Retrograde fibroblast growth factor 22 (FGF22) signaling regulates insulin-like growth factor 2 (IGF2) expression for activity-dependent synapse stabilization in the mammalian brain
Figures
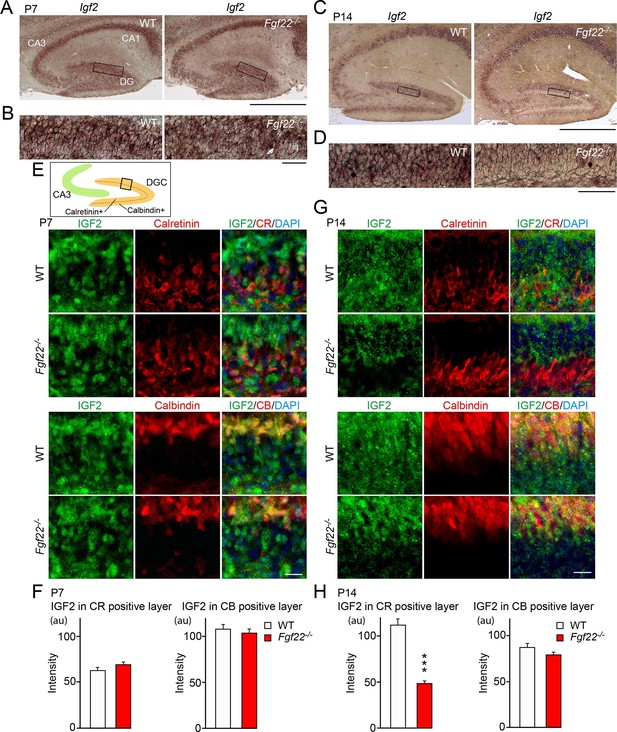
IGF2 expression is decreased in young DGCs in Fgf22-/- mice during the stage of synapse stabilization.
(A–D) In situ hybridization for Igf2 mRNA. (A) At P7 (initial stage of synaptic differentiation), Igf2 mRNA is similarly expressed in the hippocampus of WT and Fgf22-/- mice. Higher magnification views of the boxed areas are shown in (B). (C) At P14 (around the time of synaptic stabilization), Igf2 mRNA is decreased in Fgf22-/- mice in the inner molecular layer of DGCs. Higher magnification views of the boxed areas are shown in (D). Observations are from 3–5 animals per age and strain. (E–H) P7 and P14 hippocampal sections from WT and Fgf22-/- mice were immunostained for IGF2 and for either calretinin (CR; young DGCs) or calbindin (CB; mature DGCs). The illustration shows the pictured area (boxed). (E) IGF2 expression in DGCs at P7. Quantification of IGF2 immunoreactivity in CR and CB layers is shown in (F). There is no significant difference in the IGF2 intensity in either layer of DGCs at P7. (G) IGF2 expression in DGCs at P14. Quantification of IGF2 intensity in CR and CB layers is shown in (H). In P14 Fgf22-/- mice, IGF2 is significantly decreased in the CR-positive layer, but not in CB-positive layer of DGCs. Error bars are s.e.m. Data are from 77–160 fields from 3–5 animals. Significant difference from control at ***p<0.0001 by Student's t-test. Scale bars, (A and C) 500 μm, (B and D) 50 μm, (E and G) 20 μm.
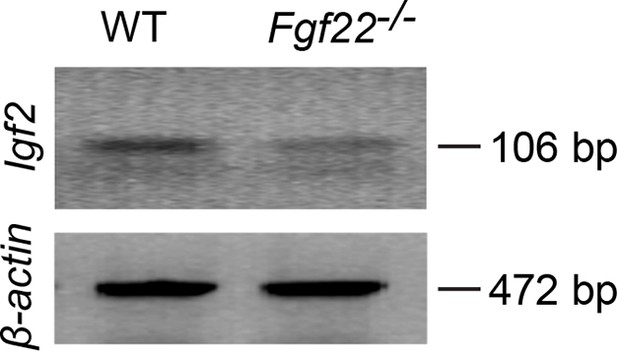
RT-PCR showing decreased Igf2 mRNA expression in DGCs of P14 Fgf22-/- mice.
Representative picture of RT-PCR detecting Igf2 mRNA. β-actin is used as a control. Total mRNA was extracted from DGCs of P14 WT and Fgf22-/- mice. The following primers were used for RT-PCR: IGF2-forward: 5’-TCTCATCTCTTTGGCCTTCGCCTT-3’, IGF2-reverse: 5’-GTCCGAACAGACAAACTGAAGCGT-3’ (amplifying 106 bp); β-actin forward: 5’-GTGGGCCGCTCTAGGCACCAA-3’, β-actin reverse: 5’-CTCTTTGATGTCACGCACGATTTC-3’ (amplifying 472 bp). Expression of Igf2 mRNA is decreased in Fgf22-/- mice compared to that in WT mice.
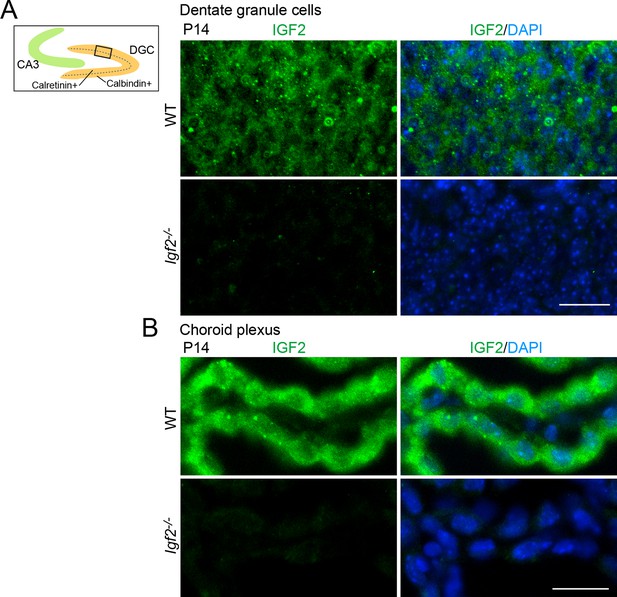
Validation of the anti-IGF2 antibody.
The anti-IGF2 antibody (Santa Cruz, sc-5622) was validated by immunostaining of brain sections prepared from P14 WT and Igf2-/- mice. (A) Representative images of IGF2 immunostaining in dentate granule cells (DGCs). The illustration shows the pictured area (boxed). IGF2, green; DAPI, blue. (B) Representative images of IGF2 immunostaining in the choroid plexus, which highly expresses IGF2. The anti-IGF2 antibody did not stain DGCs or the choroid plexus of Igf2-/- mice. Observations are from 3 animals per strain. Scale bars are 20 μm.
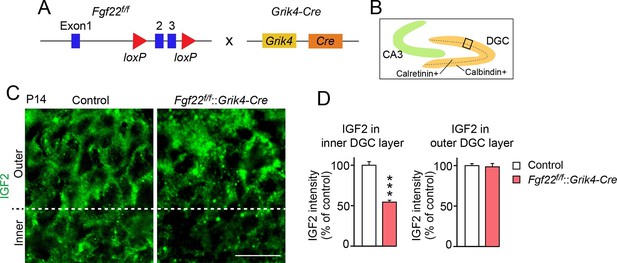
IGF2 expression in young DGCs is decreased in CA3-selective Fgf22-knockout mice.
(A) Schematic of CA3-selective Fgf22 deletion: Fgf22flox/flox(Fgf22f/f) mice were crossed with mice carrying Grik4-promoter-driven Cre (Grik4-Cre). (B–D) IGF2 staining in the DGCs of P14 Fgf22f/f::Grik4-Cre mice and control littermates (WT and Fgf22f/f mice; we did not observe any significant differences in IGF2 staining between WT and Fgf22f/f mice). (B) Illustration showing the pictured area (boxed). (C) Representative pictures of IGF2 immunostaining in DGCs. The dashed line indicates the border between the inner and outer layers of DGCs. (D) Quantification of IGF2 immunoreactivity in the inner and outer DGC layers. CA3-selective inactivation of FGF22 results in a significant decrease in the IGF2 expression in the inner DGC layer, but not in the outer DGC layer. Error bars are s.e.m. Data are from 20–25 fields (D, inner DGC layer) and from 40–50 fields (D, outer DGC layer) from 4–5 animals. Significant difference from control at ***p<0.0001 by Student's t-test. Scale bar, 20 μm.
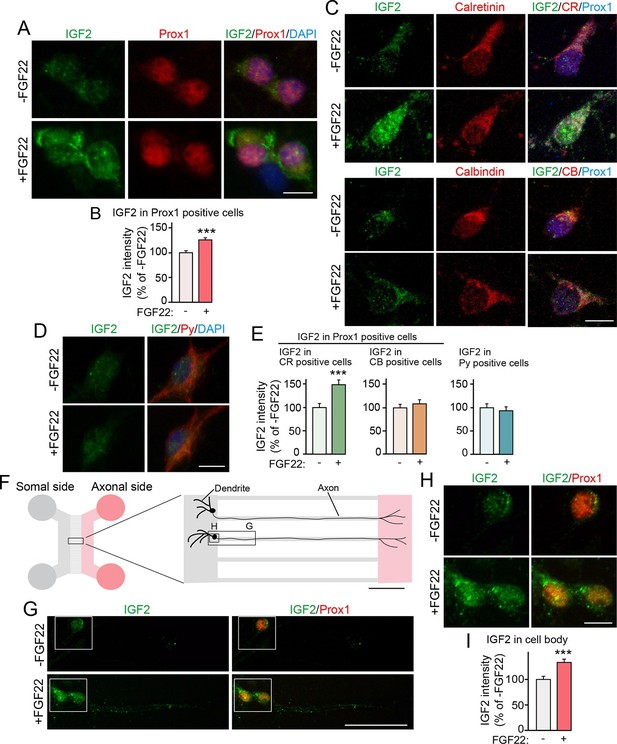
Bath and axonal application of FGF22 increases IGF2 expression in young DGCs.
(A–E) Cultured hippocampal neurons were treated with FGF22 at 1DIV, and fixed and stained at 7DIV. (A) Bath application of FGF22 increases IGF2 expression in DGCs (Prox1-positive). (B) Quantification of IGF2 immunoreactivity in the cell bodies of DGCs, normalized to untreated condition. (C) Bath application of FGF22 increases IGF2 expression in CR-positive DGCs, but not in CB-positive DGCs. (D) Bath application of FGF22 does not affect IGF2 expression in CA3 pyramidal neurons (Py-positive). (E) Quantification of IGF2 immunoreactivity in the cell bodies of CR- or CB-positive DGCs and CA3 pyramidal neurons. Data are normalized to the intensity from untreated cells. (F–I) Hippocampal neurons were plated onto the somal compartment of microfluidic chambers and cultured. FGF22 was applied into the axonal compartment at 2DIV, and cells were fixed and stained at 8DIV. (F) Schematic illustration of the microfluidic chamber. Representative pictures in (G) and (H) are taken from the boxed areas. (G–I) Axonal treatment of FGF22 increases IGF2 in the cell body of DGCs. (G) Lower magnification views of Prox1 positive DGCs. (H) Higher magnification views from the boxed areas in (G). Quantification of IGF2 immunoreactivity in the cell bodies of DGCs is shown in (I). Error bars are s.e.m. Data are from (B) 292–260 cells from 3 independent experiments, (E) 25–45 cells from 4 to 5 independent experiments, and (I) 67–78 cells from 3–4 independent experiments. Significant difference from control at ***p<0.0001 by Student's t-test. Scale bars, (A, C, D and H) 10 μm, (F) 100 μm, (G) 50 μm.
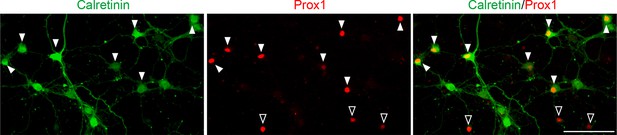
A majority of DGCs in culture are calretinin-positive.
Representative images of cultured hippocampal neurons stained for calretinin and Prox1. Cultured hippocampal neurons were fixed and stained at 7DIV. A majority of DGCs (Prox1-positive) in culture are calretinin-positive (63.65 ± 1.40%). Filled and empty arrowheads indicate calretinin-positive and negative DGCs, respectively. Data are from 9 fields from 3 independent experiments. Scale bar is 100 μm.
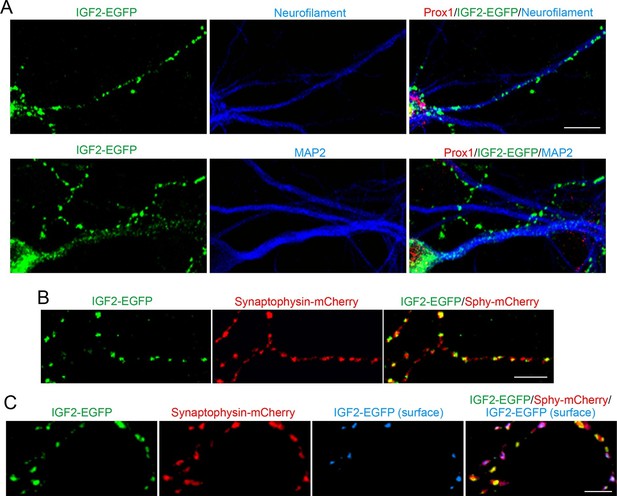
IGF2 localizes to presynaptic terminals of DGCs.
Cultured hippocampal neurons were transfected with the IGF2-EGFP plasmid at 3DIV, and fixed and stained at 10DIV. (A) IGF2-EGFP showed a punctate pattern of localization in neurofilament positive axons, while a diffuse pattern in MAP2 positive dendrites of DGCs. Observations were from at least 10 transfected Prox1 positive DGCs from 2 independent experiments. (B) The IGF2-EGFP plasmid was co-transfected with synaptophysin-mCherry (Sphy-mCherry) plasmid. Most of IGF2-EGFP puncta (83.1 ± 1.0% of total IGF2-EGFP puncta; data are from 53 cells from 3 independent experiments) co-localizes with synaptophysin-mCherry, a presynaptic terminal marker. (C) ~40% of IGF2-EGFP is localized on the surface of presynaptic terminals. At 10DIV, cells were stained with the anti-GFP antibody without a detergent, followed by Alexa Fluor 647 (shown in blue in the images). Surface IGF2-EGFP is always colocalized with synaptophysin-mCherry. Data are from 15 cells from 3 independent experiments. Scale bars, 10 μm.
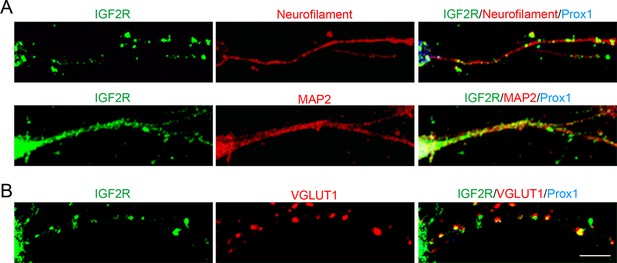
IGF2R, the major receptor for IGF2, is localized at presynaptic terminals of DGCs.
Cultured hippocampal neurons were fixed and stained at 10DIV. (A) IGF2R showed a punctate pattern in axons (neurofilament positive) and a diffuse pattern in dendrites (MAP2-positive) of DGCs (Prox1-positive). (B) Most of IGF2R puncta in DGC axons were colocalized with VGLUT1 puncta (84.32 ± 0.90%). Observation and data are from 8–12 cells from 2 independent experiments. Scale bar is 10 μm.
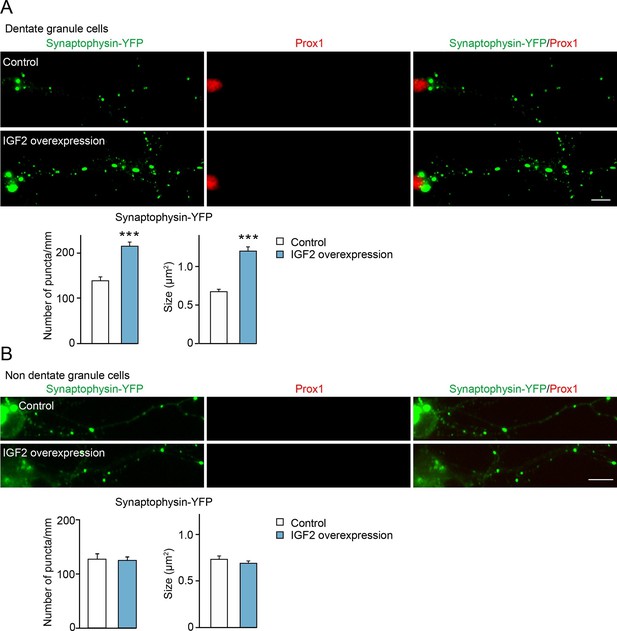
Overexpression of IGF2 in DGCs promotes their presynaptic development.
Cultured hippocampal neurons were transfected with the plasmid expressing IGF2 together with the synaptophysin-YFP plasmid at 3DIV, and fixed and stained at 10DIV. (A) Clustering of synaptophysin-YFP is increased in IGF2-overexpressed DGCs (Prox1-positive) compared to control DGCs. The graphs show quantification of the number and size of synaptophysin-YFP puncta in control and IGF2-overexpressed DGCs. (B) Overexpression of IGF2 does not alter clustering of synaptophysin-YFP in Prox1-negative hippocampal neurons. The graph shows quantification of the number and size of synaptophysin-YFP puncta in Prox1 negative neurons with or without IGF2 overexpression. Error bars are s.e.m. Data are from (A) 36–44 cells from 5–7 independent experiments, (B) 11–13 cells from 2–3 independent experiments. Significant difference from control at ***p<0.0001 by Student's t-test. Scale bars, 10 μm.
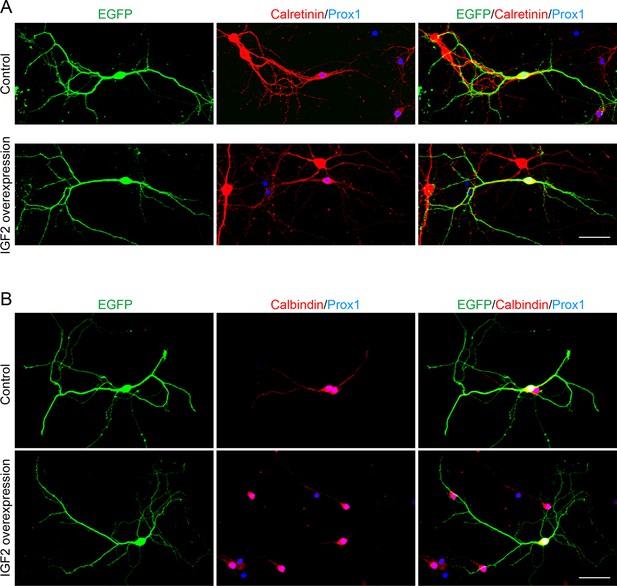
Overexpression of IGF2 does not appear to alter the morphology of DGCs.
Cultured hippocampal neurons were transfected with the IGF2 expression plasmid and EGFP-N1 plasmid at 3DIV, and fixed and stained at 10DIV. (A) Morphology of calretinin and Prox1 double-positive young DGCs, with or without IGF2 overexpression. (B) Morphology of calbindin- and Prox1-double positive mature DGCs, with or without IGF2 overexpression. Overexpression of IGF2 does not appear to affect the morphology of either calretinin- or calbindin-positive DGCs. Scale bars, 50 μm.
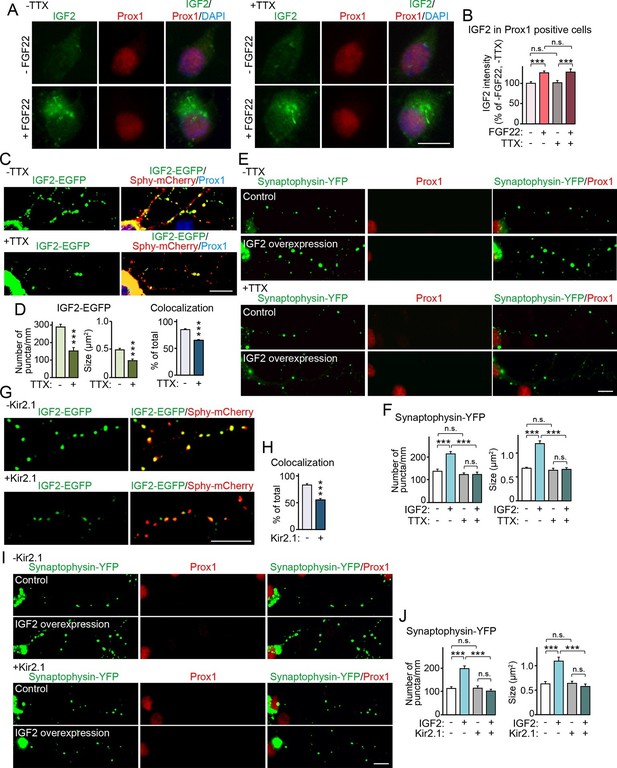
Neural activity is necessary for synaptic localization and synaptogenic effects of IGF2, but not for FGF22-dependent IGF2 expression.
(A and B) Cultured hippocampal neurons were treated with FGF22 at 1DIV, and fixed and stained at 7DIV, as in Figure 3A. At 1DIV and 5DIV, TTX was added in the media to block neuronal activity. Bath application of FGF22 increases IGF2 expression in DGCs without (left panels) as well as with activity blockade (right panels). Quantification of IGF2 immunoreactivity in the cell bodies of DGCs is shown in (B). (C–F) TTX treatment impairs synaptic localization of IGF2 and its synaptogenic function. (C and D) Cultured hippocampal neurons were transfected with the IGF2-EGFP plasmid together with synaptophysin-mCherry (Sphy-mCherry) plasmid at 3DIV, and fixed and stained at 10DIV, as in Figure 4B. At 3DIV and 7DIV, TTX was added in the media to block global neuronal activity. Quantification of the number and size of IGF2-EGFP puncta, and percentage of IGF2-EGFP puncta that colocalized with synaptophysin-mCherry puncta are shown in (D). TTX treatment decreases IGF2-EGFP clustering and synaptic localization. (E and F) Cultured hippocampal neurons were transfected with the plasmid expressing IGF2 together with the synaptophysin-YFP plasmid at 3DIV, and fixed and stained at 10DIV, as in Figure 5. TTX was added in the media at 3DIV and 7DIV. IGF2 overexpression increases clustering of synaptophysin-YFP in Prox1-positive DGCs without TTX (upper panels), but not with TTX (lower panels). (F) Quantification of the number and size of synaptophysin-YFP in DGCs with or without IGF2 overexpression in the presence or absence of TTX. (G–J) Suppression of intrinsic neuronal excitability impairs synaptic localization of IGF2 and its synaptogenic function. (G and H) Cultured hippocampal neurons were transfected with the plasmids expressing Kir2.1, IGF2-EGFP, and synaptophysin-mCherry (Sphy-mCherry) at 3DIV. Cells were fixed and stained at 10DIV. Percentage of IGF2-EGFP puncta that colocalized with synaptophysin-mCherry puncta in Prox1-positive DGCs is shown in (H). Kir2.1 expression decreases synaptic localization of IGF2-EGFP in DGCs. (I and J) Cultured hippocampal neurons were transfected with the plasmids expressing Kir2.1, IGF2, and synaptophysin-YFP at 3DIV. Cells were fixed and stained at 10DIV. (J) Quantification of the number and size of synaptophysin-YFP in DGCs with or without IGF2 overexpression in the presence or absence of Kir2.1. IGF2 overexpression increases clustering of synaptophysin-YFP in Prox1-positive DGCs, but the increase is suppressed by Kir2.1 expression. Error bars are s.e.m. Data are from (B) 35–292 cells from 3 independent experiments, (D) 20–32 cells from 3–4 independent experiments, (F) 22–24 cells from 3 independent experiments, (H) 7 cells from 3 independent experiments, (J) 20 cells from 4 independent experiments. Significant difference from control at ***p<0.0001 by two-way ANOVA followed by Tukey's multiple comparison test (B, F, and J) and by Student's t-test (D and H). n.s.: no statistical significant difference. Scale bars, 10 μm.
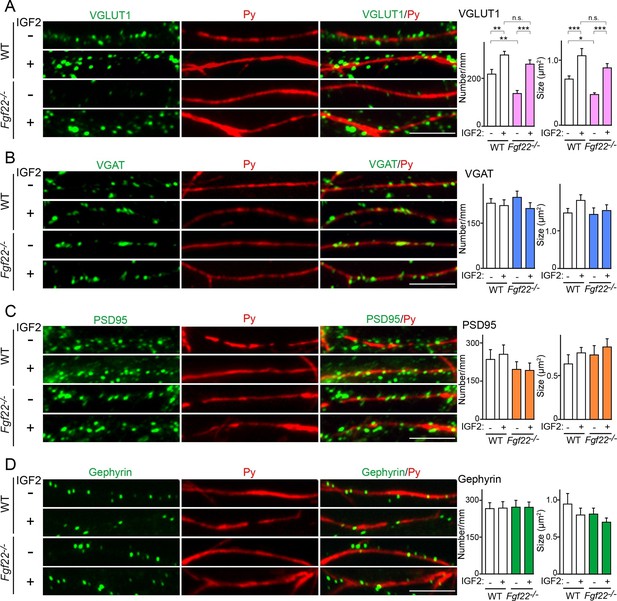
IGF2 treatment rescues the impairment of glutamatergic presynaptic development in Fgf22-/- cultures.
Hippocampal neurons from WT and Fgf22-/- mice were cultured with or without IGF2 treatment. IGF2 was applied into culture media at 1DIV, and cells were fixed and stained at 13DIV. (A) VGLUT1 clustering on the dendrites of CA3 pyramidal neurons (immunolabeled with Py antibody). IGF2 treatment increases VGLUT1 clustering in WT culture and rescues defects in VGLUT1 clustering in Fgf22-/- cultures. (B–D) Application of IGF2 does not change VGAT clustering (B), PSD95 clustering (C), and gephyrin clustering (D) on the dendrites of CA3 pyramidal neurons in WT and Fgf22-/- cultures. The density (number/mm) and size of VGLUT1, VGAT, PSD95 and gephyrin puncta on CA3 pyramidal neurons were analyzed and shown in the graphs. Error bars are s.e.m. Data are from 12–39 cells from 3–7 independent experiments. Significant difference from control at *p<0.05, **p<0.01 and ***p<0.001 by two-way ANOVA followed by Tukey's multiple comparison test. n.s.: no statistical significant difference. Scale bars, 10 μm.
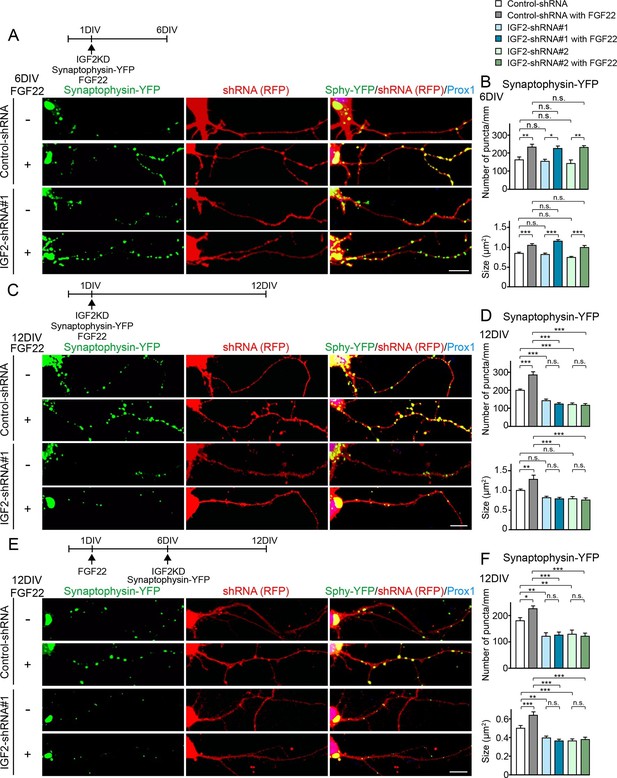
IGF2 is dispensable for FGF22-dependent initial presynaptic differentiation, but is required for subsequent presynaptic stabilization.
(A-D) Cultured hippocampal neurons were transfected with the plasmid expressing synaptophysin-YFP together with the plasmid expressing either control-shRNA, IGF2-shRNA#1, or IGF2-shRNA#2 at 1DIV. FGF22 was applied into culture media after the transfection at 1DIV. Cells were fixed, stained, and clustering of synaptophysin-YFP in DGCs was analyzed at 6DIV or 12DIV. (A and B) Clustering of synaptophysin-YFP in DGCs at 6DIV. Quantification of the number and size of synaptophysin-YFP clusters in DGCs are shown in the graph (B). FGF22 treatment increases the number and size of synaptophysin-YFP puncta in both control and IGF2-knockdown DGCs. (C and D) Clustering of synaptophysin-YFP in DGCs at 12DIV. Quantification of the number and size of synaptophysin-YFP clusters are shown in the graph (D). Without IGF2, the effects of FGF22 on synaptophysin-YFP clustering disappear. (E and F) Cultured hippocampal neurons were treated with FGF22 at 1DIV. At 6DIV, neurons were transfected with the plasmid expressing synaptophysin-YFP together with the plasmid expressing either control-shRNA, IGF2-shRNA#1, or IGF2-shRNA#2. Cells were fixed and stained at 12DIV. Quantification of the number and size of synaptophysin-YFP clusters are shown in the graph (F). IGF2-knockdown at a late stage of synapse development (from 6DIV) decreases synaptophysin-YFP clustering and blocks the synaptogenic effects of FGF22 in DGCs. Error bars are s.e.m. Data are from 12–62 cells from 5–8 independent experiments. Significant difference from control at *p<0.05, **p<0.01 and ***p<0.001 by two-way ANOVA followed by Tukey’s multiple comparison test. n.s.: no statistical significant difference. Scale bars, 10 μm.
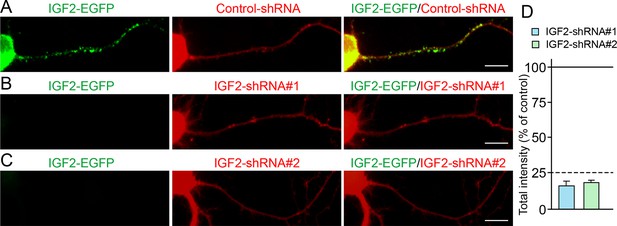
Efficiency of shRNA-mediated IGF2 knockdown.
Cultured hippocampal neurons were transfected with the plasmids expressing IGF2-EGFP and (A) control-shRNA, (B) IGF2-shRNA#1, and (C) IGF2-shRNA#2 at 3DIV. Neurons were fixed at 6DIV. Total GFP intensities in the IGF2-shRNA transfected cells (normalized to the intensity in the control-shRNA transfected cells) were analyzed and shown in (D). Both IGF2-shRNAs effectively inhibited the expression of IGF2 (to < 25% of controls). Scale bars, 10 μm.
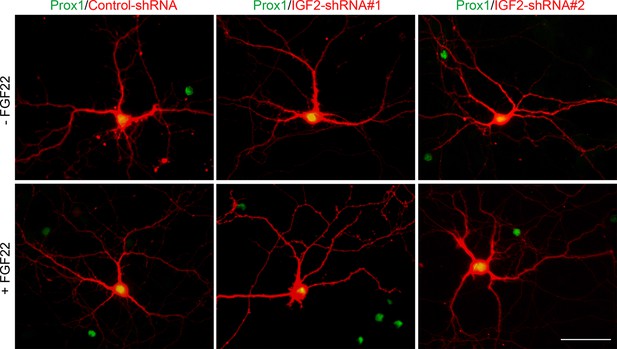
Effects of shRNA-mediated IGF2 knockdown on DGC morphology.
Cultured hippocampal neurons were transfected with the plasmid expressing either control-shRNA, IGF2-shRNA#1, or IGF2-shRNA#2 at 1DIV. FGF22 was applied into culture media after the transfection at 1DIV. Cells were fixed and stained at 12DIV for morphological observations. Morphology of transfected DGCs (labeled with co-expressing RFP) does not appear affected by shRNA-mediated knockdown of IGF2 or FGF22 treatment. Scale bar, 50 μm.
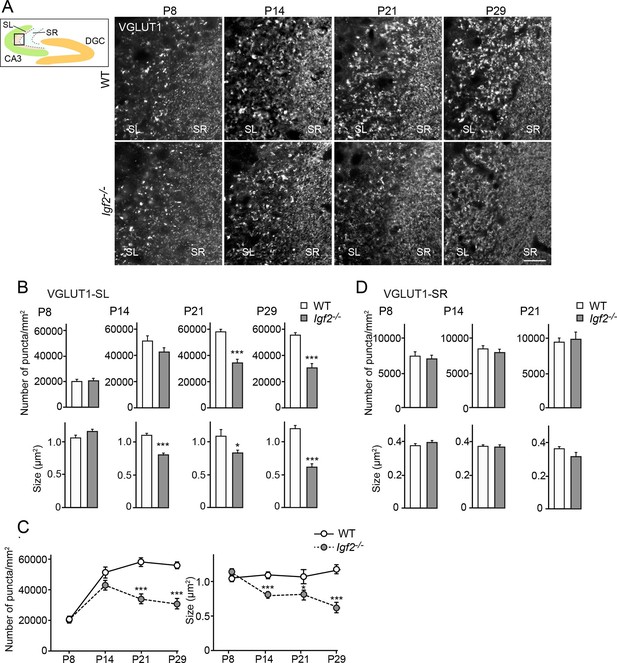
Glutamatergic presynaptic stabilization is impaired at the DGC–CA3 synapses in Igf2-/- mice.
Hippocampal sections from WT and Igf2-/- mice at P8, P14, P21, and P29 were immunostained for VGLUT1. The illustration shows the pictured area (boxed). (A) Representative pictures from CA3 regions. SL: stratum lucidum (DGC–CA3 synapses); SR: stratum radiatum (CA3–CA3 synapses). Density and size of VGLUT1 puncta are quantified and shown in (B: SL) and (D: SR). (C) Time course of VGLUT1 clustering in the SL layer. In the SL layer, Igf2-/- mice show no presynaptic defects at P8, but start to show mild defects at P14, and exhibit significant defects at P21 and P29. No significant defects were observed in the SR layer. Error bars are s.e.m. Data are from (B and C) 23–42 fields from 3–5 independent experiments, (D) 13–34 fields from 3–5 independent experiments. Significant difference from control at *p<0.05 and ***p<0.0001 by Student's t-test. Scale bar, 20 μm.
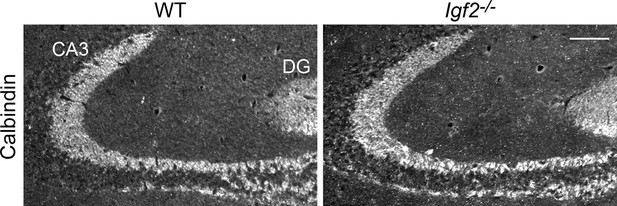
The targeting of DGC axons to CA3 appears normal in Igf2-/- mice.
Hippocampal sections from WT and Igf2-/- mice at P21 were immunostained for calbindin, which labels DGC axons (mossy fibers). No apparent defects were found in the targeting of mossy fibers to the CA3 region. Scale bar, 100 μm.
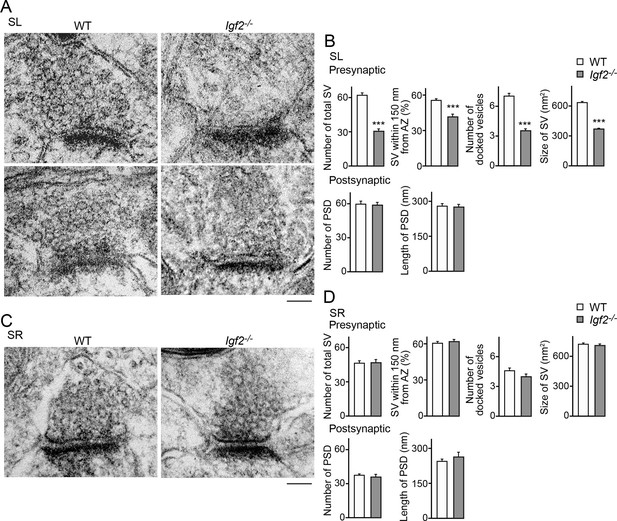
Electron microscopic analysis shows defects in excitatory presynaptic terminals selectively in the SL layer of Igf2-/- mice.
Electron microscopic analysis of asymmetric (excitatory) synapses in WT and Igf2-/- mice (P28–29). (A and B) Asymmetric synapses in the CA3 SL layer. (A) Two representative images of asymmetric synapses in WT and Igf2-/- mice. (B) Quantification of synaptic vesicles (SVs) and postsynaptic densities (PSDs). Number of SVs within 400 nm from the active zone (total SV), % SVs within 150 nm from active zone, number of docked vesicles per synapse, size of SVs, number of PSDs in 100 nm2, and length of PSDs are shown. (C and D) Asymmetric synapses in the CA3 SR layer. (C) Representative images of asymmetric synapses in WT and Igf2-/- mice. (D) Quantification of SVs and PSDs. Igf2-/- mice show a loss of SVs in the SL layer, but not in the SR layer, without apparent changes in PSDs. Error bars indicate s.e.m. Data are from 14–50 synapses from 12–13 fields from 2 mice per strain. Significant differences from WT mice at ***p<0.0001 by Student's t-test. Scale bars, 100 nm.
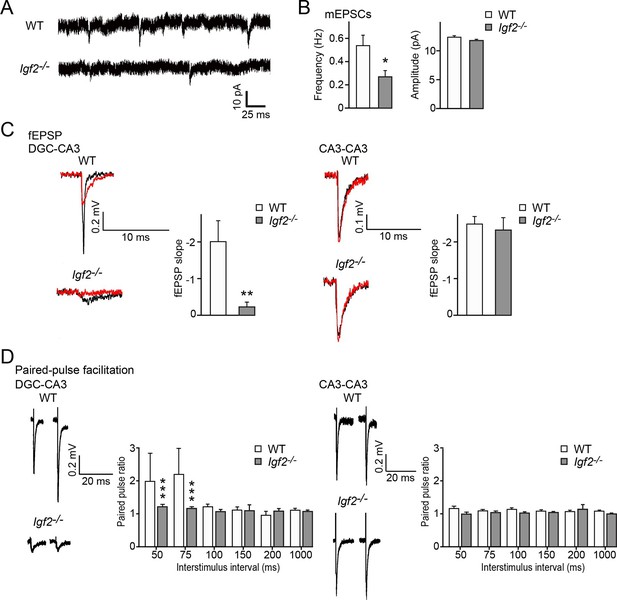
Excitatory synaptic transmission at DGC–CA3 synapses, but not CA3–CA3 synapses, is specifically impaired in Igf2-/- mice.
(A and B) Whole-cell recordings of mEPSCs from CA3 pyramidal neurons of adult WT and Igf2-/- hippocampal slices. (A) Representative traces of mEPSCs. (B) Quantification of the frequency and amplitude of mEPSCs. The frequency, but not amplitude, of mEPSCs is specifically decreased in CA3 of Igf2-/- mice. 31–32 cells from 5 mice per genotype. *p<0.05 by Student’s t-test. (C) fEPSP responses evoked in the CA3 region of the hippocampus. Responses were characterized as either DGC–CA3 or CA3–CA3 based upon sensitivity to DCG-IV treatment. Black traces: original responses, red traces: after 10 min of DCG-IV treatment. Graphs show the quantification of the maximum fEPSP slope. At DGC–CA3 synapses, the maximum elicited response is significantly smaller in Igf2-/- mice than in WT mice (n = 8 and 8; **p<0.01 by Student’s t-test). At CA3–CA3 synapses, the maximum elicited response is not different between WT and Igf2-/- mice (n = 14 and 24; p = 0.71 by Student’s t-test). (D) Paired pulse facilitation (PPF). PPF at the DGC–CA3 synapses, and not CA3–CA3 synapses, is significantly decreased in Igf2-/- mice (***p<0.0001 by Two-way ANOVA followed by a Tukey test). Example traces demonstrate responses with a 50 ms inter-stimulus interval.
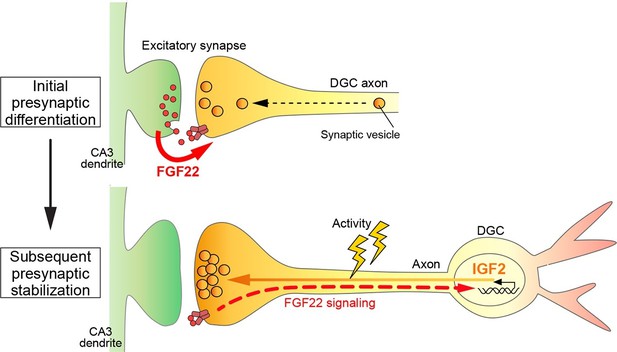
A Model showing a feedback signal for activity-dependent presynaptic stabilization.
For initial presynaptic differentiation, target-derived FGF22 locally promotes clustering of synaptic vesicles. For subsequent presynaptic stabilization, FGF22 signaling induces the expression of IGF2. IGF2, in turn, localizes to presynaptic terminals in an activity-dependent manner and stabilizes them.
Tables
List of genes that are significantly downregulated in DGCs of Fgf22-/- mice at P14. Genes with Diff Score < -33 (p-value <0.001) relative to WT are shown in the table.
Symbol | Definition | Diff Score |
---|---|---|
Slc6a13 | Solute carrier family 6 (Neurotransmitter Transporter), member 13 | -56.042 |
Col6a1 | Collagen, type VI, alpha 1 | -52.859 |
Prelp | Proline arginine-rich end leucine-rich repeat | -50.844 |
Igf2 | Insulin-like growth factor 2 | -42.987 |
Mrc2 | Mannose receptor, C Type 2 | -41.904 |
Gys3 | Glycogen synthase 3, brain | -41.834 |
Aebp1 | Adipocyte enhancer-binding protein 1 | -40.069 |
Lrrtm3 | Leucine rich repeat transmembrane neuronal 3 | -35.357 |
Zfp365 | Zinc finger protein 365 | -34.604 |
Col1a1 | Collagen, type I, alpha 1 | -33.444 |