Mass Spectrometry: Using proteomics to probe neurons
Deconstructing the immensely complex molecular basis of neuronal networks in the mammalian brain would help us to better understand how neurons develop and form connections (called synapses) with each other. Significant strides in the analysis of gene expression have been made in recent years, revealing the regulatory programs that govern the fate of individual neurons, their interactions with diverse ligands, and their ability to adapt to changes, such as environmental cues and injuries (Schreiner et al., 2014). However, measures of gene expression might not reflect the actual levels of proteins produced in cells, so efforts are being made to study the proteins directly.
Evidence suggests that changes in the abundance or activity of proteins in synapses may lead to defects in neurons that are implicated in neuropsychiatric disorders (Craft et al., 2013). Therefore, uncovering the repertoire of proteins produced by neurons could help us understand the underlying molecular basis of such conditions. The large-scale study of the proteins found in cells—known as proteomics—poses significant challenges to researchers. For example, a single gene in a mammalian cell can be used as a template to make many different forms (or ‘isoforms’) of a protein through a process called alternative splicing. These isoforms can be very similar to each other, but they can play very different roles in cells, so it is important for proteomics techniques to be able to distinguish them. The situation is further complicated by the presence of large protein families encoded by very similar genes.
Mass spectrometry is the leading approach for proteomics investigations, and encompasses both global analyses of all the proteins found in cells, and ‘targeted’ approaches that accurately measure the abundance of particular proteins. Selected reaction monitoring (SRM) mass spectrometry is the most widely used technique for targeted proteomics (Elschenbroich and Kislinger, 2011). Proteins are extracted from cells and digested by enzymes to produce millions of fragments (called peptides). However, only a small fraction of these peptides will be unique to the protein or isoform of interest. SRM mass spectrometry is able to accurately determine protein abundance because it can be used to measure just those peptides we are interested in.
The absolute quantification of proteins by SRM involves ‘spiking’ the samples with known concentrations of labelled synthetic peptides after the enzyme treatment (Barr et al., 1996). However, variations in the efficiency of the enzymes can lead to errors with this approach. Alternative approaches use known concentrations of labelled whole synthetic proteins (Brun et al., 2007; Stergachis et al., 2011), which are added to the sample before the enzyme treatment.
However, the use of SRM mass spectrometry to quantify the isoforms of protein families from complex tissues had not been explored. Now, in eLife, Peter Scheiffele and colleagues at the University of Basel—including Dietmar Schreiner and Jovan Simicevic as joint first authors—have developed SRM assays to quantify the isoforms of the neurexin family of proteins in the mouse brain (Schreiner, Simicevic et al. 2015).
Neurexins are cell adhesion proteins that play important roles in the formation and differentiation of synapses (Zhang et al., 2010). All three of the genes that encode neurexin proteins contain various segments that can be removed from messenger RNA in different combinations by alternative splicing (Chih et al., 2006). The modified messenger RNA molecules are then translated to make the different neurexin protein isoforms. The segments have been shown to regulate the interactions between ligands and their receptors on the surface of neurons and to alter the activity of synapses in a variety of ways (Aoto et al., 2013).
Schreiner, Simicevic et al. made protein standards that contained different neurexin isoforms fused to the fluorescent protein GFP before carrying out SRM assays (Figure 1). They found that, in several different regions of the brain, neurexin isoforms that contain the segments known as AS3 and AS4 were regulated in the same way, but isoforms that contain another segment called AS6 were regulated independently.
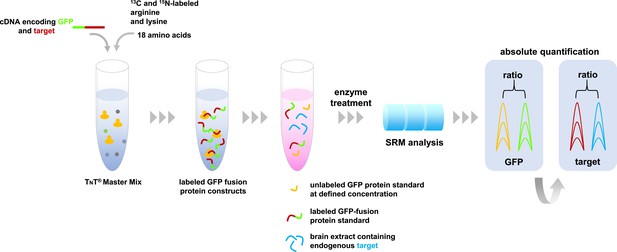
Absolute quantification of proteins by SRM mass spectrometry.
A master mix containing the components required for protein synthesis, the amino acids arginine and lysine labeled with carbon-13 and nitrogen-15, and all other unlabeled amino acids are mixed with cDNA molecules that encode the proteins of interest fused with green fluorescent protein (GFP). These fusion proteins (indicated in green-red) are combined with a sample containing the target proteins of interest (depicted in blue), and a known concentration of unlabeled GFP as an internal standard (shown in yellow). This protein mixture is digested by enzymes and the resulting peptides are analyzed by SRM mass spectrometry that specifically measures peptides that are unique to the target proteins. The resulting SRM traces contain information about the abundance of these peptides. The known spiked concentration of the unlabeled GFP standard (yellow) is used to determine the absolute amounts of the labeled GFP fusion protein standard (the green part of the fusion protein). In turn, the target protein component of the GFP fusion protein standard (the red section) is used to determine the absolute amounts of the target proteins in the biological sample (shown in blue).
The absolute quantification of the neurexins revealed that two of the isoforms (called alpha and beta) differed in abundance and that, overall, neurexins are present at relatively high levels across the whole mouse brain. The ability of neurexin isoforms to interact with ligands at synapses was associated with specific segments, which demonstrates that alternative splicing can modulate neurexin activity.
The SRM assays developed by Schreiner, Simicevic et al. enabled them to discriminate between neurexin isoforms that only differed in a single amino acid residue. Their simple approach can be applied to other protein families in any accessible tissue, and hence may be applied to a range of biological questions.
References
-
Isotope dilution–mass spectrometric quantification of specific proteins: model application with apolipoprotein A-IClinical Chemistry 42:1676–1682.
-
Isotope-labeled protein standards: toward absolute quantitative proteomicsMolecular & Cellular Proteomics 6:2139–2149.https://doi.org/10.1074/mcp.M700163-MCP200
-
Rapid empirical discovery of optimal peptides for targeted proteomicsNature Methods 8:1041–1043.https://doi.org/10.1038/nmeth.1770
Article and author information
Author details
Publication history
Copyright
© 2015, Kim and Kislinger
This article is distributed under the terms of the Creative Commons Attribution License, which permits unrestricted use and redistribution provided that the original author and source are credited.
Metrics
-
- 1,386
- views
-
- 171
- downloads
-
- 0
- citations
Views, downloads and citations are aggregated across all versions of this paper published by eLife.
Download links
Downloads (link to download the article as PDF)
Open citations (links to open the citations from this article in various online reference manager services)
Cite this article (links to download the citations from this article in formats compatible with various reference manager tools)
Further reading
-
- Cell Biology
Distal appendages are nine-fold symmetric blade-like structures attached to the distal end of the mother centriole. These structures are critical for formation of the primary cilium, by regulating at least four critical steps: ciliary vesicle recruitment, recruitment and initiation of intraflagellar transport (IFT), and removal of CP110. While specific proteins that localize to the distal appendages have been identified, how exactly each protein functions to achieve the multiple roles of the distal appendages is poorly understood. Here we comprehensively analyze known and newly discovered distal appendage proteins (CEP83, SCLT1, CEP164, TTBK2, FBF1, CEP89, KIZ, ANKRD26, PIDD1, LRRC45, NCS1, CEP15) for their precise localization, order of recruitment, and their roles in each step of cilia formation. Using CRISPR-Cas9 knockouts, we show that the order of the recruitment of the distal appendage proteins is highly interconnected and a more complex hierarchy. Our analysis highlights two protein modules, CEP83-SCLT1 and CEP164-TTBK2, as critical for structural assembly of distal appendages. Functional assays revealed that CEP89 selectively functions in RAB34+ ciliary vesicle recruitment, while deletion of the integral components, CEP83-SCLT1-CEP164-TTBK2, severely compromised all four steps of cilium formation. Collectively, our analyses provide a more comprehensive view of the organization and the function of the distal appendage, paving the way for molecular understanding of ciliary assembly.
-
- Cell Biology
The primary cilium is a microtubule-based organelle that cycles through assembly and disassembly. In many cell types, formation of the cilium is initiated by recruitment of ciliary vesicles to the distal appendage of the mother centriole. However, the distal appendage mechanism that directly captures ciliary vesicles is yet to be identified. In an accompanying paper, we show that the distal appendage protein, CEP89, is important for the ciliary vesicle recruitment, but not for other steps of cilium formation (Tomoharu Kanie, Love, Fisher, Gustavsson, & Jackson, 2023). The lack of a membrane binding motif in CEP89 suggests that it may indirectly recruit ciliary vesicles via another binding partner. Here, we identify Neuronal Calcium Sensor-1 (NCS1) as a stoichiometric interactor of CEP89. NCS1 localizes to the position between CEP89 and a ciliary vesicle marker, RAB34, at the distal appendage. This localization was completely abolished in CEP89 knockouts, suggesting that CEP89 recruits NCS1 to the distal appendage. Similarly to CEP89 knockouts, ciliary vesicle recruitment as well as subsequent cilium formation was perturbed in NCS1 knockout cells. The ability of NCS1 to recruit the ciliary vesicle is dependent on its myristoylation motif and NCS1 knockout cells expressing a myristoylation defective mutant failed to rescue the vesicle recruitment defect despite localizing properly to the centriole. In sum, our analysis reveals the first known mechanism for how the distal appendage recruits the ciliary vesicles.