Sensory Neurons: Addressing burning questions on axon regeneration
In the skin, an extensive network of sensory axons that extend from cell bodies in the spine to allow us to perceive touch, temperature, pain and other stimuli – a process essential for safely navigating our environment. When these axons are damaged through skin injuries, such as cuts or burns, regenerative mechanisms are triggered to restore lost sensations and tissue function.
This process starts with Wallerian degeneration, named after the 19th-century neurophysiologist Augustus Volney Waller (Waller and Owen, 1850). Axon segments that have been separated from their cell bodies experience a rapid influx of calcium, which contributes to the disintegration of the axonal membrane and the myelin sheath that protects it (Geden and Deshmukh, 2016). Immune cells are then recruited to the site to dispose of the damaged cell fragments, clearing the way for new axons to regrow under the influence of various pro-regenerative pathways (Mahar and Cavalli, 2018). The immediate environment surrounding the damaged cell – in particular certain biochemical signals from neighboring skin and nerve cells – plays an important role throughout this process (Avraham et al., 2021). For instance, the reactive oxygen species (ROS) formed after epithelial damage can impact axonal regrowth, either promoting or hindering this process depending on the context (Rieger and Sagasti, 2011; Cobb and Cole, 2015).
Sensory axons have the potential to fully recover after a mechanical injury, such as a cut. However, burn injuries present significant challenges to both skin and neuronal healing, with sensory axons often not fully recovering after this type of wound (Tirado-Esteban et al., 2020). Despite decades of research into the regenerative capacity of sensory and peripheral neurons, a full understanding of this process – and why it is impaired during burn recovery – has remained elusive. Now, in eLife, Anna Huttenlocher and colleagues at the University of Wisconsin-Madison – including Alexandra Fister as first author – report new insights into how the local microenvironment of burnt tissue can influence axon regeneration (Figure 1; Fister et al., 2024).
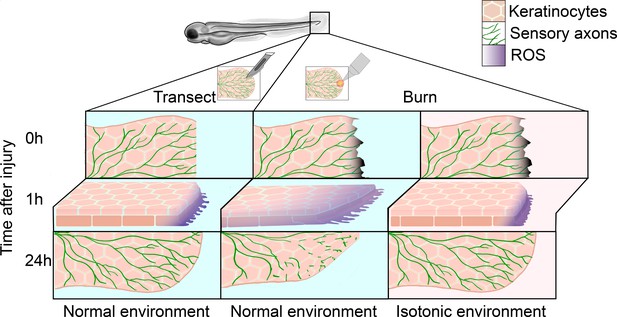
Regeneration of sensory neurons following a tail transection or burn.
In normal freshwater medium (blue), transecting the tail fin of a zebrafish larva (left) damages keratinocytes (pink) and sensory axons (green), leading to the production of reactive oxygen species (ROS, purple) at the site of injury within the first hour. After 24hours, sensory axons and keratinocytes have regenerated. In the same conditions (center), burning the tail fin increases the migration of keratinocytes and results in ROS production extending further from the wound edge. There is also impaired sensory axon regeneration and wound healing within the first 24hours. Administering a burn injury in isotonic media (pink, right) rescues both the enhanced keratinocyte migration and the delayed axon regeneration observed in the normal environment.
Capitalizing on a burn wound assay they had previously established in zebrafish larvae, the team first compared how well sensory neurons regrow in these animals after a part of their tails was either burned or amputated (LeBert et al., 2018). This showed that axon healing was delayed by 72hours following a burn. The ability of the wounded area to recover touch sensitivity after a burn was also impaired, which correlated with morphological alterations in the axonal network. In line with previous findings, increased levels of calcium were also found in damaged and fragmented axons after a burn injury.
Elegant experiments showed that excising burnt tissue 5–15minutes after a burn injury led to healing proceeding at a normal pace. Performing the same manipulation six hours after the burn, however, did not accelerate the regeneration process, suggesting that the microenvironment surrounding the damaged tissue contributes to delayed healing.
After a cut, the cells of the deep skin layer (known as basal keratinocytes) collectively migrate between the relatively stationary superficial skin cells and the mesenchymal cells of the connective tissue to facilitate wound closure (Gault et al., 2014). Sensory axons run between the external keratinocyte layer and are also enveloped within the basal layer (O’Brien et al., 2012). To evaluate the impact of the environment on sensory axons, Fister et al. tracked the movement of axons and skin cells using high-resolution live imaging in a series of transgenic zebrafish lines. After a burn, basal cells migrated in an enhanced and uncoordinated manner, dragging keratinocytes in the superficial layers toward the wound and displacing sensory axons from their cell bodies. This early movement was shown to begin at the onset of axonal damage, leading Fister et al. to investigate whether the two events are causally linked.
Two separate methods were used to examine the impact of blocking this migration after a burn. Chemically inhibiting a protein complex required for cell migration slowed early keratinocyte migration but did not change axon regeneration. However, replacing the hypotonic freshwater in which zebrafish normally live with an isotonic saline solution while administering a burn facilitated axon regeneration and rapid recovery of tail fin touch sensitivity. Indeed, previous reports on mechanical injuries had shown that initial wound closure and basal cell migration are triggered by exposure to hypotonic freshwater, which, unlike isotonic saline, encourages water to enter cells, resulting in osmotic cell swelling (Gault et al., 2014). However, improvements in regeneration and sensitivity were not observed if the larvae were only exposed to isotonic saline one hour after the burn injury, supporting the idea that the local mechanisms influencing axon regeneration take place shortly after burning occurs.
To identify the environmental signals involved, Fister et al. next tracked ROS production in recovering wounds. Compared to cuts, increased ROS signals were detected in burns further from the wound margin. Preventing basal cell migration either chemically or via exposure to isotonic saline blocked enhanced ROS production deep inside the burn injury, but not at its edges. Taken together, this suggests that the enhanced keratinocyte migration observed after a burn disrupts how and where ROS are produced during early recovery. Blocking production of ROS either chemically or genetically proved fatal for the larvae, and therefore further experiments are required to establish whether ROS signals directly delay axon regeneration in burns.
The findings of Fister et al. identify novel links between the local microenvironment, ROS production and the regenerative capacity of peripheral sensory axons in response to burn wounds. Overall, the experiments indicate that burns lead to enhanced and uncoordinated basal keratinocyte migration that displaces sensory axons, which is associated with the start of axonal damage. At the same time, it remains unclear whether exposing the burn to isotonic conditions rescues axon regeneration by blocking keratinocyte migration, or through further mechanisms. Notably, exposing wounded or burnt tissue to the normal hypotonic environment of zebrafish leads to osmotic cell swelling, which is completely blocked under isotonic conditions. Investigating whether any signaling pathways and cellular responses related to swelling contribute to the beneficial effects of isotonic saline could be worthwhile in future studies.
References
-
Oxidative and nitrative stress in neurodegenerationNeurobiology of Disease 84:4–21.https://doi.org/10.1016/j.nbd.2015.04.020
-
Osmotic surveillance mediates rapid wound closure through nucleotide releaseThe Journal of Cell Biology 207:767–782.https://doi.org/10.1083/jcb.201408049
-
Axon degeneration: context defines distinct pathwaysCurrent Opinion in Neurobiology 39:108–115.https://doi.org/10.1016/j.conb.2016.05.002
-
Intrinsic mechanisms of neuronal axon regenerationNature Reviews Neuroscience 19:323–337.https://doi.org/10.1038/s41583-018-0001-8
-
Coordinate development of skin cells and cutaneous sensory axons in zebrafishThe Journal of Comparative Neurology 520:816–831.https://doi.org/10.1002/cne.22791
-
Experiments on the section of the glossopharyngeal and hypoglossal nerves of the frog, and observations of the alterations produced thereby in the structure of their primitive fibresPhilosophical Transactions of the Royal Society of London 140:423–429.https://doi.org/10.1098/rstl.1850.0021
Article and author information
Author details
Publication history
Copyright
© 2024, Kaszás and Enyedi
This article is distributed under the terms of the Creative Commons Attribution License, which permits unrestricted use and redistribution provided that the original author and source are credited.
Metrics
-
- 403
- views
-
- 44
- downloads
-
- 0
- citations
Views, downloads and citations are aggregated across all versions of this paper published by eLife.
Download links
Downloads (link to download the article as PDF)
Open citations (links to open the citations from this article in various online reference manager services)
Cite this article (links to download the citations from this article in formats compatible with various reference manager tools)
Further reading
-
- Cell Biology
Proliferating animal cells maintain a stable size distribution over generations despite fluctuations in cell growth and division size. Previously, we showed that cell size control involves both cell size checkpoints, which delay cell cycle progression in small cells, and size-dependent regulation of mass accumulation rates (Ginzberg et al., 2018). While we previously identified the p38 MAPK pathway as a key regulator of the mammalian cell size checkpoint (S. Liu et al., 2018), the mechanism of size-dependent growth rate regulation has remained elusive. Here, we quantified global rates of protein synthesis and degradation in cells of varying sizes, both under unperturbed conditions and in response to perturbations that trigger size-dependent compensatory growth slowdown. We found that protein synthesis rates scale proportionally with cell size across cell cycle stages and experimental conditions. In contrast, oversized cells that undergo compensatory growth slowdown exhibit a superlinear increase in proteasome-mediated protein degradation, with accelerated protein turnover per unit mass, suggesting activation of the proteasomal degradation pathway. Both nascent and long-lived proteins contribute to the elevated protein degradation during compensatory growth slowdown, with long-lived proteins playing a crucial role at the G1/S transition. Notably, large G1/S cells exhibit particularly high efficiency in protein degradation, surpassing that of similarly sized or larger cells in S and G2, coinciding with the timing of the most stringent size control in animal cells. These results collectively suggest that oversized cells reduce their growth efficiency by activating global proteasome-mediated protein degradation to promote cell size homeostasis.
-
- Cell Biology
- Physics of Living Systems
The regulation of cell physiology depends largely upon interactions of functionally distinct proteins and cellular components. These interactions may be transient or long-lived, but often affect protein motion. Measurement of protein dynamics within a cellular environment, particularly while perturbing protein function with small molecules, may enable dissection of key interactions and facilitate drug discovery; however, current approaches are limited by throughput with respect to data acquisition and analysis. As a result, studies using super-resolution imaging are typically drawing conclusions from tens of cells and a few experimental conditions tested. We addressed these limitations by developing a high-throughput single-molecule tracking (htSMT) platform for pharmacologic dissection of protein dynamics in living cells at an unprecedented scale (capable of imaging >106 cells/day and screening >104 compounds). We applied htSMT to measure the cellular dynamics of fluorescently tagged estrogen receptor (ER) and screened a diverse library to identify small molecules that perturbed ER function in real time. With this one experimental modality, we determined the potency, pathway selectivity, target engagement, and mechanism of action for identified hits. Kinetic htSMT experiments were capable of distinguishing between on-target and on-pathway modulators of ER signaling. Integrated pathway analysis recapitulated the network of known ER interaction partners and suggested potentially novel, kinase-mediated regulatory mechanisms. The sensitivity of htSMT revealed a new correlation between ER dynamics and the ability of ER antagonists to suppress cancer cell growth. Therefore, measuring protein motion at scale is a powerful method to investigate dynamic interactions among proteins and may facilitate the identification and characterization of novel therapeutics.