasia and spanning most of the Cenozoic on the base of 471 specimens representing 279 species and 37 genera plus outgroup samples. Molecular phylogeny using six genes uncovered six major clades within the family, along with numerous unresolved taxonomic issues. Dating of cladogenetic events and ancestral range estimation traced the origin of Nemacheilidae to Indochina around 48 mya. Subsequently, one branch of Nemacheilidae colonised eastern, central, and northern Asia, as well as Europe, while another branch expanded into the Burmese region, the Indian subcontinent, the Near East, and northeast Africa. These expansions were facilitated by tectonic connections, favourable climatic conditions, and orogenic processes. Conversely, aridification emerged as the primary cause of extinction events. Our study marks the first comprehensive reconstruction of the evolution of Eurasian freshwater biodiversity on a continental scale and across deep geological time.
-
- Evolutionary Biology
Gene duplication drives evolution by providing raw material for proteins with novel functions. An influential hypothesis by Ohno (1970) posits that gene duplication helps genes tolerate new mutations and thus facilitates the evolution of new phenotypes. Competing hypotheses argue that deleterious mutations will usually inactivate gene duplicates too rapidly for Ohno’s hypothesis to work. We experimentally tested Ohno’s hypothesis by evolving one or exactly two copies of a gene encoding a fluorescent protein in Escherichia coli through several rounds of mutation and selection. We analyzed the genotypic and phenotypic evolutionary dynamics of the evolving populations through high-throughput DNA sequencing, biochemical assays, and engineering of selected variants. In support of Ohno’s hypothesis, populations carrying two gene copies displayed higher mutational robustness than those carrying a single gene copy. Consequently, the double-copy populations experienced relaxed purifying selection, evolved higher phenotypic and genetic diversity, carried more mutations and accumulated combinations of key beneficial mutations earlier. However, their phenotypic evolution was not accelerated, possibly because one gene copy rapidly became inactivated by deleterious mutations. Our work provides an experimental platform to test models of evolution by gene duplication, and it supports alternatives to Ohno’s hypothesis that point to the importance of gene dosage.