Ion Channels: Signaling the differences between cilia
Almost all eukaryotic cells have hair-like projections called cilia on their surface. Cilia sense environmental cues, play crucial roles in organ development, and are responsible for moving cells and fluids. Problems that affect how cilia work cause a diverse set of ‘ciliopathies’, including polycystic kidney disease, infertility, airway diseases and hearing defects (Fliegauf et al., 2007). But how do cilia differ among various cell types?
Calcium ion (Ca2+) signaling is central for controlling how cilia move and respond to signals received from outside the cell (Phua et al., 2015). Now, in eLife, Julia Doerner, Markus Delling and David Clapham at Harvard Medical School and Boston Children’s Hospital demonstrate that the key principles of Ca2+ signaling differ significantly between different types of cilia (Doerner et al., 2015).
Cilia are categorized as either primary cilia or undulipodia based on obvious structural differences (Figure 1). Primary cilia are not able to move themselves, but help cells detect external cues and serve as a signaling hub during development (Goetz and Anderson, 2010). In contrast, undulipodia are able to move themselves and are subdivided into motile cilia that move fluid over the outer surface of the cell, and flagella that propel the cell through fluids (Fliegauf et al., 2007). Ciliary movement is directly associated with the axoneme – a structure made of microtubules that serves as a ‘skeleton’ and extends along the length of the cilium. All primary cilia contain nine pairs of microtubules that are arranged in a circle to give the so called 9+0 structure. Undulipodia have two additional microtubules in the center of the axoneme (the 9+2 arrangement; Figure 1).
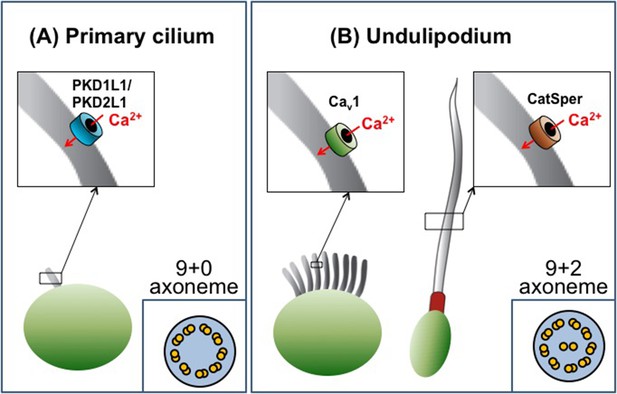
The diversity of cilia.
There are two types of cilia, primary cilia and undulipodia, which are distinguished by differences in the structure of their axoneme. The level of calcium ions (Ca2+) inside the cilia is regulated by specialized ion channels. (A) The main Ca2+ channel for primary cilia has been identified as the PKD1L1/PKD2L1 complex. (B) There are two types of undulipodia: motile cilia and flagella. The main Ca2+ channel of mammalian flagella was identified as a protein called CatSper in 2013. Doerner et al. have now identified an L-type voltage-gated Ca2+ channel (Cav1 family) as the main Ca2+ channel for motile cilia.
Beyond these obvious differences in structure, differences in the molecular pathways that control how the cilia work remained largely unknown. Direct investigation of the molecular composition of cilia is challenging, mainly due to their small size, the extremely low volume of ciliary cytosol, and the tight structural associations between various molecular components. Together, these adverse factors complicate the application of all biochemical, electrophysiological, or optical analytic methods.
However, since 2001 the Clapham lab has been instrumental in using the patch-clamp technique to study various ciliary structures, starting with the discovery of a mysterious channel-like protein located in the sperm flagellum (Ren et al., 2001). This protein, called CatSper, is structurally similar to the voltage-gated Ca2+ channels found in neurons. However, in the CatSper channel four individual subunits work together to form a functional channel, whereas in neuronal channels the four subunits are linked into a single molecule. Applying the whole-cell patch-clamp technique to sperm cells revealed that CatSper is only weakly voltage-dependent, defying expectations. Instead, its activity is controlled by the pH inside the cell (Kirichok et al., 2006) and by the female steroid hormone progesterone (Lishko et al., 2011; Strunker et al., 2011). CatSper is now established as the principal, and perhaps only, Ca2+ channel in the flagella of sperm cells and is, therefore, indispensable for normal sperm motility and male fertility (Figure 1).
In another technological and conceptual breakthrough in 2013, the Clapham group applied the patch-clamp technique directly to primary cilia and found that the main Ca2+ channel of this organelle consists of two subunits: PKD1L1 and PKD2L1 (DeCaen et al., 2013; Delling et al., 2013; Figure 1). These proteins belong to a group of proteins called polycystins, some of which are mutated in patients with polycystic kidney disease. In mice, PKD1L1 mutations disrupt left-right patterning and cause the developing embryos to die (Field et al, 2011). In contrast, PKD2L1-deficient mice can survive, but demonstrate intestinal malrotation – a condition that causes parts of the gut to develop in the wrong positions (Delling et al., 2013). The Clapham group demonstrated that PKD1L1-PKD2L1 channels in the ciliary membrane cause a resting Ca2+ concentration that is approximately five times greater in the primary cilia than in the cell body. Thus, primary cilia are a specialized Ca2+ signaling organelle, in which Ca2+ may be required for regulating sonic hedgehog and other signaling pathways.
Doerner, Delling and Clapham have now made the first patch-clamp recordings on motile cilia. The recordings were made on ependymal cells, which line fluid-filled spaces in the brain and spinal cord, and revealed several differences between motile cilia and other cilia types. Firstly, the abundance of Ca2+ channels in the plasma membrane of motile cilia appears to be much lower than in primary cilia and flagella. Only one type of Ca2+ channel was apparently present in the motile cilia of ependymal cells: a voltage-gated Ca2+ channel (in the Cav1 subfamily). Moreover, the ciliary membrane contained many fewer of these channels than the plasma membrane of the rest of the cell (Figure 1).
Furthermore, Doerner et al. found that the resting Ca2+ concentration in the motile cilia was much lower than that in primary cilia. Finally, although the voltage-gated Ca2+ channels cause a substantial elevation of the Ca2+ concentration inside the cilium upon membrane depolarization, this does not measurably change ciliary motility. This is in striking contrast to the sperm flagellum, where motility is strongly regulated by the movement of Ca2+ ions through CatSper. However, a possibility still remains that in addition to voltage-gated Ca2+ channels, motile cilia contain other, yet unidentified, types of Ca2+ channels that would be more efficient in controlling ciliary beat.
The remarkable technical and conceptual advances made by the Clapham group should lead to the discovery of other ion channels and new regulatory pathways in these fascinating structures.
References
-
When cilia go bad: cilia defects and ciliopathiesNature Reviews Molecular Cell Biology 8:880–893.https://doi.org/10.1038/nrm2278
-
The primary cilium: a signalling centre during vertebrate developmentNature Reviews Genetics 11:331–344.https://doi.org/10.1038/nrg2774
Article and author information
Author details
Publication history
- Version of Record published: December 23, 2015 (version 1)
Copyright
© 2015, Lishko et al.
This article is distributed under the terms of the Creative Commons Attribution License, which permits unrestricted use and redistribution provided that the original author and source are credited.
Metrics
-
- 2,209
- views
-
- 360
- downloads
-
- 5
- citations
Views, downloads and citations are aggregated across all versions of this paper published by eLife.
Download links
Downloads (link to download the article as PDF)
Open citations (links to open the citations from this article in various online reference manager services)
Cite this article (links to download the citations from this article in formats compatible with various reference manager tools)
Further reading
-
- Structural Biology and Molecular Biophysics
The proteasome controls levels of most cellular proteins, and its activity is regulated under stress, quiescence, and inflammation. However, factors determining the proteasomal degradation rate remain poorly understood. Proteasome substrates are conjugated with small proteins (tags) like ubiquitin and Fat10 to target them to the proteasome. It is unclear if the structural plasticity of proteasome-targeting tags can influence substrate degradation. Fat10 is upregulated during inflammation, and its substrates undergo rapid proteasomal degradation. We report that the degradation rate of Fat10 substrates critically depends on the structural plasticity of Fat10. While the ubiquitin tag is recycled at the proteasome, Fat10 is degraded with the substrate. Our results suggest significantly lower thermodynamic stability and faster mechanical unfolding in Fat10 compared to ubiquitin. Long-range salt bridges are absent in the Fat10 structure, creating a plastic protein with partially unstructured regions suitable for proteasome engagement. Fat10 plasticity destabilizes substrates significantly and creates partially unstructured regions in the substrate to enhance degradation. NMR-relaxation-derived order parameters and temperature dependence of chemical shifts identify the Fat10-induced partially unstructured regions in the substrate, which correlated excellently to Fat10-substrate contacts, suggesting that the tag-substrate collision destabilizes the substrate. These results highlight a strong dependence of proteasomal degradation on the structural plasticity and thermodynamic properties of the proteasome-targeting tags.
-
- Biochemistry and Chemical Biology
- Structural Biology and Molecular Biophysics
The articles in this special issue highlight how modern cellular, biochemical, biophysical and computational techniques are allowing deeper and more detailed studies of allosteric kinase regulation.