CO2-evoked release of PGE2 modulates sighs and inspiration as demonstrated in brainstem organotypic culture
Figures
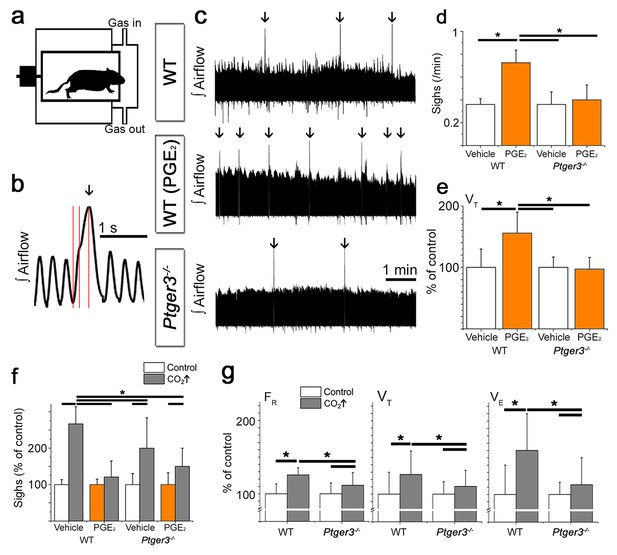
PGE2 and CO2 increase sigh activity via EP3R signaling.
Respiratory activity was recorded in vivo in a two-chamber plethysmograph (a). Sighs, defined by an increase in inspiratory volume and respiratory cycle period with a biphasic inspiration (b), increase in frequency after intracerebroventricular injection (i.c.v.) of PGE2. This effect is absent in mice lacking EP3R (Ptger3-/-, c, arrows, d). I.c.v. injection of PGE2 also increases the tidal volume (VT) in wild-type C57BL/6J (WT) mice (e). The sigh frequency is increased by hypercapnic (5% CO2 in normoxia) conditions in wild-type and Ptger3-/- mice but less so in Ptger3-/- mice (f). In wild-type mice, the increase is abolished after i.c.v. injection of PGE2 (f). Hypercapnic exposure causes an increase in respiratory frequency (FR), tidal volume (VT), and minute ventilation (VE) (g), but the increase is attenuated in Ptger3-/- mice. Data are presented as means ± SD. *p<0.05 Source data are available in a separate source data file.
-
Figure 1—source data 1
In vivo plethysmography data.
- https://doi.org/10.7554/eLife.14170.005
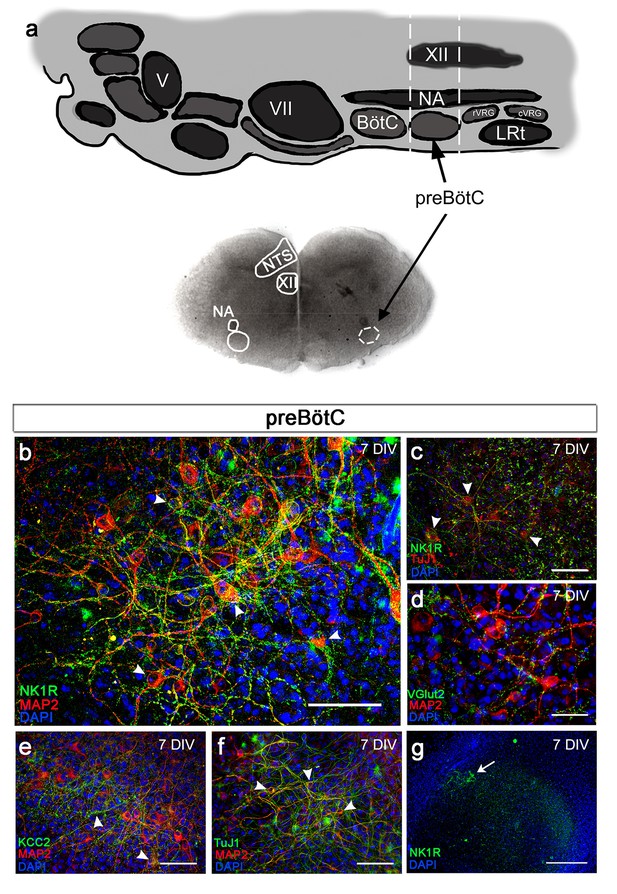
Brainstem slice cultures have a preserved structure and neurons with functional potential.
Brainstem slices containing the preBötC were used to create slice cultures. Anatomical landmarks, including the nucleus ambiguus (NA), nucleus tractus solitarius (NTS), and nucleus hypoglossus (XII; a), as well as the distinct expression of NK1R (b, c, g) enabled the identification of the preBötC region. The brainstem slice displayed MAP2-/Tuj1-positive neurons expressing NK1R (b, c), VGlut2 (d), and/or KCC2 (e). The abundant MAP2-/Tuj1-positive cells demonstrated a preserved neuronal network within the preBötC (g). KCC2 expression was found in the NTS, NA, and preBötC (e). DIV; days in vitro. Arrowheads: double-labeled cells. Scale bars: 100 µm in b–f, 500 µm in g.
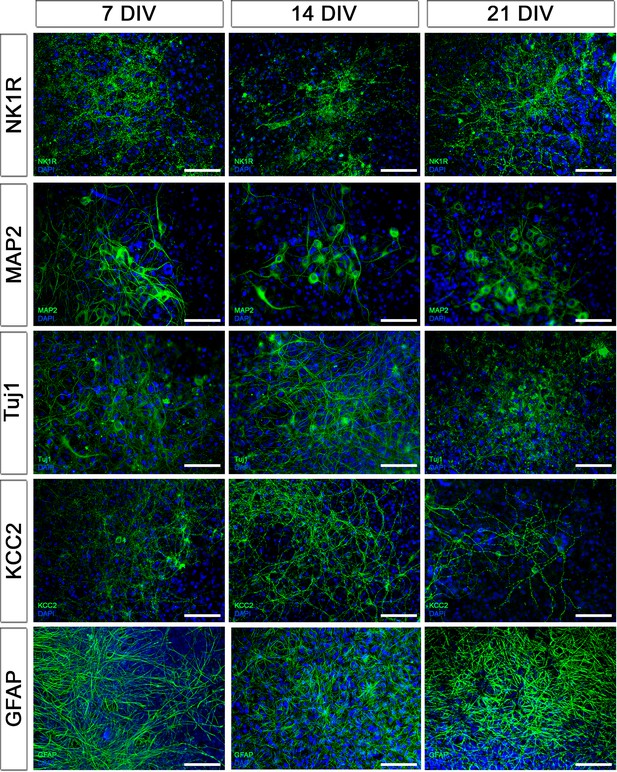
Protein expression pattern is preserved during cultivation.
The expression pattern of neuronal the markers NK1R, MAP2, Tuj1 and KCC2 and the astrocyte marker GFAP did not change during cultivation for 3 weeks. DIV: days in vitro. Scale bars: 100 µm.

Slices flatten during cultivation.
The gross morphology of the slices changed slightly during cultivation due to thinning and spreading. DIV: days in vitro. Scale bar: 500 µm.

Brainstem slice cultures are viable.
An individual single necrotic cells (8 ± 3%, n=257, propidium iodide-stained) were found in the brainstem slice cultures (N=12) that had been cultivated for 3 weeks, but no large clusters of necrotic cells were detected (a, d). The few necrotic cells were observed in the thickest regions, indicating that diffusion-based oxygenation is critically dependent on slice thickness. Oxygen glucose deprivation (OGD) for 1 hr produced clear positive PI-staining throughout the slice (b; N=5). Cultures also showed low apoptotic activity (2 ± 1%, n=187), as evaluated by caspase-3 staining (c, d; N=20). DIV: days in vitro. N: slices, n: cells. Scale bars: 100 µm.
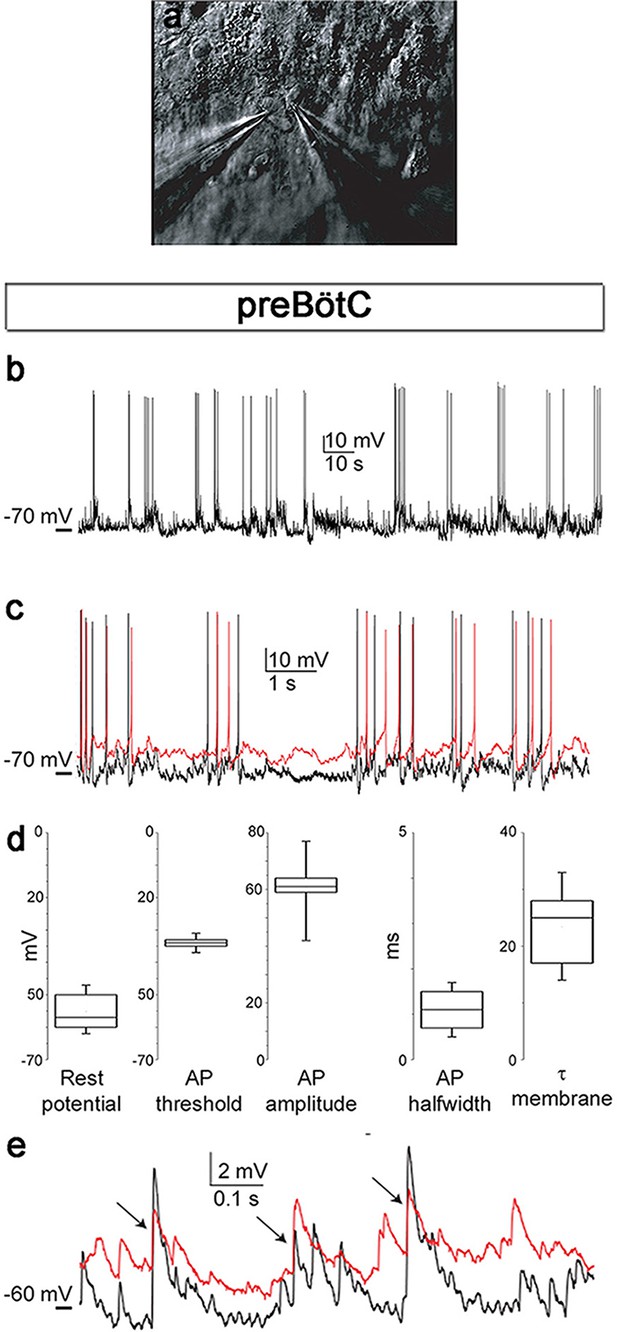
Neuronal electrical activity indicates preserved networks.
Neurons in a preBötC slice (7 DIV), patched in the whole-cell configuration in current-clamp mode (a), exhibit regular rhythmic bursting activity (b). The neurons exhibited a hyperpolarized resting potential, action potentials, synaptic input, and spontaneous electrical activity, with epochs of action potential activity (b, c). The different measured variables indicated healthy and normally functioning neurons (d). Depicted here are two simultaneously patched neurons that also received common synaptic input (e, arrows). Spiking epochs occurred simultaneously, suggesting synchronized network oscillations. Direct connectivity between the depicted neurons showed that they were neither chemically nor electrically synaptically connected to each other. This finding indicates that the observed correlation was induced by common input from a preserved network structure. AP: action potential. DIV: days in vitro. Source data are available in a separate source data file.
-
Figure 3—source data 1
Electrophysiology patch clamp data.
- https://doi.org/10.7554/eLife.14170.012
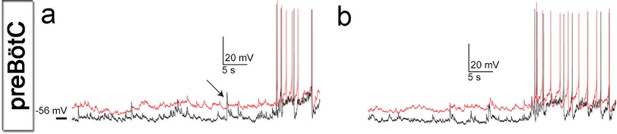
Cells of brainstem slice cultures retain neuronal electrical properties.
Recorded neurons showed correlated epochs of spontaneous activity (a, b) and received synaptic input that was often synchronized (a, arrow), suggesting common presynaptic neurons. Note the similarity in neuronal properties of the recorded neighboring neurons (a, b). Source data are available in a separate source data file.
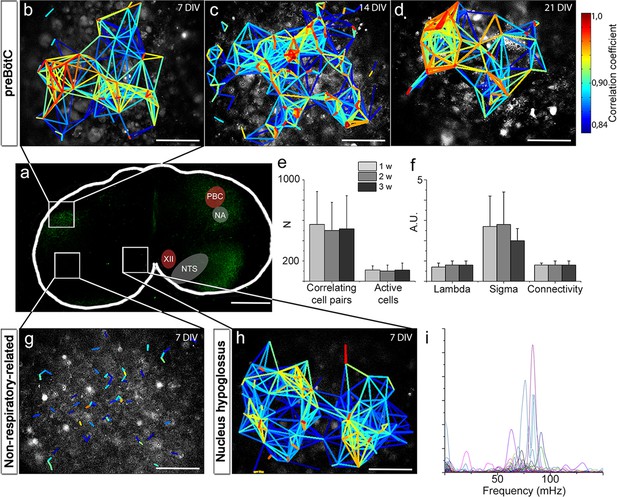
Neural activity in the preBötC is arranged in a functional respiratory network with respiratory-related motor output.
In the preBötC slice (a), a cross-correlation analysis of Ca2+ time-lapse imaging data (Figure 4—figure supplement 1) revealed small-world network-structured correlated activity in the preBötC (b–d). The number of correlating cell pairs did not change over time (e), nor did the small-world network parameter or connectivity (f). TMR-SP-positive regions contained more correlated cell pairs than TMR-SP-negative regions (621 ± 284, N=14 and 56 ± 48, N=9, respectively; p<0.05), although there was no difference in the number of active cells (112 ± 57, N=14 and 144 ± 68, N=9, respectively, N.S.; g). As in the preBötC, the nucleus hypoglossus maintained correlated neural network activity (h). Ten percent of the cells (n=8–12/slice) in the hypoglossal nucleus exhibited a regular spiking frequency of ~50–100 mHz (i). The multicolored bar indicates the correlation coefficient in b–h; warmer colors indicate more strongly correlated activity between two cells connected by the line. DIV: days in vitro. A.U.: arbitrary units. w: week. N: number of slices, n: number of cells. Scale bars: 500 µm in a, 100 µm in b–d and g–h. Multicolored bar: color-coded correlation coefficient values. Data are presented as means ± SD. Source data are available in a separate source data file.
-
Figure 4—source data 1
Correlation data preBötC.
- https://doi.org/10.7554/eLife.14170.015
-
Figure 4—source data 2
Frequency data with DAMGO.
- https://doi.org/10.7554/eLife.14170.016
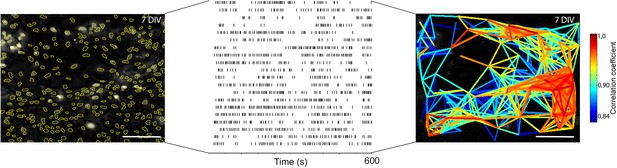
Single cell events provide information about correlated activity.
Ca2+ signals are measured in over 200 regions of interest in a single experiment. Events, here simplified as peak maxima with a minimum 20% increase above baseline, over time (lines) are identified. The locations of such events, or rather variations in the Ca2+ signal, in both time and space are used to calculate the correlation coefficient between the cells (Smedler et al., 2014). Those correlation coefficients are then drawn as lines between their corresponding cells, providing a graphical image of the network structure. DIV: days in vitro. Scale bar: 100 µm. Multicolored bar: color-coded correlation coefficient values.
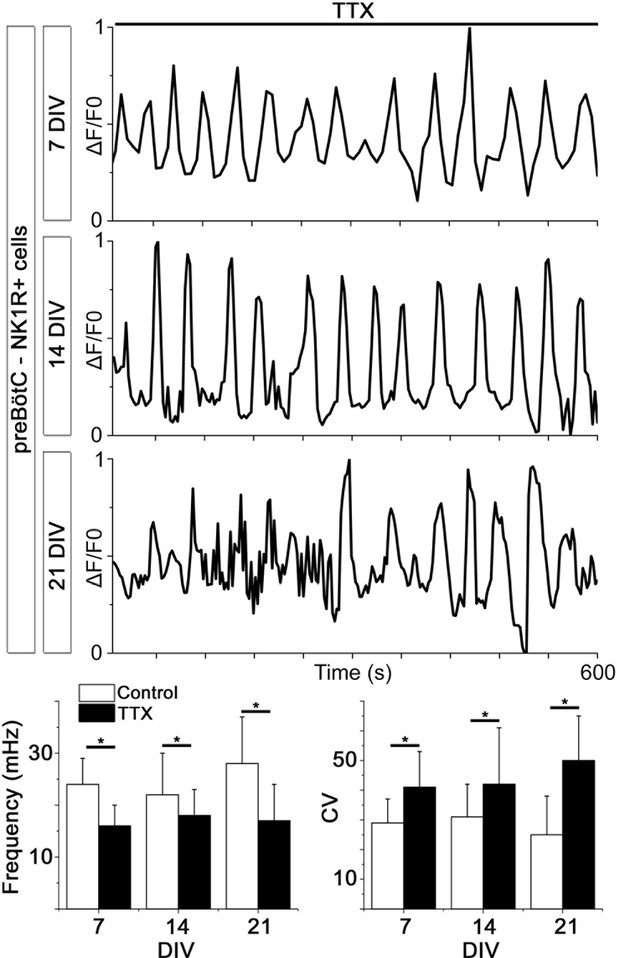
Spontaneous Ca2+ activity is preserved for 3 weeks.
NK1R-expressing neurons exhibit rhythmic Ca2+ activity after 1, 2, and 3 weeks of cultivation, even during treatment with TTX, which inhibits synapse signaling. There were no significant differences in the average frequency or regularity among slice cultures of different ages. n=840 at 7 DIV, n=621 at 14 DIV and n=456 at 21 DIV. DIV: days in vitro. n: number of cells. *p<0.05.
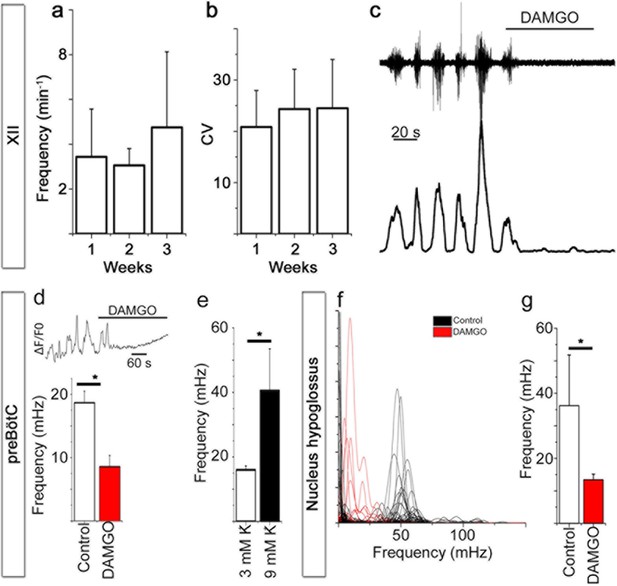
Breathing brainstem in a dish: ongoing/persistent rhythmic XII motor activity.
The connected preBötC neural networks generate respiratory-related motor neuronal output delivered through the 12th cranial nerve (XII). The hypoglossal nucleus/nerve discharge frequency varied among the brainstem slice cultures but did not depend on brainstem slice culture age (a, N=16 at 7 DIV, N=3 at 14 DIV, and N=6 at 21 DIV). The regularity of respiration-related motor activity, measured as CV (coefficient of variation), remained stable during 3 weeks of culture (b). The µ-opioid receptor agonist DAMGO (0.5 µM) silenced the XII nerve activity in 5/5 brainstem slice cultures, as depicted here in (c) from a 7-DIV brainstem culture (filtered trace, above, and rectified and smoothed trace, below). DAMGO lowered the Ca2+. In the hypoglossal nucleus, DAMGO (0.5 μM) lowered the frequency of regularly-spiking cells (f, g). N: number of slices. Data are presented as means ± SD. *p<0.05 Source data are available in a separate source data file.
-
Figure 5—source data 1
12th cranial nerve electrophysiology recordings.
- https://doi.org/10.7554/eLife.14170.021
-
Figure 5—source data 2
Frequency data with DAMGO.
- https://doi.org/10.7554/eLife.14170.022
-
Figure 5—source data 3
High potassium frequency data.
- https://doi.org/10.7554/eLife.14170.023
-
Figure 5—source data 4
Network topology and frequency data with DAMGO.
- https://doi.org/10.7554/eLife.14170.024
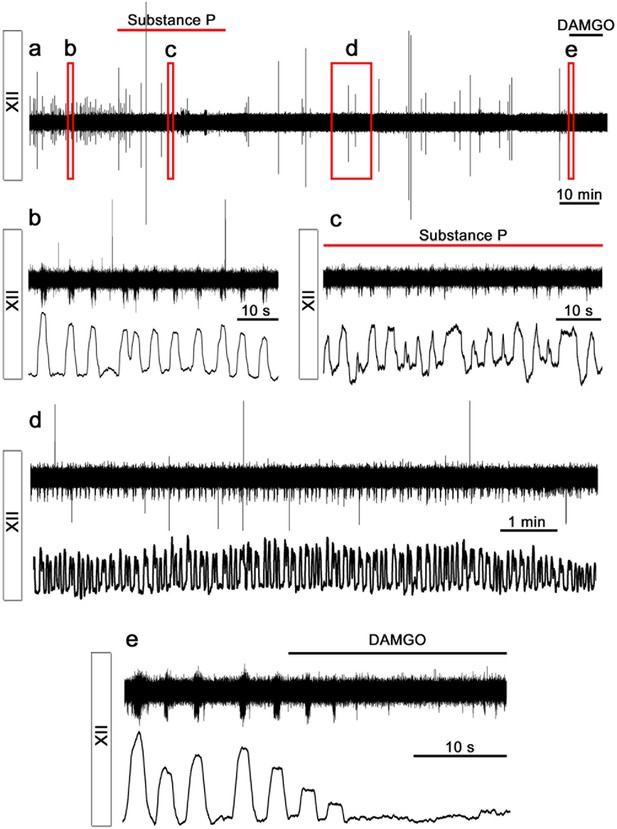
Rhythmic respiratory-related output is preserved.
Here, the rhythmic respiratory-related output, recorded from the hypoglossal motor nucleus of a 3-week-old brainstem slice culture (N=6), is displayed. Panels show filtered (above) and rectified and smoothed (below) traces of the extracellular recording. Rhythmic activity was maintained for 137 min (a). The frequency was regular (b) and increased after Substance P application (1 µM; N=7; c). Thirty minutes after Substance P application, the respiratory-related rhythm returned to the control frequency (d). The activity was inhibited by DAMGO (0.5 µM; N=5, e). N: number of slices.
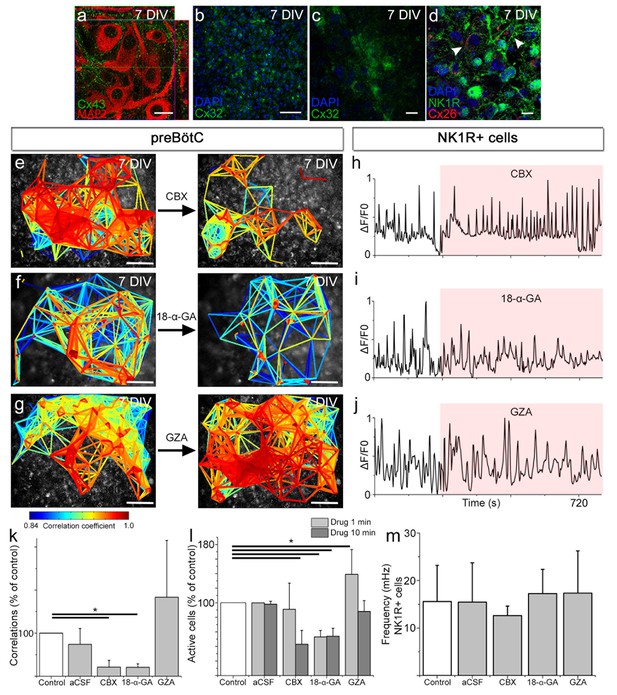
Gap junctions are necessary to maintain part of the correlated respiratory network.
In the respiratory regions, the gap junction proteins Cx43 (a, N=9), Cx32 (b, c, N=8), and Cx26 (d, arrowheads; double-labeling with NK1R, N=5) are present. Gap junction inhibitors CBX (e) and 18-α-GA (f) reduced network synchronization in the preBötC. Notably, the Ca2+ activity of individual NK1R-positive cells was not affected (h–j, m). Correlating cell pair numbers decreased to 21% (N=8) and 20% (N=6) of their respective controls after treatment with CBX and 18-α-GA, respectively (k). Network properties were not affected by GZA, an analog to CBX that lacks the ability to block gap junctions, (g, j–k, N=7) or aCSF (N=8). An initial increase in fluorescence intensity was noted after adding CBX and GZA but not after adding 18-α-GA, indicating an immediate excitatory effect of CBX and GZA (l). 18-α-GA reduced the number of active cells in the network at 1 min after application (53%), but CBX did not (91%, N.S.). At the same time point, an increased number of active cells were observed with GZA treatment (139%). After 10 min, a reduction of the number of active cells was found after treatment with both 18-α-GA and CBX (54% and 43%). However, the number of active cells returned to normal after GZA application (89%, N.S.; l). DIV: days in vitro. N: number of slices. Scale bars: 10 µm in a, c, and d, 100 µm in others. Multicolored bar: color-coded correlation coefficient values. Data are presented as means ± SD. *p<0.05. Source data are available in a separate source data file.
-
Figure 6—source data 1
Gap junction inhibition data.
- https://doi.org/10.7554/eLife.14170.028
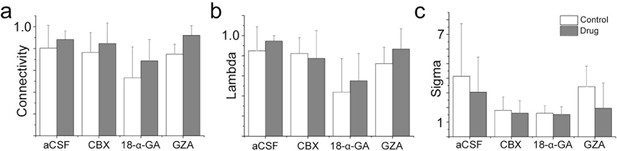
A gap junction-independent network is present within the preBötC.
Gap junction inhibitors did not affect the general topology of the respiratory network (a–c). Data are presented as means ± SD. Source data are available in a separate source data file.
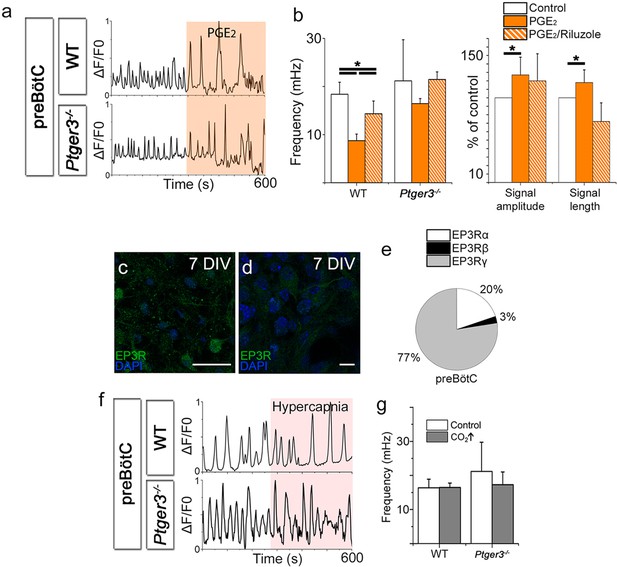
PGE2 modulates preBötC network activity.
PGE2 lowered the Ca2+ signaling frequency of the preBötC network in WT mice but not in Ptger3-/- mice (a–b). The effect was attenuated but not abolished by Riluzole (b). PGE2 also increased signal amplitude and length (a–b), an effect that was abolished after Riluzole application (b). Ptger3 is expressed in the preBötC (c, d), and 20% of the EP3Rs were of the α (Gi-protein coupled) subtype and 77% of the γ (Gs-protein coupled) subtype (e). Hypercapnic exposure (pCO2 elevated from 4.6 to 6.6 kPa) did not affect the signal frequency of the preBötC (f–g). DIV: days in vitro. Scale bars: 50 µm in c and 10 µm in d. *p<0.05 Source data are available in a separate source data file.
-
Figure 7—source data 1
PGE2 data preBötC.
- https://doi.org/10.7554/eLife.14170.031
-
Figure 7—source data 2
Hypercapnia data preBötC.
- https://doi.org/10.7554/eLife.14170.032
-
Figure 7—source data 3
Hypercapnia data preBötC 2.
- https://doi.org/10.7554/eLife.14170.033
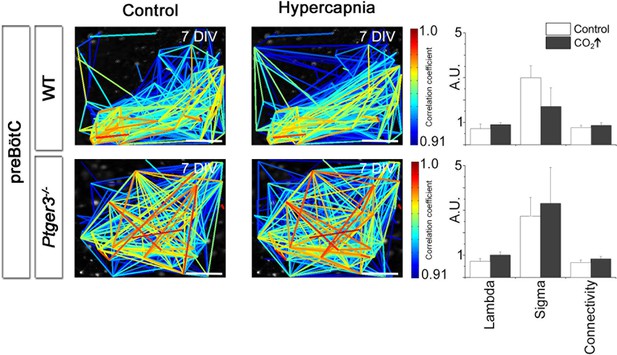
Hypercapnia had no effect on the preBötC.
Hypercapnic exposure (pCO2 elevated to 6.6 kPa) did not affect the preBötC network structure. DIV: days in vitro.
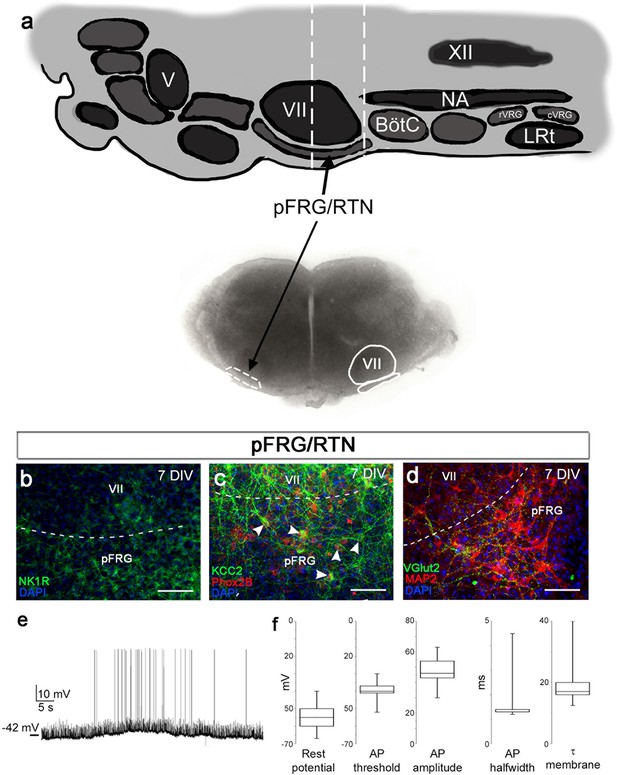
pFRG/RTN brainstem slice culture.
pFRG/RTN slices were selected based on the location of the facial nucleus (VII; a). In the brainstem slice culture, pFRG/RTN expressed the neuronal markers NK1R (b), KCC2 (c), Phox2b (c), vGlut2 (d), and MAP2 (d). The pFRG/RTN neurons also retained adequate electrical properties and generated spontaneous action potentials individually or in clusters (e–f). Data are presented as box plots with minimum and maximum values. DIV: days in vitro. Scale bars: 100 µm.
-
Figure 8—source data 1
pFRG/RTN characterization.
- https://doi.org/10.7554/eLife.14170.036
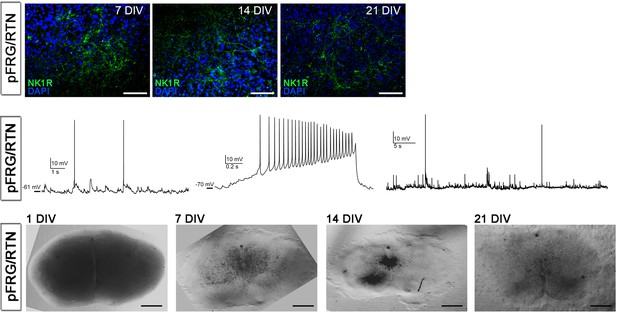
Cultivation of pFRG/RTN slices.
NK1R expression was preserved in the pFRG/RTN during cultivation (upper panel). Whole-cell recordings from neurons in 1-week-old pFRG/RTN brainstem slice cultures shows the existence of spontaneous synaptic input as well as excitable membrane properties (middle panel). The overall morphology of the pFRG/RTN brainstem slice cultures changed slightly during cultivation, becoming thinner (lower panel). DIV: days in vitro. Scale bars: 100 µm in upper panel, 500 µm in lower panel.
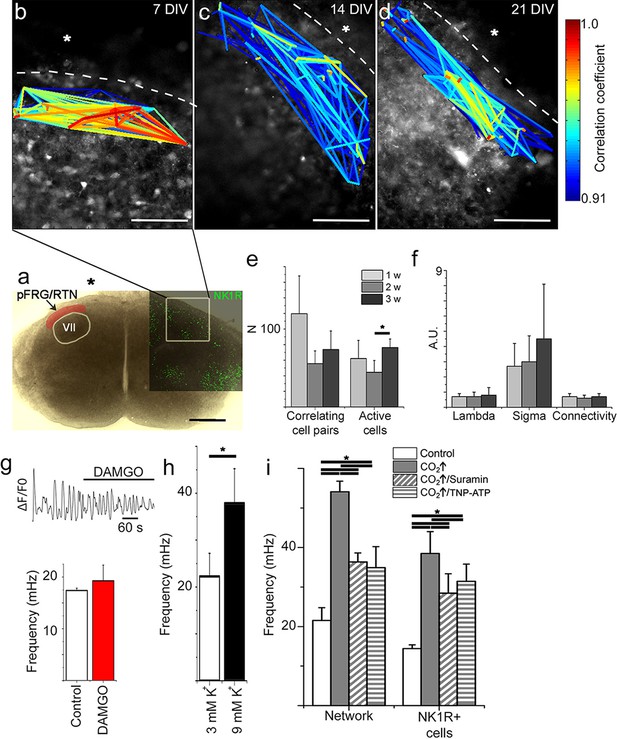
The pFRG/RTN respiration-related network generates correlated neural activity and responds to CO2.
The pFRG/RTN network is arranged in a small-world manner just ventral to the facial nucleus. The network structure was preserved during cultivation (a–d). The number of correlating cell pairs did not change with longer culturing times, but the number of active cells was higher at 3 weeks than at 2 weeks (45 ± 27<76 ± 19, p<0,05; e). The network parameters were stable during cultivation (f). The pFRG/RTN network did not respond to the µ-opioid receptor agonist DAMGO (0.5 µM; n=420, N=4; a), but the average network frequency increased with higher potassium concentrations (22 ± 5 mHz and 38 ± 7 mHz, N=12; b). Both the neural network and individual NK1R/TMR-SP-labeled cells responded to increases in CO2 pressure (pCO2 elevated to 6.6 kPa), indicating that the chemosensitivity was preserved in the pFRG/RTN brainstem slice culture. Suramin, a P2 receptor antagonist, and TNP-APT, a P2X receptor antagonist, attenuated the CO2 response but did not abolish it (g). DIV: days in vitro. Scale bars: 100 µm. Multicolored bar: color-coded correlation coefficient values. N: number of slices, n: number of cells. Data are presented as means ± SD. *p<0.05. Source data are available in a separate source data file.
-
Figure 9—source data 1
Correlation data pFRG/RTN.
- https://doi.org/10.7554/eLife.14170.039
-
Figure 9—source data 2
Hypercapnia data.
- https://doi.org/10.7554/eLife.14170.040
-
Figure 9—source data 3
High potassium frequency data.
- https://doi.org/10.7554/eLife.14170.041
-
Figure 9—source data 4
Riluzole and TTX data.
- https://doi.org/10.7554/eLife.14170.042
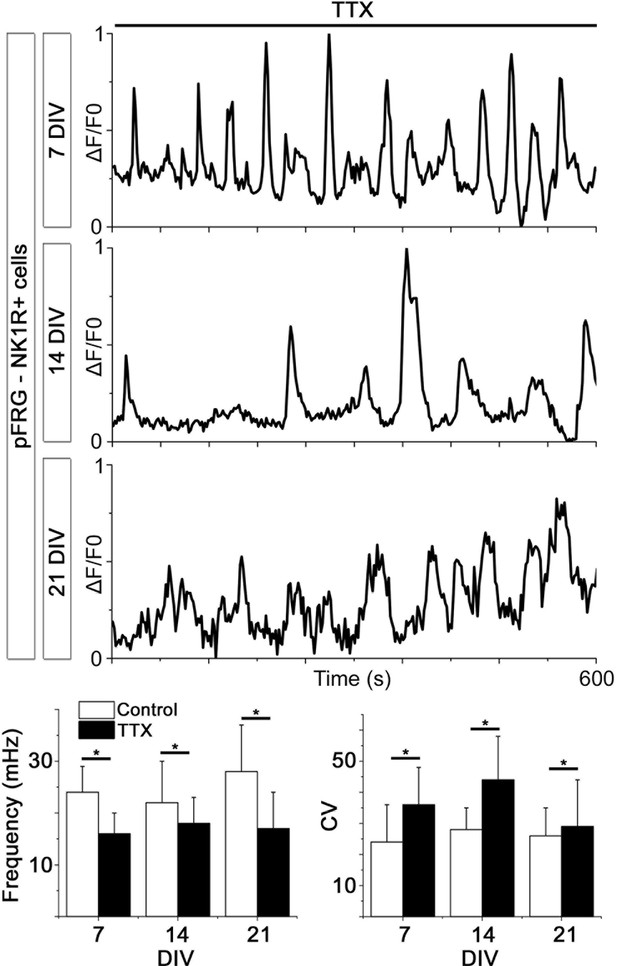
Spontaneous Ca2+ activity is preserved during cultivation.
NK1R-expressing neurons have spontaneous Ca2+ activity after 1, 2, and 3 weeks of cultivation. This activity remains during synaptic inhibition via addition of TTX. Within the culture, the frequency varied between cells, but there were no significant differences in average frequency or regularity among cultures of different ages. n=315 at 7 DIV, n=429 at 14 DIV, and n=192 at 21 DIV. DIV: days in vitro. n: number of cells. *p<0.05.
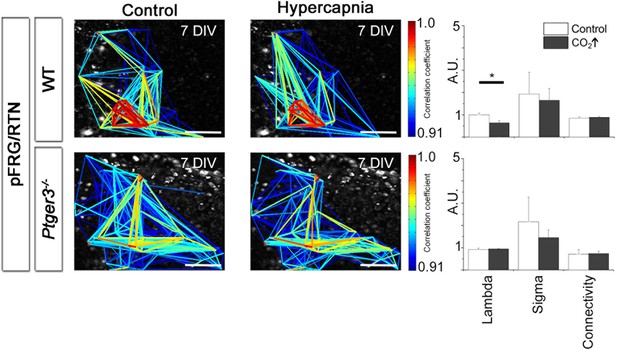
Hypercapnia reduces mean path lengths in the pFRG/RTN of wild-type mice.
A decrease in mean path length during hypercapnia was seen in the wild-type pFRG/RTN (N=18). Other parameters remained unchanged. No parameters were affected in the Ptger3-/- pFRG/RTN (N=7). N: number of slices. DIV: days in vitro. Scale bars: 100 µm. Multicolored bar: color-coded correlation coefficient values. Data are presented as means ± SD. *p<0.05. Source data are available in a separate source data file.
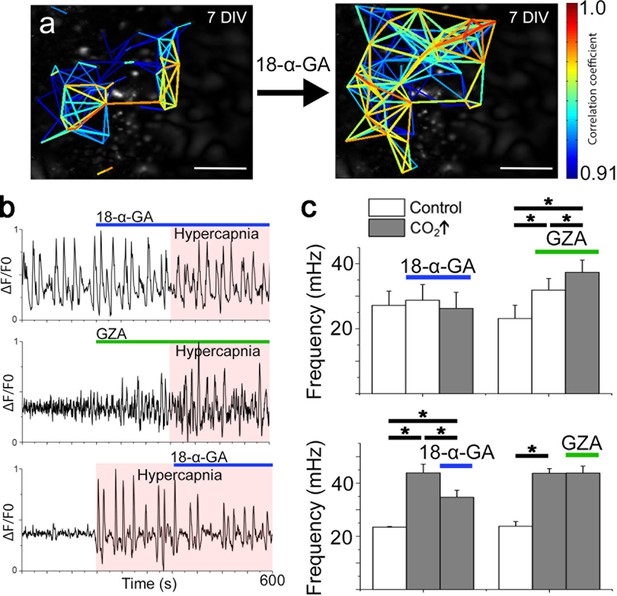
Correlated pFRG/RTN network activity is not dependent on gap junctions, but hypercapnic responses are.
Blocking gap junctions in the pFRG/RTN did not change the functional network structure of the respiratory center or alter its frequency (a and c, N=7). However, hypercapnic responses (CO2↑) were abolished when gap junctions were inhibited by 18-α-GA (b, top trace; c, left graph, N=7). GZA (a structural analog of CBX without gap junction-inhibiting properties) increased the frequency, and hypercapnia increased it further (b, middle trace; c, middle graph, N=7). An initiated hypercapnic response was attenuated but not completely reversed by 18-α-GA (b, bottom trace; c, lower graph, N=5). This dynamic was not seen after application of GZA. DIV: days in vitro. Scale bars: 200 µm. N: number of slices. Multicolored bar: color-coded correlation coefficient values. Data are presented as means ± SD. *p<0.05. Source data are available in a separate source data file.
-
Figure 10—source data 1
Hypercapnia and gap junction inhibition frequency data.
- https://doi.org/10.7554/eLife.14170.049
-
Figure 10—source data 2
Hypercapnia and gap junction inhibition network data.
- https://doi.org/10.7554/eLife.14170.050
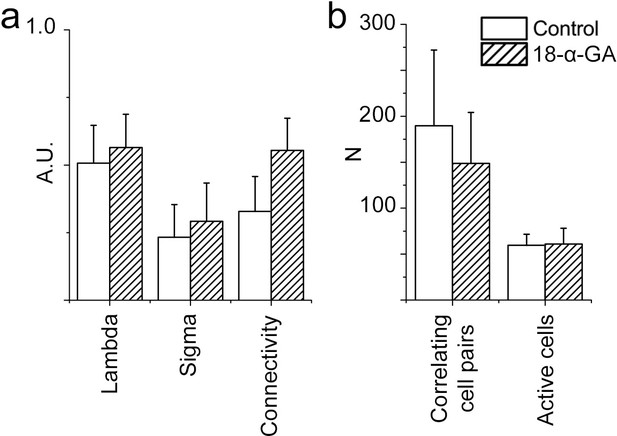
Network structure in the pFRG/RTN is not dependent on gap junctions.
Blocking gap junctions in the pFRG did not change the network parameters, correlating cell pairs, or number of active cells (N=7). Data are presented as means ± SD. N: slices. Source data are available in a separate source data file.
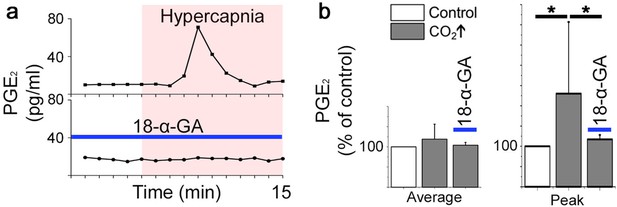
PGE2 is released during hypercapnia.
The aCSF contents exhibited an increase in microenvironmental PGE2 levels during hypercapnia in 12 out of 12 slices. Here, the PGE2 concentration of a brainstem slice culture is displayed during control and hypercapnic periods (a). When gap junctions were inhibited (18-α-GA, blue line), the PGE2 levels remained unaltered during hypercapnia (N=4). The average PGE2 level throughout the whole experiment was not affected by hypercapnia, but the peak value was higher during hypercapnia than under control conditions (b). N: number of slices. Data are presented as means ± SD. *p<0.05. Source data are available in a separate source data file.
-
Figure 11—source data 1
Hypercapnia PGE2 ELISA data.
- https://doi.org/10.7554/eLife.14170.053
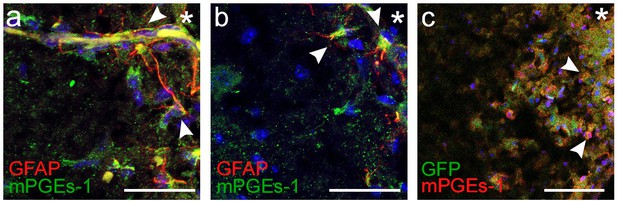
mPGEs-1 is expressed in astrocytes in the proximity of the ventral border of the pFRG.
Expression of mPGEs-1, critical for PGE2 production, was found in GFAP-expressing astrocytes (arrowheads) proximal to the ventral medullary border in acute frozen brainstem tissue. This was evident in both wild-type mice and transgenic mice with GFAP-driven expression of GFP (N=11/11 and 6/6 respectively). N: number of slices. Scale bars: 100 µm. * indicates the ventrolateral edge of the brainstem.
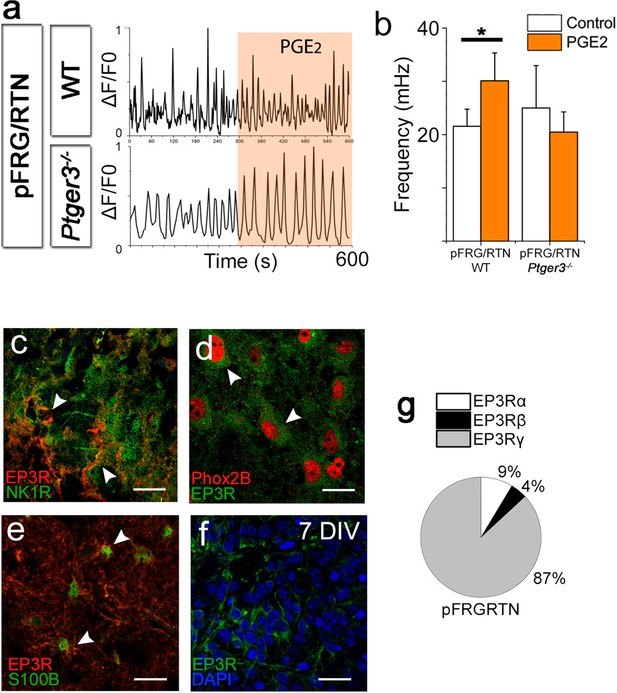
PGE2 alters respiratory network activity.
In the pFRG/RTN, PGE2 increased the frequency of respiratory (NK1R-expressing) neurons. This PGE2 effect was absent in brainstem slice cultures lacking EP3R (Ptger3-/-; a–b). EP3Rs were present in NK1R-expressing neurons in the pFRG/RTN (c, arrowheads, f) and co-localized with Phox2b (d, arrowheads). EP3Rs were also found on S100B-expressing astrocytes (e, arrowheads). Staining was performed on acutely fixed tissue (c–e) and brainstem slice cultures (f). qRT-PCR showed an abundance of the EP3Rγ (Gs-protein coupled) in the pFRG/RTN (N=7; f). N: number of slices. DIV: days in vitro. Scale bars: 100 µm. Data are presented as means ± SD. *p<0.05 Source data are available in a separate source data file.
-
Figure 12—source data 1
PGE2 frequency data pFRG/RTN.
- https://doi.org/10.7554/eLife.14170.056
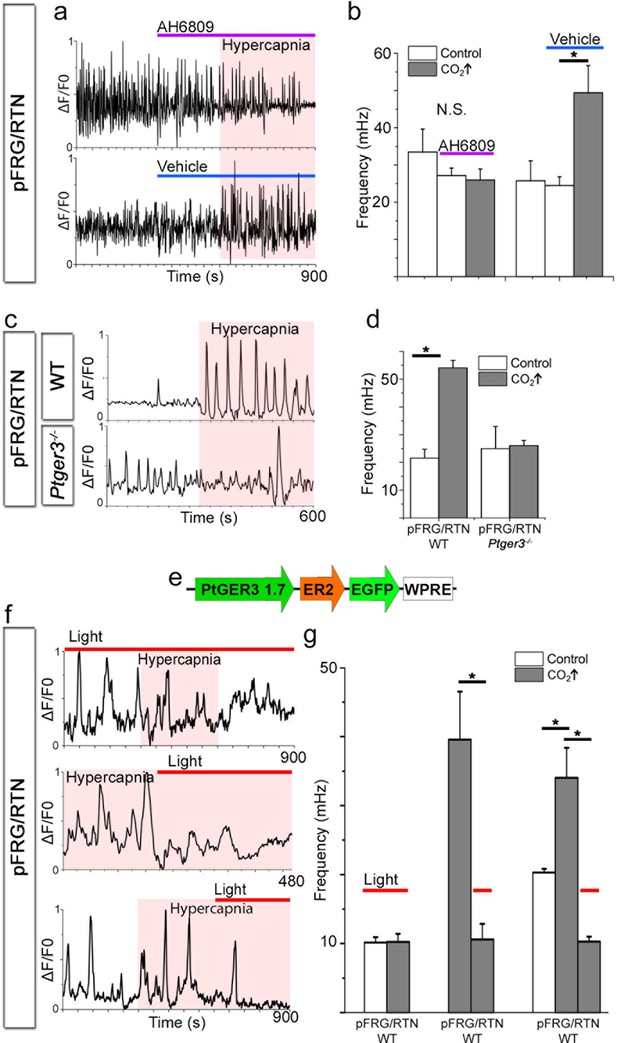
PGE2, acting through EP3R, is crucial for the hypercapnic response.
Pharmacological inhibition of EP3R by the EP receptor antagonist AH6809 inhibited the response to hypercapnia (increased pCO2[CO2↑]) in the pFRG/RTN (N=6, n=472, N.S.; a–b). The hypercapnic response was also absent in pFRG/RTN slices lacking EP3R (Ptger3−/−; N=5, n=348, N.S.; c–d). Layout of the lentivirus containing Halo57 (ER2) and eGFP genes under the control of the EP3R promoter (Ptger3) used for optogenetics (WPRE=gene enhancing element; e). During optogenetic silencing of Ptger3-expressing cells, no frequency changes were observed in response to hypercapnia (f, top trace; g, left graph). The hypercapnic response was also reversed by activating Ptger3-Halo57 (f, middle and bottom trace; g, middle and right graph). Red line: Halo57 activation in response to 625 nm light. N: slices, n: cells. Data are presented as means ± SD. *p<0.05. Source data are available in a separate source data file.
-
Figure 13—source data 1
EP antagonist data.
- https://doi.org/10.7554/eLife.14170.059
-
Figure 13—source data 2
Hypercapnia EP3R data.
- https://doi.org/10.7554/eLife.14170.060
-
Figure 13—source data 3
Optogenetics data.
- https://doi.org/10.7554/eLife.14170.061
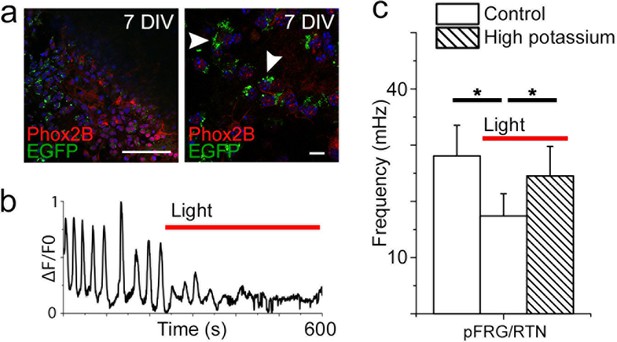
Optogenetic silencing of Ptger3-expressing cells decreases respiration-related activity.
Transduced slices show the expression of EGFP in the pFRG/RTN, localized through Phox2b staining (a, left). Phox2b-positive cells express EGFP after transduction (a, right, arrowheads). Silencing Ptger3 cells with Halo57 stimulation decreased the frequency of the entire pFRG/RTN (b). General depolarization caused by an increase in the potassium concentration to 9 mM increased the average network frequency in both the preBötC and pFRG/RTN during optogenetic inhibition (N=6; c). Red line: Halo57 activation in response to 625 nm light. N: number of slices. DIV: days in vitro. Scale bars: 100 µm (a, left), 10 µm (a, right). Data are presented as means ± SD. *p<0.05. Source data are available in a separate source data file.
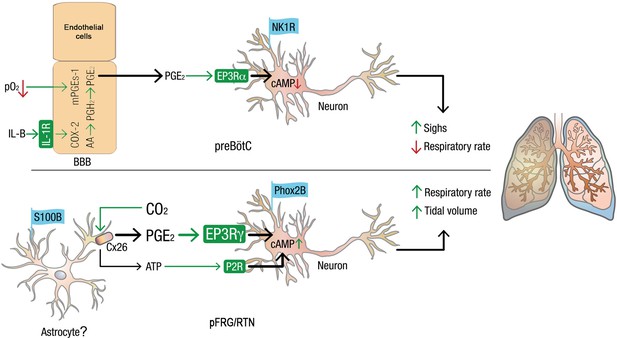
Model of how PGE2 modulates respiration and sighs in the preBötC and pFRG/RTN.
Systemic inflammation, through the proinflammatory cytokine IL-1β and hypoxia, induces the production of PGE2 in blood brain barrier (BBB) endothelial cells (Hofstetter et al., 2007).PGE2 subsequently induces respiratory depression and increases sigh activity via the inhibitory G-protein coupled receptor EP3Rα in the preBötC. In the pFRG/RTN, PGE2 plays a role in the response to elevated pCO2. CO2 directly modulates connexin 26 (Cx26) hemichannels, leading to ATP release. The results in this study suggest that Cx26 also releases PGE2, possiblyfrom mPGEs-1+ astrocytes. PGE2 increases respiratory activity via the stimulatory G-protein coupled receptor EP3Rγ on pFRG/RTN neurons. Thus, inflammation, hypoxia, and hypercapnia alter respiratory neural network and motor output and breathing activity through distinct effects of PGE2 in the pFRG/RTN and the preBötC, respectively. Chronically elevated PGE2 levels, as observed during ongoing inflammation, may decrease the central pattern generators’ ability to respond to hypoxic and hypercapnic events. In extreme cases, this decrease may have fatal consequences.
Videos
NK1R+ respiratory neurons in the preBötC are identified using TMR-SP (red dye), followed by Ca2+ oscillations visualized with Fluo-4.
After 25 s, the µ-opioid receptor agonist DAMGO (0.5 µM) is added and reduces the signaling frequency of the network. fps: frames per second.
Ca2+ oscillations visualized with Fluo-4 in the chemosensitive region pFRG/RTN.
Low network activity is increased by exposure to hypercapnia after 15 s. fps: frames per second.
Parallel display of Ca2+ oscillations visualized with Fluo-4 in the pFRG/RTN (left) and preBötC (right).
After 15 s, PGE2 (10 nM) is added. This increases the activity of the pFRG/RTN network while the preBötC activity is inhibited. fps: frames per second. For high-resolution versions of the videos, please follow this link to the Karolinska Institutet Cloud Storage system (Box): https://ki.box.com/s/abzuei0yzl4dzbn99995382va6btsq4l.
Tables
Respiratory parameters under basal conditions. Ptger3-/- mice are heavier than wild-type mice of the same age. They do not, however, differ in respiratory frequency (FR), tidal volume (VT), or minute ventilation (VE). I.c.v. injection of PGE2 increases VT and VE in wild-type mice but not Ptger3-/- mice. Respiratory frequency, tidal volume, and minute ventilation all increased during hypercapnic exposure. n: number of animals. Data are presented as mean ± SD.
Weight (g) | FR (breaths/min) | VT (µl /g) | VE (µl/g/min) | FR (breaths/min) Hypercapnia | VT (µl /g) Hypercapnia | VE (µl /g) Hypercapnia | |
---|---|---|---|---|---|---|---|
WT - vehicle n=5 | 3.7 ± 0,5# | 206 ± 28* | 9.7 ± 2.9* † | 2.0 ± 0.8*† | 259 ± 20*# | 12.3 ± 3.1*# | 3.2 ± 1.0*# |
WT - PGE2 n=8 | 3.9 ± 0.4 | 210 ± 15* | 15.1 ± 3.3† | 3.2 ± 0.8*† | 267 ± 31*# | 15.9 ± 2.6 | 4.2 ± 0.8*# |
Ptger3-/- - vehicle n=5 | 4.8 ± 0.4# | 215 ± 32* | 14.2 ± 2.4* | 3.0 ± 0.5* | 240 ± 37*# | 15.7 ± 3.1*# | 3.4 ± 1.1*# |
Ptger3-/- - PGE2 n=7 | 4.4 ± 0.3 | 211 ± 18* | 13.8 ± 2.7* | 2.9 ± 0.6* | 241 ± 30*# | 14.9 ± 2.7*# | 3.5 ± 0.4*# |
-
*p<0.05 (normocapnia vs. hypercapnia),
-
#p<0.05 (WT vs. Ptger3-/-),
-
†p<0.05 (vehicle vs. PGE2).
PGE2 and hypercapnia induce sighs. Sigh frequency does not differ between wild-type mice and Ptger3-/- mice. In wild-type mice, PGE2 increases sigh frequency. Hypercapnia also increases sigh frequency more in wild-type mice than in Ptger3-/- mice. PGE2 abolishes this increase in wild-type mice but not in Ptger3-/- mice (*p<0.05). n: number of animals. Data are presented as mean ± SD.
Sighs/min Normocapnia | Sighs/min Hypercapnia | |
---|---|---|
WT - vehicle n=5 | 0.4 ± 0.1 | 1.0 ± 0.2* |
WT - PGE2 n=8 | 0.7 ± 0.1* | 0.9 ± 0.3 |
Ptger3-/-- vehicle n=5 | 0.4 ± 0.1 | 0.7 ± 0.3* |
Ptger3-/—- PGE2 n=7 | 0.4 ± 0.1 | 0.6 ± 0.2* |
The preBötC network parameters remain unchanged for 21-DIV cultures.The results of correlation analysis for the preBötC are shown. N.S.: not significant. N: number of slices. Data are presented as mean ± SD.
preBötC | 7 DIV (N=12) | 14 DIV (N=13) | 21 DIV (N=8) | |
---|---|---|---|---|
Correlating cell pairs | 560 ± 325 | 501 ± 277 | 517 ± 327 | N.S. |
Active cells | 110 ± 40 | 100 ± 59 | 110 ± 69 | N.S. |
Correlations per active cell | 6 ± 4 | 6 ± 5 | 7 ± 6 | N.S. |
Connectivity | 0.8 ± 0.1 | 0.8 ± 0.2 | 0.8 ± 0.2 | N.S. |
Mean shortest path length (λ) | 0.7 ± 0.2 | 0.8 ± 0.2 | 0.8 ± 0.2 | N.S. |
Clustering coefficient (σ) | 2.7 ± 1.5 | 2.8 ± 1.6 | 2 ± 0.6 | N.S. |
Small-world parameter (γ) | 4.2 ± 3.0 | 3.4 ± 1.7 | 2.7 ± 1.7 | N.S. |
The pFRG/RTN network parameters remain unchanged for 21-DIV cultures. The results of correlation analysis for the pFRG/RTN are shown. Among the analyzed network parameters, only the number of active cells differed at the analyzed time points, and only between 14 and 21 DIV. N.S.: not significant. N: number of slices. Data are presented as mean ± SD.
pFRG/RTN | 7 DIV (N=12) | 14 DIV (N=11) | 21 DIV (N=6) | |
---|---|---|---|---|
Correlating cell pairs | 118 ± 69 | 61 ± 31 | 74 ± 42 | N.S. |
Active cells | 49 ± 26 | 41 ± 21* | 76 ± 19* | *p<0.05 |
Correlations per active cell | 3.1 ± 2.2 | 1.7 ± 1.1 | 1.0 ± 0.7 | N.S. |
Connectivity | 0.7 ± 0.2 | 0.6 ± 0.2 | 0.7 ± 0.2 | N.S. |
Mean shortest path length (λ) | 0.7 ± 0.2 | 0.7 ± 0.3 | 0.8 ± 0.5 | N.S. |
Clustering coefficient (σ) | 2.7 ± 1.6 | 3.0 ± 1.7 | 4.5 ± 3.6 | N.S. |
Small-world parameter (γ) | 3.6 ± 2.5 | 4.2 ± 2.6 | 3.3 ± 1.6 | N.S |
pFRG/RTN slices respond to CO2 if the EP3R is present. The average mean frequency of all cells in the network and the average mean frequency of NK1R-positive cells during the control period or during exposure to hypercapnia are shown (pCO2 = 55 mmHg, pH = 7.5). N.S.: not significant. N: number of slices, n: number of cells. Data are presented as mean ± SD.
Mean frequency (mHz) | ||||||
---|---|---|---|---|---|---|
Network | NK1R+ cells | |||||
Control | Hypercapnia | Control | Hypercapnia | |||
pFRG/RTN - WT (N=7, n=343) | 21.6 ± 3.2 | 54.1 ± 2.7* | p<0.05 | 14.4 ± 0.9 | 38.5 ± 5.5* | p<0.05 |
pFRG/RTN - Ptger3−/− (N=5, n=448) | 25.0 ± 7.9 | 26.0 ± 1.9 | N.S. | 11.4 ± 5.8 | 11.6 ± 3.8 | N.S. |
preBötC - WT (N=5, n=1737) | 16.4 ± 2.5 | 16.5 ± 1.3 | N.S. | 16.6 ± 4.6 | 15.7 ± 5.3 | N.S. |
preBötC - Ptger3−/− (N=4, n=822) | 21.1 ± 8.6 | 17.3 ± 3.8 | N.S. | 22.7 ± 5.9 | 17.8 ± 7.7 | N.S. |
PGE2 increases the frequency of pFRG/RTN neurons and decreases the frequency of preBötC neurons.The mean frequencies of NK1R-positive cells during the control period or during exposure to 10 nM PGE2 are shown. N.S.: not significant. N: number of slices, n: number of cells. Data are presented as mean ± SD.
Mean frequency (mHz) | |||
---|---|---|---|
Control | PGE2 | ||
pFRG/RTN - WT (N=5, n=343) | 13.7 ± 1.1 | 21.5 ± 2.9* | p<0.05 |
pFRG/RTN - Ptger3−/− (N=4, n=448) | 12.1 ± 2.0 | 8.5 ± 2.9 | N.S. |
preBötC - WT (N=7, n=1737) | 20.3 ± 2.2 | 8.7 ± 1.4* | p<0.05 |
preBötC - Ptger3−/− (N=5, n=822) | 22.8 ± 2.3 | 16.4 ± 1.1 | N.S. |
Silencing of Ptger3-expressing cells inhibits the response to hypercapnia.Mean frequencies of the pFRG/RTN network during the control period and during exposure to hypercapnia with and without Halo57 stimulation are shown. N.S.: not significant. N: number of slices. Data are presented as mean ± SD.
N=41 | Mean frequency (mHz) | ||
---|---|---|---|
Control | Hypercapnia | ||
Control | 22.9 ± 9.0* | 34.0 ± 4.3* | p<0.05 |
Halo57 stimulation | 9.0 ± 1.7 | 10.3 ± 1.1 | N.S. |
Primers used for qRT-PCR.
Oligo name | Sequence |
---|---|
EP3alfa forward EP3alfa reverse | GCTTCCAGCTCCACCTCCTT CATCATCTTTCCAGCTGGTCACT |
EP3 sense EP3beta anti-sense | 5′-TGACCTTTGCCTGCAACCTG-3′ 5′-GACCCAGGGAAACAGGTACT-3′ |
EP3gamma forward EP3gamma reverse | AGTTCTGCCAGGTAGCAAACG GCCTGCCCTTTCTGTCCAT |
Successful experiments behind representative images.
Figure | Panel | Number of experiments |
---|---|---|
1 | c | 25 |
2 | b c d e f g | 31 23 9 19 14 112 |
2 – S1 | 112, 23, 12 33, 23, 10 27, 12, 11 22, 15, 8 15, 8, 8 | |
2 – S2 | 5 | |
2 – S3 | a b c | 12 5 20 |
3 | a b | 5 5 |
3 – S1 | a b | 5 5 |
4 | b c d g h | 12 13 8 9 5 |
4-S2 | 840, 621, 456 | |
5 | a | 16 |
5 – S1 | a | 6 |
5 – S2 | a c | 11 5 |
6 | a b c d e f g h i j | 9 8 8 3 8 6 7 8 6 7 |
7 | a c d f | 5, 4 9 12 5, 4 |
7 – S1 | 4, 4 | |
8 | b c d e | 9 9 9 5 |
8 – S1 | 35, 22, 19 9, 9 5 | |
9 | b c d | 12 11 6 |
9 – S1 | 315, 429, 192 | |
9 – S2 | 18, 7 | |
10 | a b | 7 7, 7 and 5 |
11 | a | 12, 4 |
11 – S1 | a b c | 11 11 6 |
12 | a c d e f | 5, 4 16 44 6 12 |
13 | a c f | 6, 5 7, 5 41 |
13 – S1 | a b | 6 27 |
Statistical details for presented figures.
Figure | Test used | Exact p-value | Degrees of freedom&F/t/z/R value |
---|---|---|---|
1d | Full factorial two-way ANOVA | 0.0084 | F=0.612, DFE=21 |
1e | Full factorial two-way ANOVA | 0.0365 | F=0.284, DFE=21 |
1f | Full factorial two-way ANOVA | 0.0157 | F=0.329, DFE=21 |
1g | Full factorial two-way ANOVA | 0.0017 0.018 0.007 | F=0.547 F=0.332 F=1.618 DFE=23 |
2 – S1d | Student’s t-test | 0.35 0.45 | DF = 9, 19 |
4e | Student’s t-test | 0.63 0.76 0.91 | DF=11, 12, 8 |
4f | Student’s t-test | 0.13, 0.34, 0.68 0.21, 0.28, 0.86 0.76, 0.76, 1.00 | DF=11, 12, 8 |
4 – S2 | Student’s t-test | 0.43, 0.34, 0.12 0.11, 0.57, 0.19 | DF=1916 |
4 – S2 | Paired t-test | 0.02, 0.02, 0.04 0.03, 0.02, 0.01 | DF=1916 |
5b | Student’s t-test | 0.42, 0.51, 0.80 | DF=15 |
5c | Student’s t-test | 0.50, 0.62, 0.98 | DF=15 |
5 – S2a | Paired t-test | 0.0029 | DF=6 |
5 – S2b | Paired t-test | 0.041 | DF=10 |
5 – S2d | Paired t-test | 0.03 | DF=4 |
6k | Paired t-test | 0.03125 0.00391 0.3125 0.625 | DF=23 |
6l | Paired t-test | 0.01563 0.00781 0.28125 0.25 | DF=23 |
6i | Paired t-test | 0.492 0.331 0.390 0.390 | DF=23 |
6m | Paired t-test | 0.457 0.124 0.567 0.143 | DF=23 |
6 – S1a | Paired t-test | 0.15625 0.46094 0.0625 0.625 | DF=23 |
6 – S1b | Paired t-test | 0.15625 0.74219 0.15625 0.8125 | DF=23 |
6 – S1c | Paired t-test | 0.1246 0.07813 0.3125 0.8125 | DF=23 |
7b | Paired t-test | 0.018 0.034 0.047 0.079 0.132 0.084 0.028 0.063 0.067 0.012 0.077 0.90 | DF= 4, 3, 7 |
7g | Paired t-test | 0.95 0.51 | DF=4, 3 |
7-S1 | Paired t-test | 0.51861 0.15558 0.69733 0.51508 0.36415 0.2433 | DF=16 |
9e | Student’s t-test | 0.15, 0.51, 0.57 0.29, 0.061, 0.0081 | DF=11, 10, 7 |
9f | Student’s t-test | 0.38, 0.51, 0.75 0.59, 0.66, 0.99 0.10, 0.10, 0.95 | DF=11, 10, 7 |
9g | Paired t-test | 0.43 | DF=3 |
9h | Paired t-test | 0.0334 | DF=11 |
9i | Paired t-test | 0.0141 0.0283 0.00475 | DF=6, 5, 3 |
9 – S1 | Student’s t-test | 0.77, 0.51, 0.92 0.28, 0.07, 0.60 | DF=935 |
9 – S1 | Paired t-test | 0.04, 0.04, 0.01 0.03, 0.02, 0.01 | DF=935 |
9 – S2 | Paired t-test | 0.00507 0.5745 0.22731 0.68788 0.81018 0.66252 | DF=16 |
10c | Paired t-test | 0.53, 0.20, 0.61 0.009, 0.015, 0.041 0.023, 0.045, 0.035 0.01, 0.09, 0.14 | DF=11, 7, 7, 9 |
10 – S1a | Paired t-test | 0.153 0.0848 0.388 | DF=6 |
10 – S1b | Paired t-test | 0.59 0.43 | DF=6 |
11b | Full factorial two-way ANOVA | 0.418 0.0161 | F=0.054 F=0.97 |
12b | Paired t-test | 0.00038 0.28 | DF= 6, 4 |
13b | Paired t-test | 0.13, 0.56 0.16 0.24, 0.12, 0.012 | DF=7 |
13d | Paired t-test | 0.00046 0.87 | DF=6, 4, 4, 3 |
13g | Paired t-test | 0.4112 0.0001 | DF=40 |
13 – S1c | Paired t-test | 0.0125 0.00098 | DF=5 |
Additional files
-
Source code 1
Cross-correlation analysis.
- https://doi.org/10.7554/eLife.14170.069