The critical role of membralin in postnatal motor neuron survival and disease
Figures
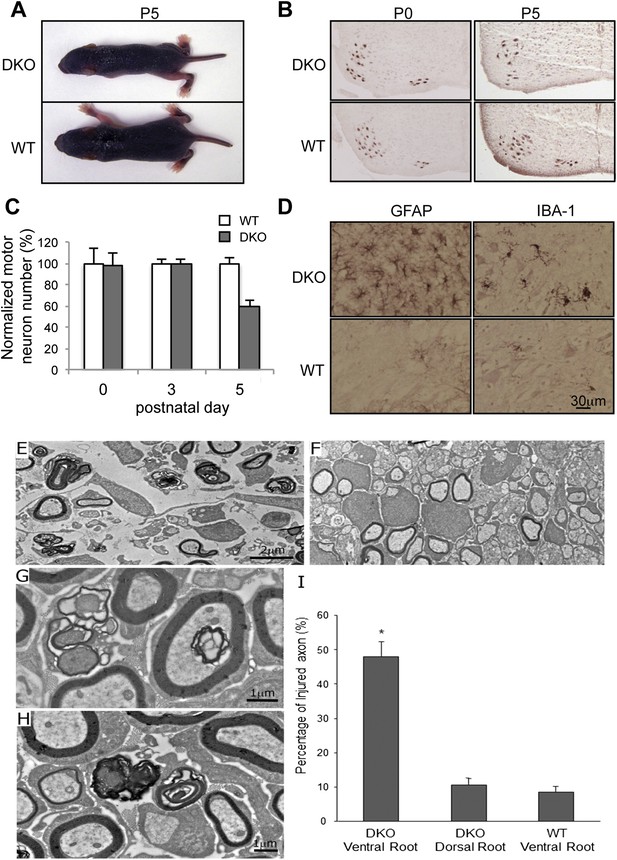
Motor neuron death in GluN3B/Membralin DKO mice.
(A) DKO and WT littermate mice are shown at P5. The DKO mouse was unable to maintain correct posture. (B) Immunostaining with anti-Hb9 antibody revealed a dramatic decrease in the number of motor neurons in the lumbar spinal cord of DKO mice relative to WT littermate mice at P5 but not at P0. (C) The number of motor neurons in the lumbar spinal cord of KO and WT mice was not significantly different at P0 and P3. However, severe motor neuron loss was observed by P5 in DKO mice (n = 3) compared with WT mice (n = 3, *p < 0.05 by Student's t-test). (D) Glial fibrillary-associated protein (GFAP) and ionized calcium-binding adapter molecule 1 (IBA-1) immunostaining in the spinal cord of WT and DKO mice. GFAP-positive astrocytes and IBA-1-positive microglial cells surrounding motor neurons (counterstained with Giemsa) of DKO mice showed swelling cell bodies and processes, a typical sign of astrogliosis. (E,F) Electron microscopic analysis of spinal roots showed that DKO mice at age P5 had severe damage of motor neuron axons in the ventral root (E) but not of sensory neuron axons in the dorsal root (F). Bar, 2 µm. (G,H) Damaged axons in the ventral root showed either vacuoles or loss of axoplasm (G), as an early sign of axonal damage. At a later stage of axonal damage, dark disintegrated myelin sheaths and amorphous lipid were present (H). Bar, 1 µm. (I) Percentage of injured axons in the ventral and dorsal roots of DKO and WT mice (*p < 0.05 by Student's t-test, n = 3). Data are mean ± s.e.m.
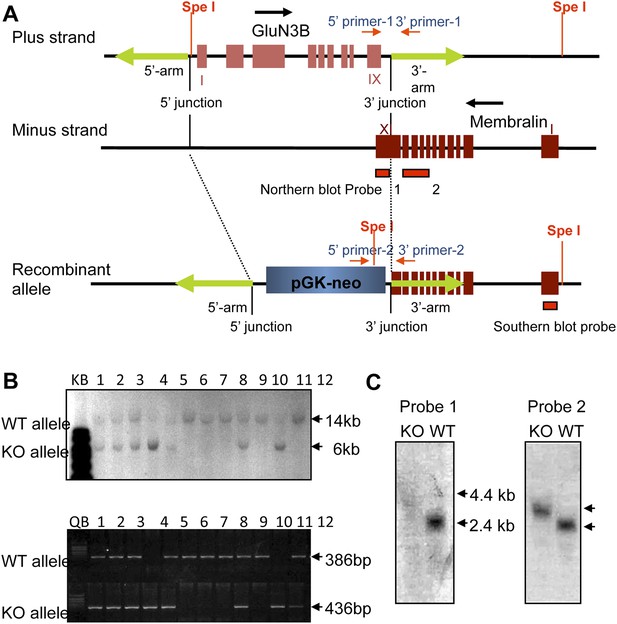
Generation of GluN3B/membralin DKO mice.
(A) GluN3B and membralin genes arranged in tail-to-tail fashion with overlapping 3′ coding regions of both genes. Blue and brown boxes indicate predicted exons of GluN3B and membralin genes, respectively. The entire GluN3B coding region and part of the 3′ coding region of membralin between the 5′- and 3′-arm (green arrows) in the WT allele were replaced by a Neo/PGK cassette in the DKO allele by homologous recombination. Spe I restriction sites, Southern blot and Northern blot probes, and primers used for PCR genotyping are indicated. (B) Genotyping by Southern blot analysis of SpeI-digested genomic DNA and by PCR. Mouse genomic DNA from 12 littermates from a single breeding pair of heterozygotes was subjected to Southern blot (top panel) and PCR analysis (bottom panel). Hybridizing bands or PCR fragments corresponding to WT and DKO allele are indicated. Both methods identified 4 WT (#6, 7, 8, and 10), 6 heterozygote (#1, 2, 3, 5, 9, and 12), and 2 homozygote (#4 and 11) mice. (C) Northern blot analysis of total RNA from null (KO) and WT mice using probes 1 and 2, derived from the last exon (XI) or exon VII-X of membralin, respectively (see A). Probe 1 generated a hybridization band (∼2.8 kb) in WT but not in DKO mice. Probe 2 generated a ∼2.8 kb band in WT and a ∼3.4. kb band in DKO mice.
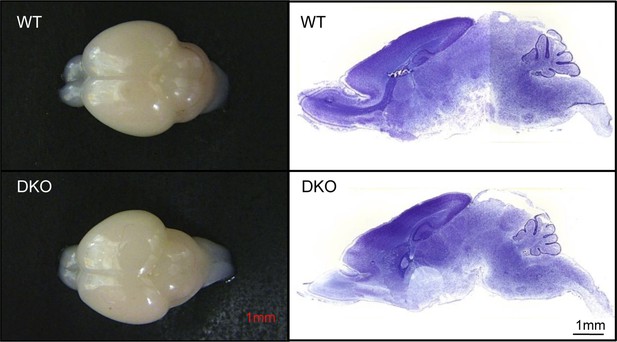
GluN3B/membralin DKO and WT mice show similar gross anatomy of the brain.
Left: whole brains dissected from DKO and WT mice at P5. Right: Nissl-stained sagittal brain sections from DKO and WT mice at P5.
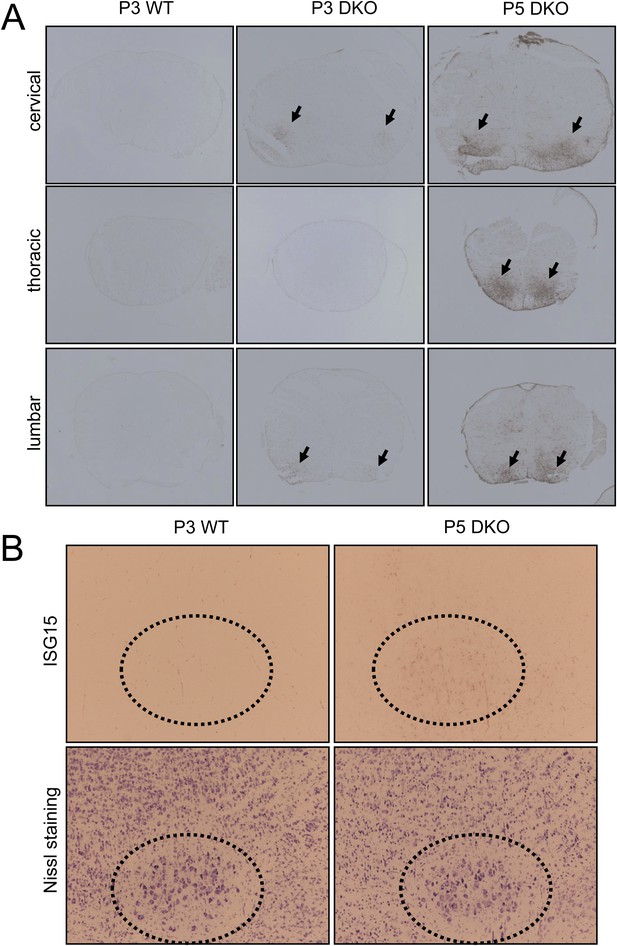
Expression of the motor neuron injury marker ISG15 in the spinal cord and brainstem of membralin KO mice.
(A) ISG15 immunosignal was barely detectable in spinal cord of P3 WT littermate mice but decorated the ventral grey matter (arrows) of cervical and lumbar (but not thoracic) spinal cord of membralin KO mice. By P5, stronger ISG15 immunostaining appeared in the ventral grey matter (arrows) at all levels of the spinal cord in KO mice. (B) Similarly, prominent ISG15 staining was observed at P5 in the facial nucleus (dotted circle) of KO but not WT littermate mice. Large motor neurons are indicated by Nissl staining of contiguous sections.
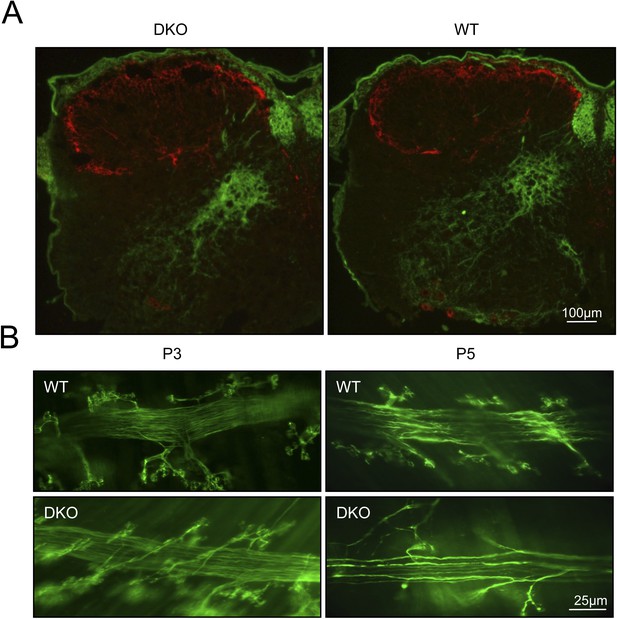
Degeneration of motor neuron fibers in phrenic nerve of GluN3B/membralin DKO mice.
(A) Primary afferent fibers, stained with anti-calcitonin gene-related peptide (CGRP, red), remained intact in DKOK compared to WT mice. Axons counterstained with anti-parvalbumin (Parv, green). (B) Axonal degeneration of phrenic motor neurons at P5 in DKO mice, as evidenced by paucity of fibers compared to WT.
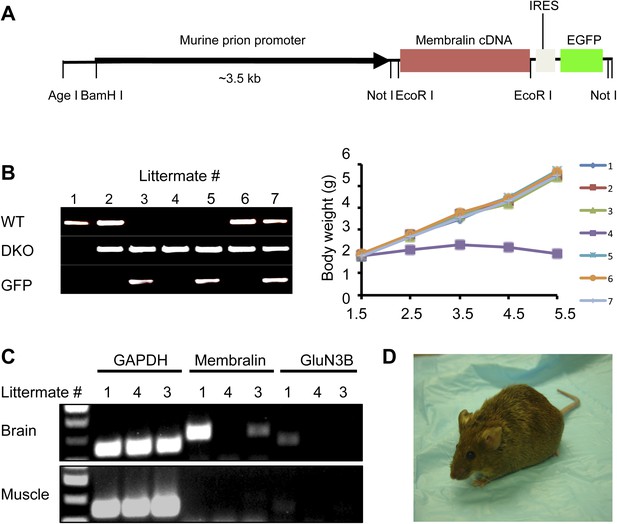
Membralin transgene [Tg (membralin)] rescues GluN3B/membralin (DKO) mice.
(A) Generation of the transgenic constructs expressing membralin. The murine prion promoter was cloned with full-length mouse membralin cDNA followed by the IRES and the EGFP sequence. The entire transgenic sequence was isolated by enzymatic digestion and used to generate Tg (membralin) mice. (B) DKO/Tg (membralin) mice were viable and fertile. Left: genotyping by PCR showed that 3 littermates (#3, 4 and 5) from a breeding of DKO and Tg (membralin) mice were positive to DKO primers and negative to WT primers (same primers used as in Figure 1). Of the three DKO mice, the two receiving membralin transgene (#3 and 5, as positively identified by GFP primers) were rescued. Right: Weight of the 7 littermates was monitored after birth. Only DKO mouse #4, which was negative for the membralin transgene, showed weight loss after P3 and died at P5.5. Data shown here are from one representative litter (5 independent litters were analyzed with similar results). (C) Gene expression analysis by RT-PCR for mice #1, 3, 4 of the same litter as in B. Membralin transgene expression is seen in the brains of littermates #1 and 3, but not 4, whereas GluN3B is only expressed in #1. Membralin and GluN3B are not expressed in muscle samples. GAPDH is expressed in both brain and muscles of all mice and served as a positive control. (D) Rescued DKO/Tg (membralin) mice lived to adulthood without any sign of paresis.
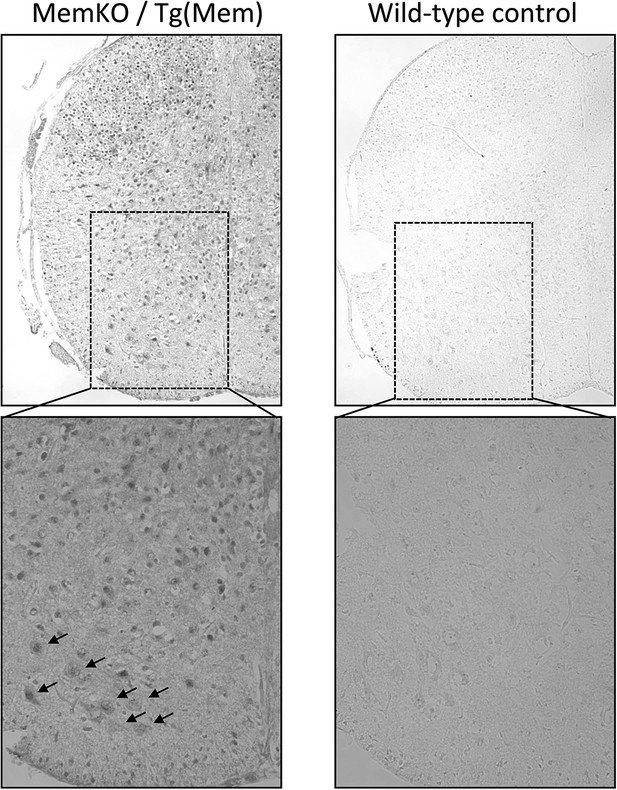
Expression of GFP-membralin transgene in spinal cord of membralin transgenic mice.
GFP immunostaining shows expression of membralin-GFP transgene in all spinal cord layers of membralin (MEM) KO and transgenic (Tg) mice (left), but not WT mice (right). GFP immunostaining was located predominantly in neurons, including motor neurons (arrows). Images at low (top) and high (bottom) magnification are shown from the lumbar spinal cord at P5.
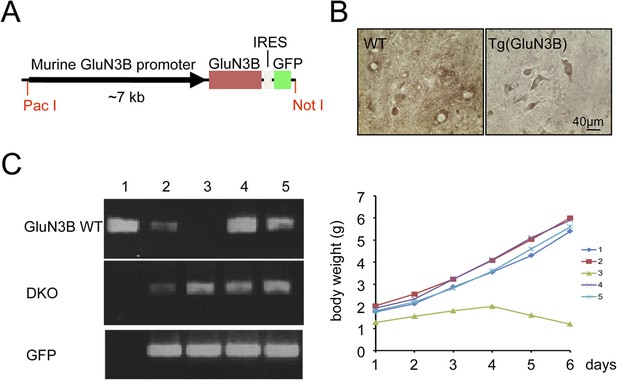
GluN3B transgene cannot rescue GluN3B/membralin DKO mice.
(A) DNA construct used for generating GluN3B transgenic [Tg (GluN3B)] mice containing the 7 kb murine GluN3B promoter followed by murine full-length coding region for GluN3B, IRES, and GFP. (B) Stronger immunocytochemical signal for GluN3B signal in motor neurons of lumbar spinal cord of Tg (GluN3B) mice compared to WT mice. (C) Tg (GluN3B) cannot rescue DKO mice. Left: PCR genotyping shows one mouse (#3) among five from a DKO x Tg (GluN3B) litter was positive for DKO and GFP primers, but negative for WT primers (same primers as used in Figure 1). Right: weight of the 5 littermates was monitored after birth, and DKO mouse #3, which contained the GluN3B transgene, lost weight, and died at P6.
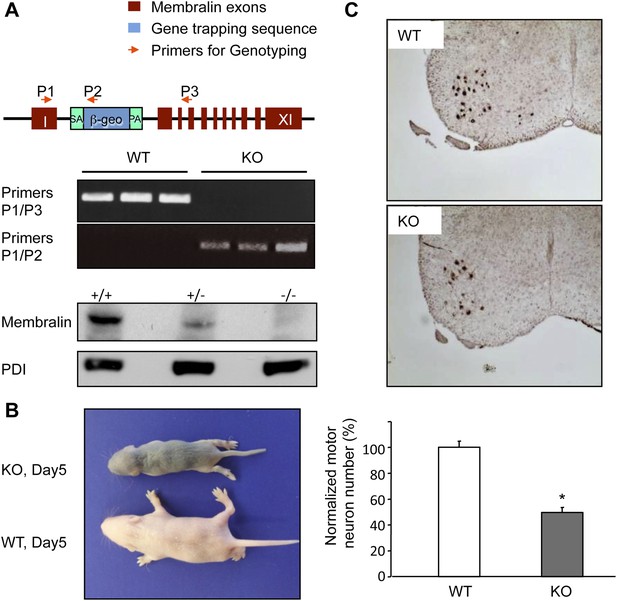
Membralin KO mice die of motor neuron degeneration and consequent paresis.
(A) Gene trapping was used to generate membralin KO mice by inserting a trapping vector that contained a splicing acceptor sequence between exon 1 and 2 to disrupt normal RNA splicing. Primers (P1, P2, P3, red arrows) were designed to detect normal and trapped membralin transcripts. RT-PCR experiments showed that PCR products using the P1 and P3 primer pair were only detected in WT mouse brain (lane 1), liver (lane 2), and kidney (lane 3), whereas PCR products using the P1 and P2 primers were only detected in these tissues of KO mice. (B) Membralin KO mice phenocopied GluN3B/membralin DKO mice and died of paresis around P5. (C) Lumbar motor neurons, identified by anti-Hb9 staining (top panels), were significantly reduced (lower panel) in membralin KO mice compared to WT mice (n = 3 for each group of mice, *p < 0.05, Student's t-test). Data are mean +s.e.m.
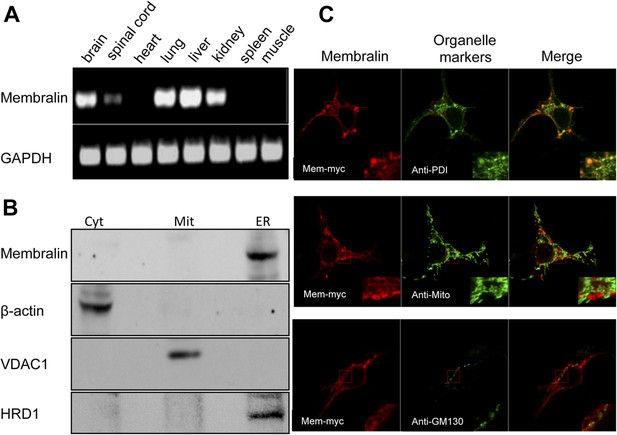
Cellular expression and localization of membralin.
(A) Semi-quantitative RT-PCR analysis showed the expression of membralin mRNA in brain (1), spinal cord (2), lung (4), liver (5), and kidney (6), but not in heart (3), spleen (7), and muscle (8) tissues of WT mice. (B) Western blot analysis showed the expression of membralin protein in the ER fraction, but not in the mitochondrial fraction (Mit) or the cytosolic fraction (Cyt), of the WT mouse brains. (C) Subcellular localization of Myc-tagged membralin in HEK 293 cells. Cells were transiently transfected with Myc-tagged membralin. At 48 hr after transfection, localization of membralin was detected by confocal microscopy (Zeiss 710). Organelles were labeled with specific markers (anti-PDI for ER, anti-mitochondria for mitochondria, and anti-GMP130 for Golgi). Region of interest shown at higher magnification in insets (bottom right corner). Merged pictures show membralin co-localized with the ER marker, PDI.
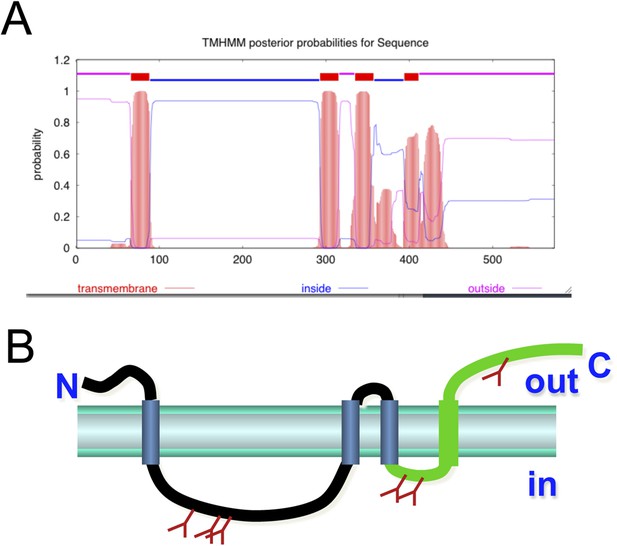
Structural prediction of membralin.
(A) Membralin's predicted structure has four transmembrane regions with termini on the same side of membrane. (B) The predicted glycosylation sites are also indicated by brown tree signs, which suggest the topology of the membralin on ER membrane (lumen and cytosolic side is indicated as ‘in’ and ‘out’, respectively).
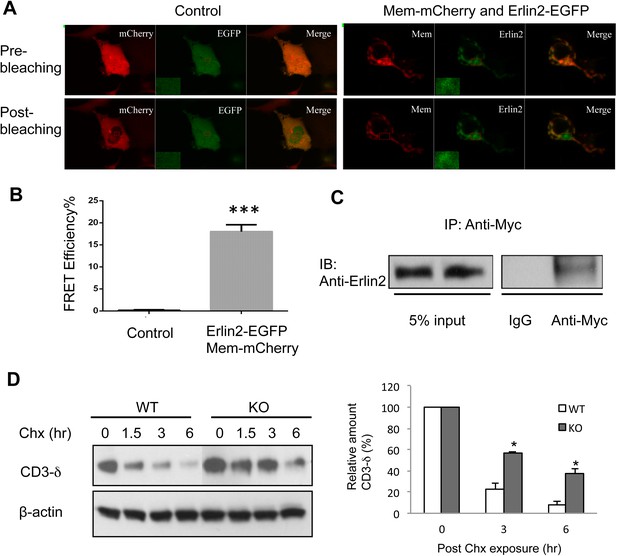
Membralin interacts with Erlin2 and regulates protein degradation.
(A) HEK 293T cells were transfected with control (mCherry and EGFP) or target (membralin-mCherry and Erlin2-EGFP) molecules. Individual or merged fluorescence images were shown in pre-bleaching and post-bleaching conditions. (B) FRET efficiency was quantified for control and target groups. All data shown are mean ± s.e.m. n = 10; ***p < 0.001 by Student's t-test. (C) The interaction of membralin with Erlin2 was confirmed by co-immunoprecipitation experiments using HA-tagged Erlin2 and Myc-tagged membralin fusion proteins co-transfected into HEK 293T cells. Whole-cell lysates were immunoprecipitated with anti-Myc or IgG (negative control) and immunoblotted using anti-Erlin2. (D) MEFs from both WT and membralin KO mouse were transfected with HA tagged CD3-δ and subjected to pulse-chase analysis of CD3-δ degradation after exposing with cycloheximide (Chx). Proteins collected at the indicated time points were subjected to immunoblotting with antibodies to HA. Image shown represents the example of immunoblotting and graphs, the quantification of three experiments. (n = 3, *p < 0.05 by Student's t-test). All data shown are mean ± s.e.m.
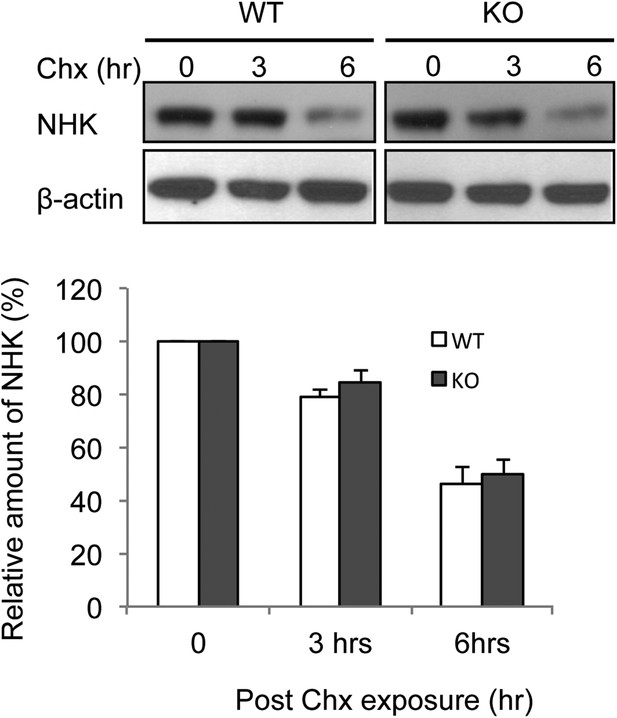
Deletion of membralin did not prolong the half life of ERAD substrate NHK.
MEFs from both WT and membralin KO mouse were transfected with HA tagged NHK and subjected to pulse-chase analysis of degradation after exposed to cycloheximide (Chx). Proteins collected at the indicated time points were subjected to immunoblotting with antibodies to HA. Image shown represent example of immunoblotting and graphs, the quantification of three experiments. All data shown are mean ± s.d.
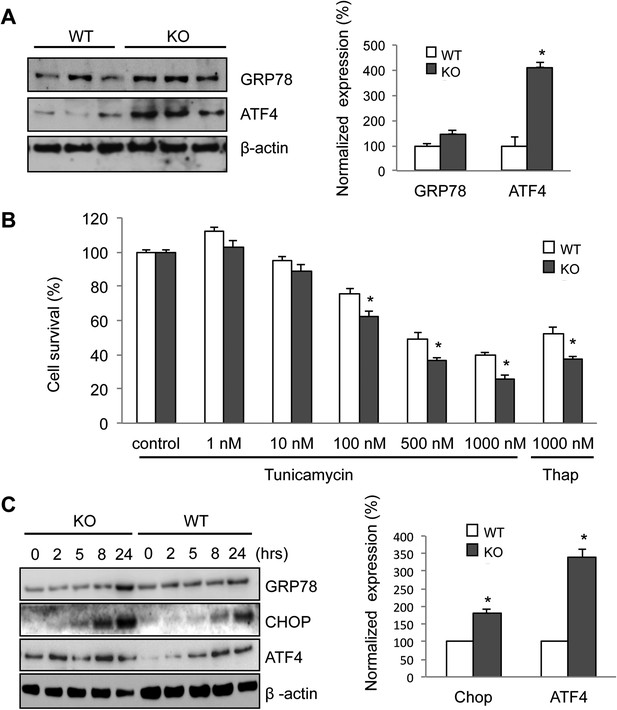
Elevated ER stress in membralin KO mice.
(A) Upregulation of GRP78 and ATF4 in spinal cord of membralin KO mice. Left: immunoblot analysis shows higher levels of GRP78 and ATF4 in the spinal cord of P3 membralin KO mice compared to that of littermate WT mice. Right: increased expression level of GRP78 and ATF4 normalized to actin in membralin KO mice over WT mice. (B) Survival of MEFs after 24-hr exposure to tunicamycin or thapsigargin determined by a cytotoxicity assay. (C) The expression level of GRP78, CHOP, and ATF4 in MEFs from KO and WT mice is shown after exposure to tunicamycin at different time points. Levels of CHOP and ATF4 are elevated earlier and higher in MEFs from KO than that from WT mice. A representative immunoblot is shown; graphs include data from three experiments. For each panel, data are mean ± s.e.m.; n = 3 for each bar; *p < 0.05 by Student's t-test.
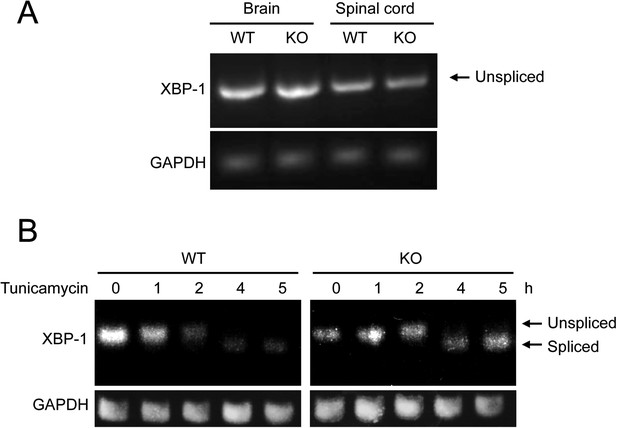
Membralin deletion does not alter Xbp-1 splicing.
(A) RT-PCR assay of Xbp-1 mRNA from brain and spinal cord from membralin WT and KO mouse. (B) RT-PCR assay of spliced and unspliced Xbp1 mRNA from membralin WT and KO MEF cells exposed to the ER stress inducer, tunicamycin (300 nM), for the indicated times.
Additional files
-
Supplementary file 1
Primer list for genotyping and sequencing.
- https://doi.org/10.7554/eLife.06500.018