MutSα maintains the mismatch repair capability by inhibiting PCNA unloading
Figures
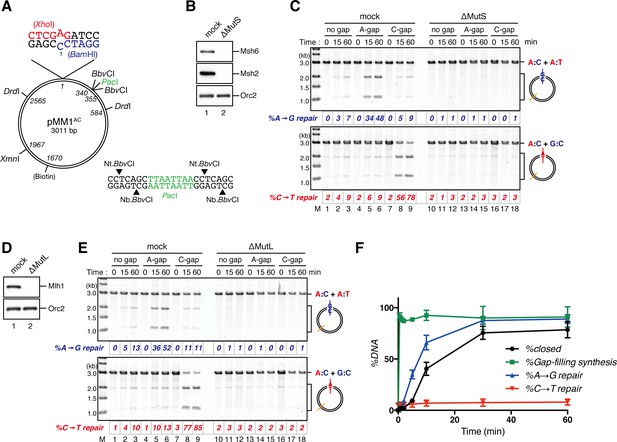
NPE promotes gap-directed MMR with efficient DNA synthesis at the gap site.
(A) DNA substrates used in this study. The 3011-base pair plasmid DNA carries an A:C mispair (pMM1AC), or an A:T base-pair (pMM1AT) at position 1. Sequences surrounding an A:C mispair are designed so that the A:T or G:C repair product forms an XhoI or BamHI cleavage site, respectively. Two BbvCI nicking restriction enzyme sites were used to introduce a 15-nt single-strand gap. A PacI site was placed within the gap. If needed, a biotin-dT modification was introduced at position 1670. (B) NPE was depleted using pre-immune antibodies (lane 1) or a mixture of Msh2 and Msh6 antibodies (lane 2). Immunoblots of the NPE samples (0.05 μl each) are shown. Orc2 served as a loading control. (C) Covalently closed (lanes 1–3 and 10–12), A-strand-gap (3’ to the mismatch)-carrying (lanes 4–6 and 13–15) or C-strand-gap (5’ to the mismatch)-carrying pMM1AC (lanes 7–9 and 16–18) were incubated in NPE described in (B), and sampled at the indicated times. DNA was purified and digested with XmnI and either BamHI (upper, A to G repair) or XhoI (lower, C to T repair). %repair was calculated based on the percentage of XhoI or BamHI sensitive DNA molecules. (D) NPE was depleted using pre-immune antibodies (lane 1) or Mlh1 antibodies (lane 2). Immunoblots of the NPE samples (0.05 μl each) are shown. (E) The MMR reaction in NPE described in (D). DNA was digested with XmnI and either BamHI (upper, A to G repair) or XhoI (lower, C to T repair). (F) Percentages of DNA synthesis at the gap, estimated by PacI sensitivity (%Gap-filling synthesis), A to G repair (%A→G repair), C to T repair (%C→T repair) and closed circular molecules (%closed; Figure 1—figure supplement 5), calculated from two independent experiments including the one described in Figure 1—figure supplement 6, were plotted onto a graph. To calculate the %Gap-filling synthesis, DNA was digested with XmnI and PacI. The mean values were connected by lines. Error bars: ± 1 standard deviation (SD).
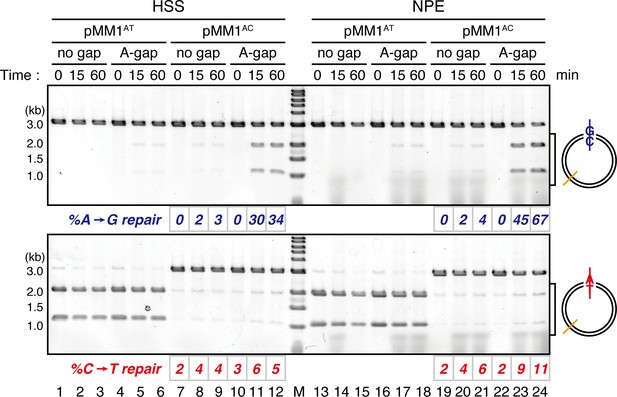
Comparison of the MMR efficiencies between in crude Xenopus egg extracts and in NPE.
Homoduplex pMM1AT carrying no strand break (lanes 1–3 and 13–15) or A-strand gap (lanes 4–6 and 16–18), or mismatch-containing pMM1AC carrying no strand break (lanes 7–9 and 19–21), or A-strand gap (lanes 10–12 and 22–24) was incubated in High speed supernatants (HSS; lanes 1–12) or NPE (lanes 13–24), and sampled at the indicated times. %repair was calculated as described in Figure 1C. Gap-directed A to G MMR was more efficient in NPE (67%) than in HSS (34%), indicating that NPE has higher MMR activity than HSS.
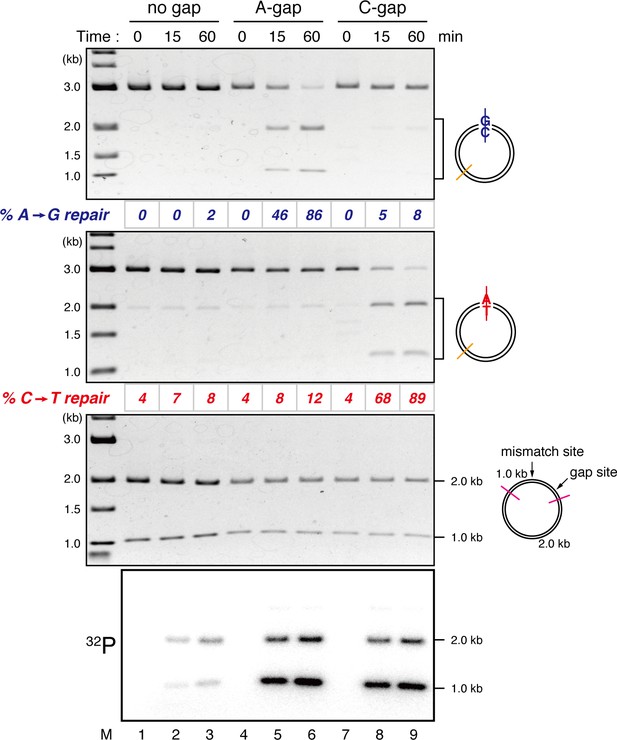
Detection of tracts of DNA repair synthesis during gap-directed MMR in NPE.
Covalently closed (lanes 1–3), A-strand-gap-carrying (lanes 4–6) or C-strand-gap-carrying pMM1AC (lanes 7–9) was incubated in NPE, and sampled at the indicated times. DNA was purified and digested with XmnI and either BamHI (upper, A to G repair) or XhoI (middle, C to T repair). To analyze the incorporation of radioactivity, DNA was digested with DrdI (bottom). α-[32P]-dCTP was preferentially incorporated into the 1 kb fragment corresponding to the shorter path between the gap and the mismatch in both 5’-gap- and 3’-gap-directed MMR.
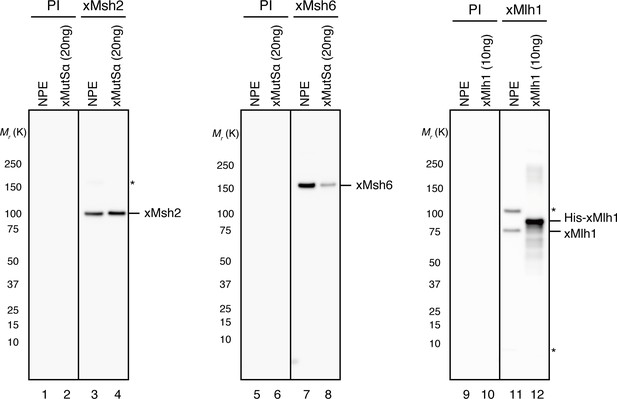
Characterization of xMsh2, xMsh6 and xMlh1 sera.
0.1 μl of NPE and the indicated amounts of recombinant proteins were separated using 4–15% SDS-PAGE and transferred onto PVDF membranes.
Each membrane strip was probed with either the indicated antiserum or pre-immune serum (PI) from the same rabbit. The same exposure sets with PI and antisera are presented. Either xMsh2 (Mr = 1.04 × 105) or xMsh6 (Mr = 1.49 × 105) was detected as nearly a single band in NPE. Two major bands were detected with Mlh1 antibodies, and we concluded that the more quickly migrating band is xMlh1, because its mobility on SDS-PAGE was closer to the expected molecular weight of Mlh1 (Mr = 8.41 × 104), it migrated slightly faster than recombinant xMlh1 tagged with 6×His-epitope through a 12 amino acid linker, and this band was specifically immunoprecipitated by xMlh1 antibodies (see Figure 1D). (*) indicates cross-reacting band.
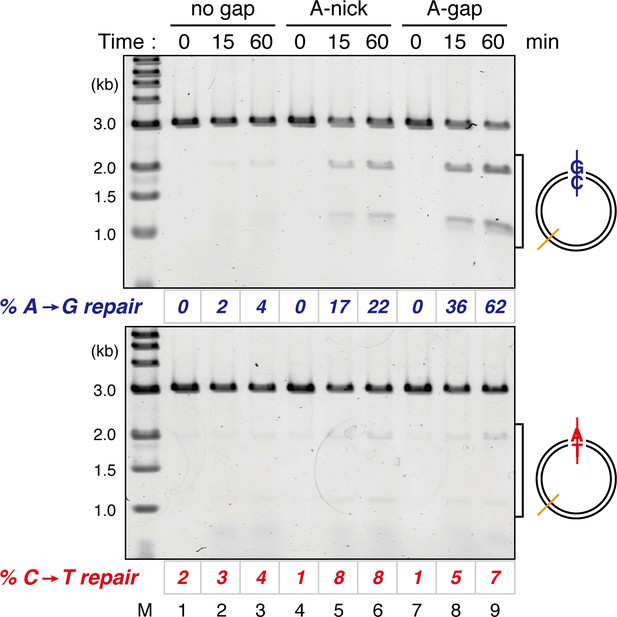
Comparison of the MMR efficiencies between nick-carrying and gap-carrying substrates.
Mismatch-containing pMM1AC carrying no strand break (lanes 1–3), A-strand nick carrying pMM2AC (lanes 4–6), or A-strand gap carrying pMM1AC (lanes 7–9) was incubated in NPE, and sampled at the indicated times. %repair was calculated as described in Figure 1C. Gap-directed A to G MMR (62%) was more efficient than nick-directed A to G MMR (22%).
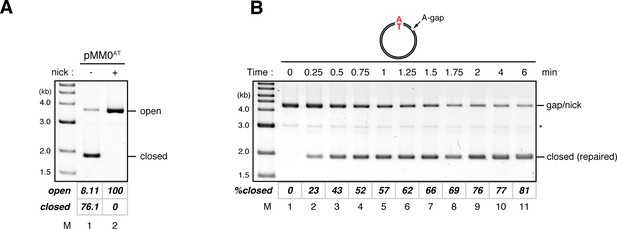
Kinetics of gap filling in NPE in the absence of a mismatch.
(A) Calculation of the relative coefficient of SYBR Gold fluorescence for closed-form DNA over open-form DNA. A reaction mixture containing in vitro synthesized pMM0AT (a pMM1 derivative that carries no BbvCI nicking site) was split into two aliquots and incubated in the absence (lane 1) or presence (lane 2) of the nicking endonuclease Nb.BtsI, and separated by ethidium bromide containing agarose gel electrophoresis. The gel was stained with SYBR Gold nucleic acid stain and scanned with Typhoon FLA9000 using SYBR Gold specific setting (a 473 nm excitation laser and a 510 nm Long pass filter). A representative result is shown. The relative fluorescence coefficient for closed-form DNA over open-form DNA (c), which reflects difference in the saturating amount of SYBR Gold stain between open and closed form DNA, was calculated by the following formula.
The calculated c value was 1.15 (standard deviation = 0.06, n = 10). Percentage of closed circular molecules (%closed) was calculated by the following formula.
(B) Kinetics of the gap-filling reaction in NPE on a homoduplex DNA. Gap-carrying homoduplex pMM1AT was incubated in NPE and sampled at the indicated times. %closed was calculated as described in (A). (*) indicates linearized fragments produced by the nicking endonuclease.
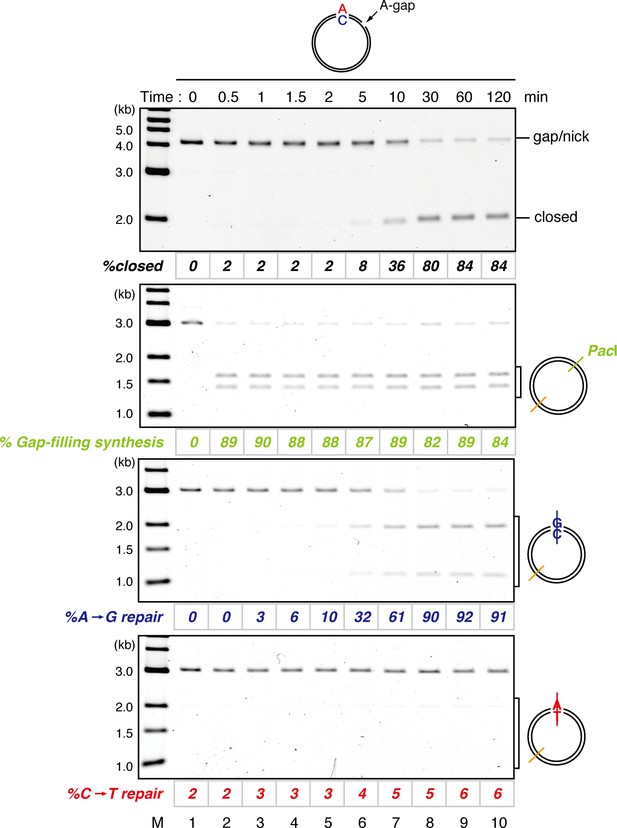
Kinetics of gap filling and MMR on a mismatch-carrying DNA in NPE.
A-strand-gap-carrying pMM1AC was incubated in NPE and sampled at the indicated times. %closed,% Gap-filling synthesis and %repair were calculated as described in Figure 1—figure supplement 5, Figure 1F, and C, respectively.
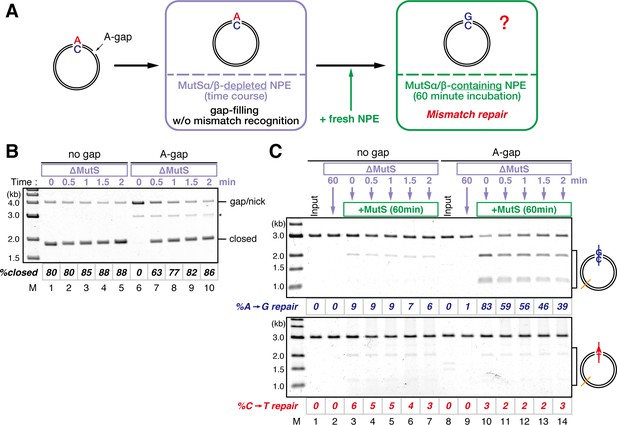
Strand memory derived from a gap directs MMR after the completion of gap filling.
(A) Schematic diagram of the stepwise incubation assay. pMM1AC carrying a 15-nt gap was incubated in a MutS-depleted NPE to fill the gap without recognizing the mismatch. Subsequently, fresh NPE was added to an aliquot of the reaction to initiate MMR by supplying MutSα/β. After 60 min incubation, DNA was purified and the direction and the efficiency of repair were examined. (B) Kinetics of the gap-filling reaction in MutS-depleted NPE. Covalently closed (lanes 1–5) or A-strand-gap-carrying pMM1AC (lanes 6–10) was incubated in MutS-depleted NPE and sampled at the indicated times. (*) indicates linear DNA produced by contaminating endonuclease activity in Nt.BbvCI. These linear molecules were excluded from the calculation of %closed. (C) Strand-specific MMR reaction after supplying MutSα/β. Aliquots were sampled at the indicated times, mixed with fresh NPE, and incubated for an additional 60 min. No repair was observed when the second NPE was omitted (lanes 2 and 9).
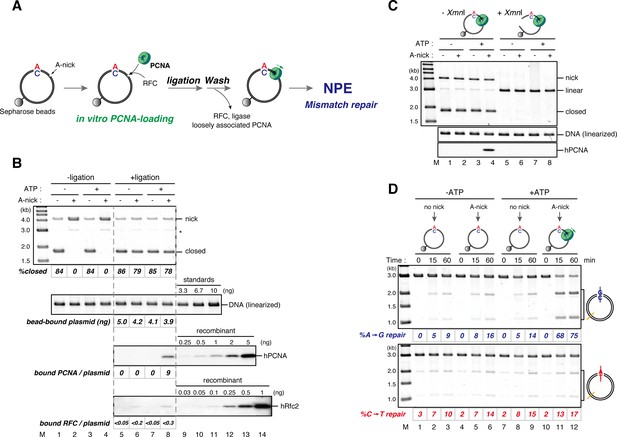
DNA-bound PCNA bypasses the requirement of a gap for strand-specific MMR in NPE.
(A) Schematic diagram of the assay. Singly biotinylated pMM2AC (a pMM1 derivative carrying only one BbvCI site) carrying a nick was bound to Sepharose beads and incubated with recombinant hPCNA and hRFC. The nick was then ligated, and the complex was washed with a buffer containing 1 M KCl. The hPCNA-DNA complex was incubated in NPE to test whether MMR occurs. (B) In vitro PCNA-loading reaction. Untreated DNA (top), linearized DNA (middle, by XmnI), and quantitative immunoblottings for hPCNA and hRfc2 (bottom) of samples from the in vitro PCNA-loading assay using covalently closed, and A-strand-nick-carrying pMM2AC are presented. (*) indicates linear DNA, which was excluded from the calculation of %closed. (C) The hPCNA-DNA complexes described in (B) were split into two portions to test whether PCNA encircles DNA (C) and whether MMR occurs upon incubation in NPE (D). The one portion was treated with either control buffer, or buffer containing XmnI whose cleavage site is located 1382 bp away from the PCNA entry point. DNA from the reaction (top), linearized DNA (middle, by XmnI), and a hPCNA immunoblot (bottom) are shown. Since nick-carrying molecules were accumulated during incubation, the level of closed-circular molecules was lower than the original substrates shown in (B). (D) The other potion of the hPCNA-DNA complexes described in (B) was incubated in NPE. The MMR efficiencies were calculated as described in Figure 1C.
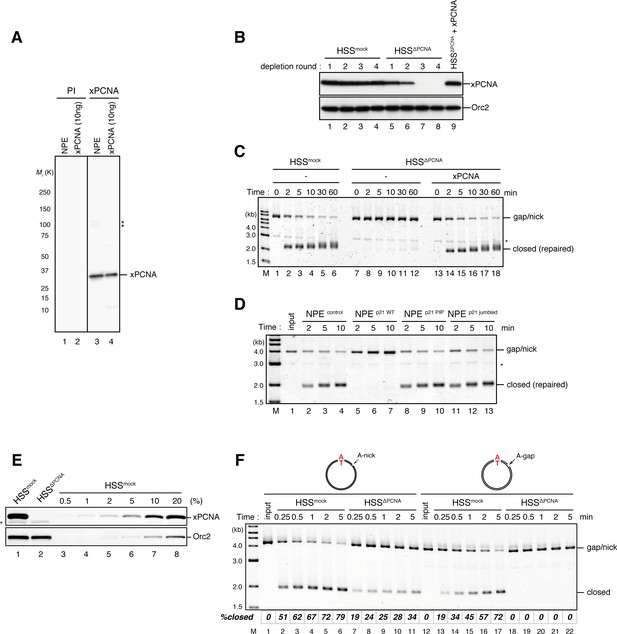
Requirement of PCNA for gap-filling and nick-ligation reactions in Xenopus egg extracts.
(A) Characterization of xPCNA sera. 0.1 μl of NPE and 10 ng of recombinant xPCNA were separated using 4–15% SDS-PAGE and transferred onto PVDF membranes. Each membrane strip was probed with either the xPCNA or pre-immune serum (PI) from the same rabbit. The same exposure sets of PI and xPCNA antibodies are shown. xPCNA (Mr = 2.88 × 104 as a monomer) was detected as nearly a single band in NPE. (*) indicates cross-reacting band. (B) The efficiency of PCNA depletion from HSS. HSS was depleted with pre-immune (lanes 1–4) or xPCNA antibodies (lanes 5–9), and supplemented with either control buffer (lanes 4 and 8) or 0.5 μM recombinant xPCNA (lane 9). HSS (0.5 μl) from each depletion rounds was separated by SDS-PAGE and probed with the indicated antibodies. Orc2 served as a loading control. (C) The gap-filling reaction in PCNA-depleted HSS. Gap-carrying homoduplex pMM1AT plasmids were incubated in PCNA-depleted HSS described in (B) and sampled at the indicated times. The efficiency of the gap-filling reaction was analyzed using ethidium bromide containing agarose gel electrophoresis. Depletion of PCNA inhibited appearance of closed-form plasmids even after 60 min (lane 12), indicating that PCNA is essential for either DNA synthesis at the gap site, flap-processing, or ligation at the gap in HSS. (*) indicates linearized fragments produced by the nicking endonuclease. (D) The gap-filling reaction in p21-peptide supplemented NPE. Because PCNA-depletion from NPE was not possible due to its high concentration, p21 peptides (Mattock et al. 2001) were used to inhibit PCNA function in NPE. Gap-carrying homoduplex pMM1AT plasmids were incubated in NPE supplemented with either control buffer (lanes 2–4), 1 mg/ml wildtype (lanes 5–7), PIP mutant (lanes 8–10) or jumbled p21 peptide (lanes 11–13), and sampled at the indicated times. The efficiency of gap filling was analyzed using ethidium bromide containing agarose gel electrophoresis. The wildtype but not the PIP mutant nor jumbled p21 peptide inhibited appearance of closed-form plasmids, suggesting that PCNA is required for either DNA synthesis at the gap site, flap-processing, or ligation at the gap in NPE. (*) indicates linearized fragments produced by the nicking endonuclease. (E) The depletion efficiency of PCNA from HSS. HSS was depleted using pre-immune (lane 1) or xPCNA antibodies (lane 2). Immunoblots of the HSS samples (0.5 μl each) are shown. Orc2 served as a loading control. (*) indicates cross-reacting band. (F) Comparison of nick ligation and gap filling in PCNA-depleted HSS. Nick-carrying homoduplex pMM2AT (lanes 1–11) or gap-carrying homoduplex pMM1AT (lanes 12–22) was incubated in either mock-treated or PCNA-depleted HSS described in (E), and sampled at the indicated times. The efficiencies of accumulation of closed-circular molecules were analyzed using ethidium bromide containing agarose gel electrophoresis. Although gap filling was completely inhibited by PCNA-depletion, nick ligation was only partially inhibited, indicating that PCNA is only partially required for nick ligation in HSS.
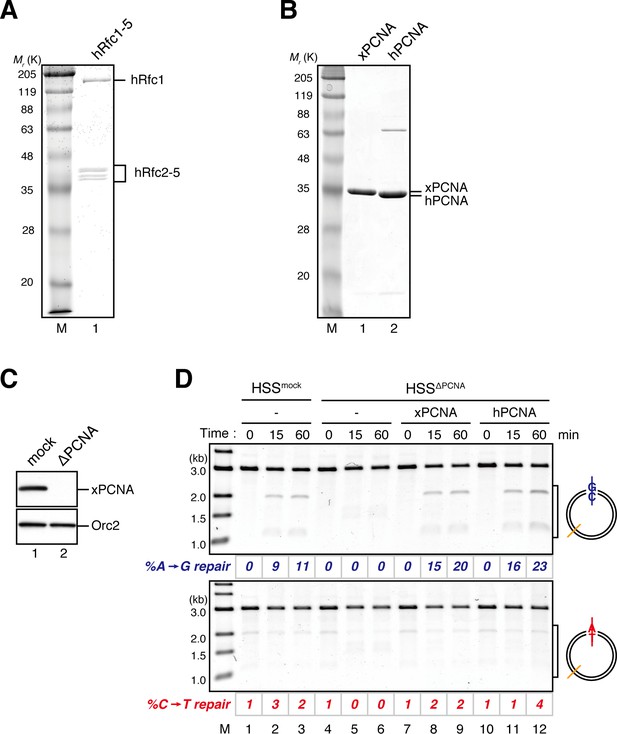
Characterization of recombinant hRFC and hPCNA.
(A) 300 ng of recombinant hRfc1-5 purified from baculovirus-infected High Five insect cells was separated using SDS-PAGE and stained with Coomassie brilliant blue R-250. (B) 1 μg each of recombinant xPCNA and hPCNA purified from E. coli was separated using SDS-PAGE and stained with Coomassie brilliant blue R-250. (C) The depletion efficiency of PCNA from HSS. HSS was depleted using pre-immune (lane 1) or xPCNA antibodies (lane 2). The HSS samples were separated using SDS-PAGE and probed with the indicated antibodies. Orc2 served as a loading control. (D) hPCNA can replace xPCNA in the MMR reaction in HSS. A-strand-gap-carrying pMM1AC plasmids were incubated in HSS described in (C) supplemented with either control buffer (lanes 1–6), 0.5 μM of xPCNA (lanes 7–9) or hPCNA (lanes 10–12) and sampled at the indicated times. %repair was calculated as described in Figure 1C. hPCNA, as well as xPCNA, restored the A to G MMR in xPCNA-depleted HSS, indicating that hPCNA can functionally replace xPCNA in MMR. Note that the overall MMR efficiency was weakened because of the rather harsh xPCNA depletion condition (4 round depletion).
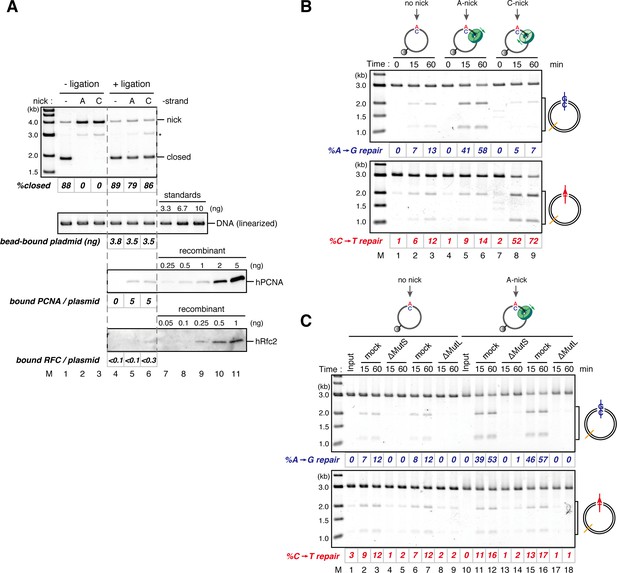
The orientation of PCNA loading determines strand specificity of MMR in NPE.
(A) In vitro PCNA-loading reaction with pMM2AC carrying no nick, an A-strand nick or a C-strand nick. Untreated DNA (top), linearized DNA (middle, by XmnI), and quantitative immunoblots (bottom) are presented. (B) The hPCNA-DNA complexes described in (A) were incubated in NPE. (C) The hPCNA-DNA complexes (Figure 4—figure supplement 2) were incubated in mock-treated, MutS-depleted or MutL-depleted NPE described in Figure 4—figure supplement 2.
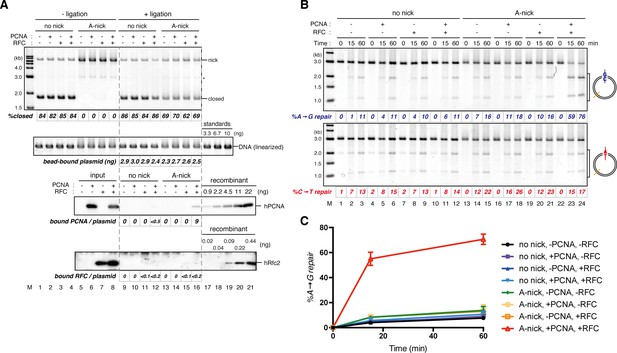
Requirement of hPCNA and hRFC in PCNA-directed MMR in NPE.
(A) Singly biotinylated pMM2AC, either covalently closed (lanes 1–4 and 9–12) or A-strand nick carrying (lanes 5–8 and 13–16), was bound to Sepharose beads and incubated with either hPCNA (lanes 2, 6, 10 and 14), hRFC (lanes 3, 7, 11 and 15), or both (lanes 4, 8, 12, and 16). Untreated DNA (top), linearized DNA (middle, by XmnI), and quantitative immunoblottings of hPCNA and hRfc2 (bottom) are shown. (B) The hPCNA-DNA complexes described in (A) were incubated in NPE. %repair was calculated as described in Figure 1C. Strand-specific A to G MMR was induced only when both hPCNA and hRFC were present in the loading reaction (lanes 22–24). (C) The efficiencies of MMR were calculated from three independent experiments including the one shown in (B) and plotted onto a graph. Error bars: ± 1 SD.
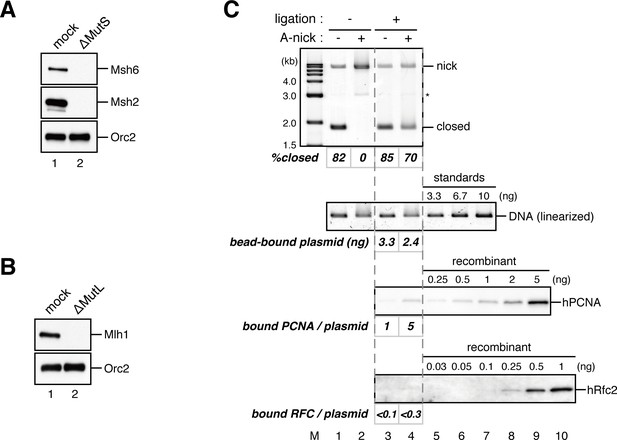
Requirement of the MutS and MutL complexes in PCNA-directed MMR in NPE.
(A, B) Depletion of the MutSα/β (A) or the MutLα/β/γ complexes (B) from NPE. NPE was depleted using pre-immune antibodies (lane 1), a mixture of Msh2 and Msh6 (A; lane 2), or Mlh1 antibodies (B; lane 2). The NPE samples were separated using SDS-PAGE and probed with the indicated antibodies. Orc2 served as a loading control. (C) In vitro PCNA-loading reaction. Untreated DNA (top), linearized DNA (middle; by XmnI), and quantitative immunoblottings of hPCNA and hRfc2 (bottom) from in vitro PCNA-loading assays used in Figure 4C are shown.
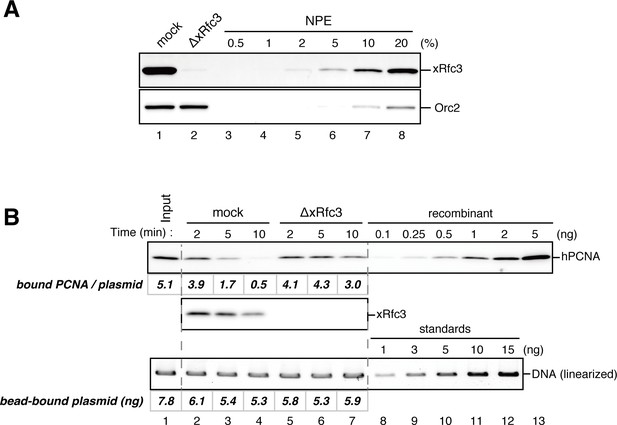
Rfc3 is required for the unloading of PCNA from closed-circular DNA.
(A) Depletion efficiency of xRfc3 from NPE. The depletion efficiency was estimated to be >98%. (B) hPCNA loaded onto immobilized pMM2AT (carrying no mismatch) was incubated in NPE described in (A). The efficiency of nick ligation in the PCNA loading reaction was estimated to be ~89%.
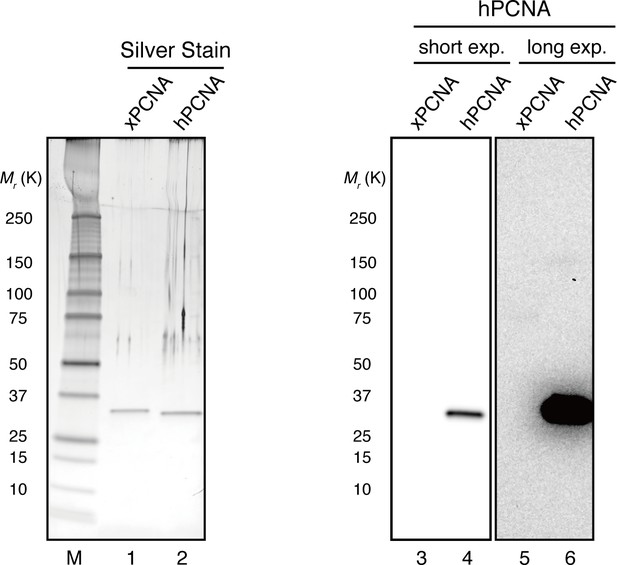
Specific detection of hPCNA by a monoclonal antibody.
The hPCNA monoclonal antibody used in this study does not detect xPCNA. 10 ng each of recombinant xPCNA and hPCNA were separated using 4–15% SDS-PAGE. Recombinant proteins were visualized using silver nitrate or transferred onto PVDF membranes. The membrane strip was probed with hPCNA antibody. hPCNA monoclonal antibodies do not detect recombinant xPCNA even when the membrane was exposed for longer periods (lanes 3–6).
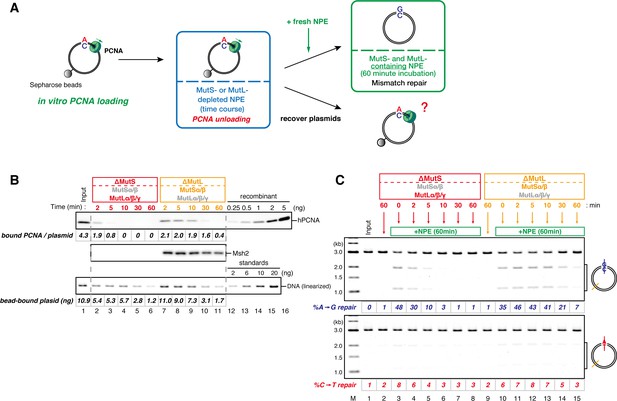
The MutS complexes inhibit Rfc3-dependent PCNA unloading in NPE.
(A) Schematic diagram of the stepwise incubation assay. After the in vitro PCNA-loading reaction, hPCNA-DNA complexes were incubated in either MutS- or MutL-depleted NPE. The samples were split into two portions, fresh NPE was added to one portion to measure MMR, and DNA-bound proteins were recovered from the other portion to quantify hPCNA. (B) hPCNA loaded onto immobilized pMM2AC carrying an A-strand nick was incubated in NPE depleted of either MutSα/β or MutLα/β/γ. The efficiency of nick ligation in the PCNA loading reaction was estimated to be ~72%. The amounts of DNA-bound hPCNA are shown. See Figure 6—figure supplement 1 for the depletion efficiencies (MutS: >98%, MutL: >98%). (C) Kinetics of the PCNA-directed MMR capability in the presence or absence of MutSα/β. Aliquots were sampled from the reaction described in (B) and mixed with fresh NPE.
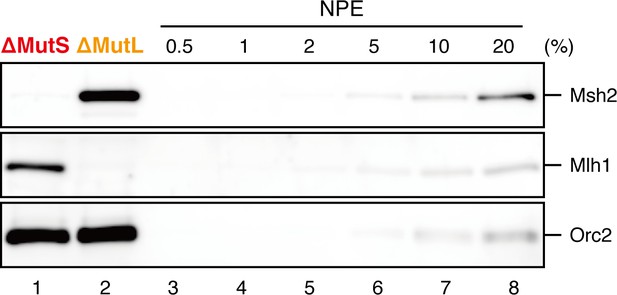
Depletion efficiencies of Msh2 and Mlh1 from NPE.
NPE was depleted using a mixture of Msh2 and Msh6 antibodies (lane 1) or Mlh1 antibodies (lane 2). The NPE samples and a dilution series of NPE were separated using SDS-PAGE and probed with the indicated antibodies for the depletion efficiencies, which we estimated that >98% for both Msh2 and Mlh1. Orc2 served as a loading control.
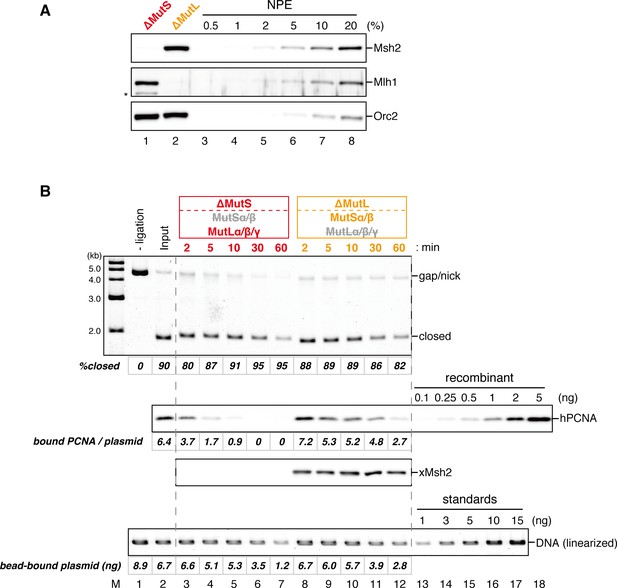
Effect of MutS- and MutL-depletion on the level of closed-circular DNA molecules.
(A) NPE was depleted using a mixture of Msh2 and Msh6 antibodies (lane 1) or Mlh1 antibodies (lane 2). The NPE samples and a dilution series of NPE were separated using SDS-PAGE and probed with the indicated antibodies for the depletion efficiencies, which we estimated >98% for both Msh2 and Mlh1. Orc2 served as a loading control. (*) indicates cross-reacting band. (B) After the in vitro PCNA-loading reaction, the hPCNA-DNA complex (lane 2) was incubated in either MutS- or MutL-depleted NPE described in (A). Untreated DNA (top), DNA-bound PCNA and Msh2 (middle), and linearized DNA (bottom, by XmnI) are shown. Depletion of either MutS or MutL complex did not significantly affect the level of closed-circular molecules.
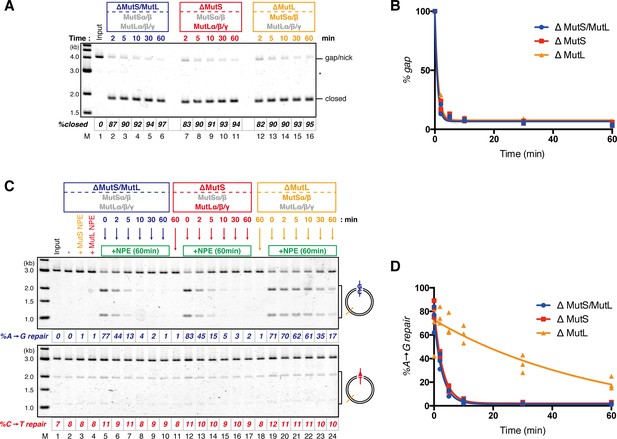
The MutS complexes retain strand memory derived from a gap in NPE.
(A) The gap-filling reaction of A-strand-gap-carrying pMM1AC in NPE described in Figure 7—figure supplement 1. (B) The efficiencies of the gap-filling reaction were calculated from three independent experiments including the one described in (A) and plotted on a graph. The lines represent one-phase decay fitting of the data. See Table 1 for the fitting parameters. (C) Strand-specific MMR reaction after supplying fresh NPE. (D) The efficiencies of MMR were calculated and presented as described in (B).
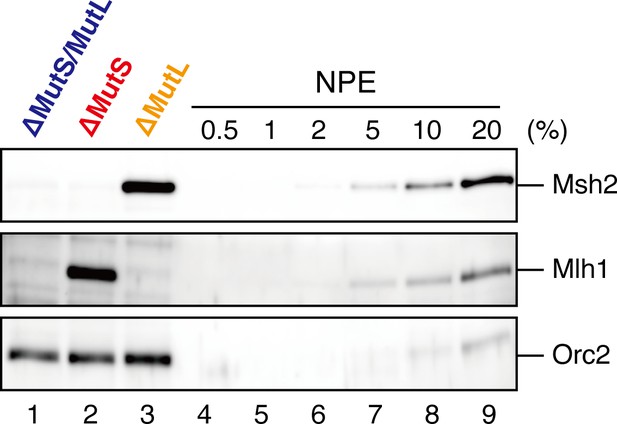
Depletion efficiencies of Msh2 and Mlh1 from NPE.
NPE was depleted using a mixture of Msh2, Msh6, and Mlh1 antibodies (lane 1), a mixture of Msh2 and Msh6 antibodies (lane 2) or Mlh1 antibodies (lane 3). The depletion efficiencies were estimated to be >99% for both Msh2 and Mlh1.
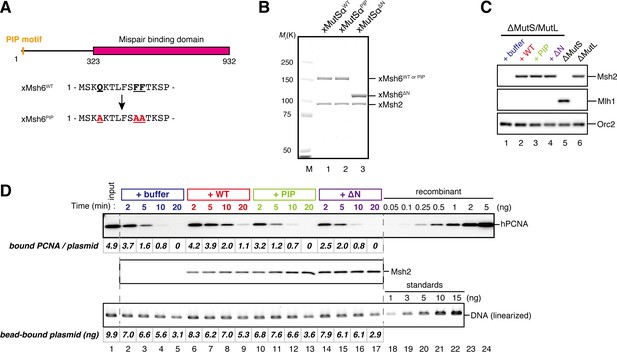
The PIP-motif of xMutSα is important for retention of PCNA.
(A) The primary structure of Msh6 and the mutation sites in the PIP motif are presented. (B) 1 μg each of purified xMutSα complexes were separated by SDS-PAGE and stained with Coomassie brilliant blue R-250. (C) NPE was depleted of both MutS and MutL (lanes 1–4), MutS (lane 5), or MutL (lane 6) and supplemented with either control buffer, 630 nM of xMutSαWT, xMutSαPIP, or xMutSαΔN. Immunoblots of each NPE are shown. (D) hPCNA loaded onto immobilized pMM2AC carrying an A-strand nick was incubated in NPE described in (C). The efficiency of nick ligation in the PCNA loading reaction was estimated to be ~75%.
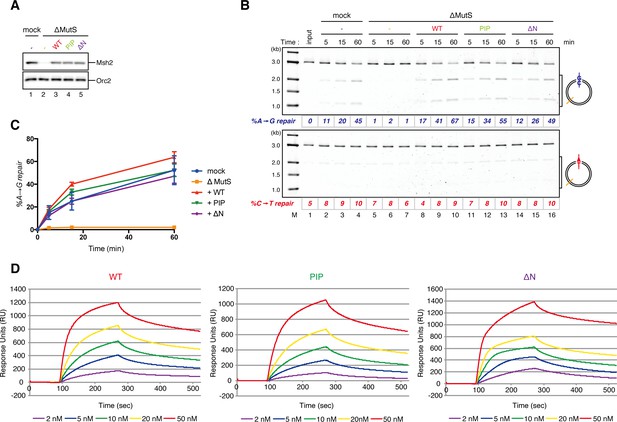
Requirement of the PIP-containing N-terminal domain of MutSα in MMR and mismatch binding.
(A-C) Effect of the MutSα mutant complexes on gap-directed MMR in NPE. (A) NPE was depleted with pre-immune antibodies (lane 1) or a mixture of Msh2 and Msh6 antibodies (lanes 2–5), and supplemented with either control buffer (lanes 1 and 2), 630 nM of xMutSαWT (lane 3), xMutSαPIP (lane 4) or xMutSαΔN (lane 5). The NPE samples were separated by SDS-PAGE and probed with the indicated antibodies. Orc2 served as a loading control. (B) The MMR assay in NPE. A-strand-gap-carrying pMM1AC were incubated in NPE described in (A) and sampled at the indicated times. %repair was calculated as described in Figure 1C. The xMutSαPIP and xMutSαΔN complexes nearly fully restored MMR in the MutS depleted NPE (lanes 11–16). (C) The efficiencies of MMR were calculated from three independent experiments including the one presented in (B) and plotted onto a graph. Error bars: ± 1 SD. (D) Dissociation constants of the MutSα mutants on mismatch-carrying DNA. The xMutSαWT, xMutSαPIP and xMutSαΔN complexes were flowed over a sensor chip coated with mismatch-carrying oligo DNA. The kinetics of association and dissociation of xMutSα were monitored by surface plasmon resonance using BIACORE 3000 (GE Healthcare, Little Chalfont, UK). The KD of the xMutSαWT, xMutSαPIP and xMutSαΔN for the mismatched DNA were 2.2 nM, 4.4 nM and 3.6 nM, respectively (n = 2).
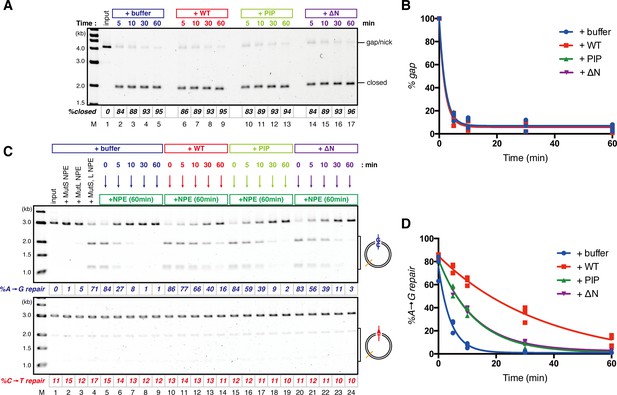
The PIP-motif of xMutSα is important for retention of the MMR capability derived from a gap.
(A) Kinetics of the gap-filling reaction in MutS/MutL-depleted NPE containing 630 nM of xMutSαWT, xMutSαPIP, or xMutSαΔN. (B) The efficiencies of the gap-filling reaction were calculated from three independent experiments including the one shown in (A) and plotted onto a graph. Lines: one-phase decay fitting. See Table 2 for the fitting parameters. (C) Kinetics of MMR after supplying fresh NPE to the reaction described in (A). Addition of neither MutS-depleted nor MutL-depleted NPE alone, but both restored MMR in MutS/MutL-depleted NPE (lanes 2–4), showing both MutSα/β and MutLα/β/γ were functionally depleted. (D) The efficiencies of MMR were calculated and presented as described in (B).
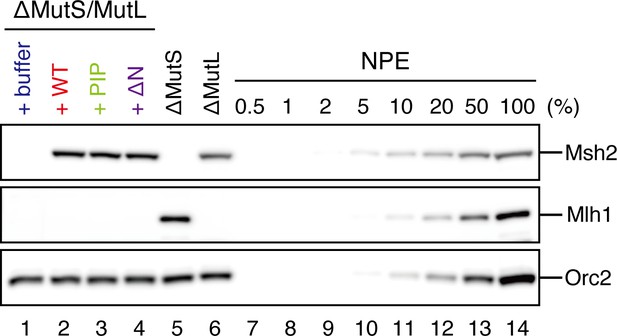
Depletion efficiencies of Msh2 and Mlh1 from NPE.
NPE was depleted using a mixture of Msh2, Msh6, and Mlh1 antibodies (lanes 1–4), a mixture of Msh2 and Msh6 antibodies (lane 5) or Mlh1 antibodies (lane 6) and supplemented with either control buffer, 630 nM of xMutSαWT, xMutSαPIP, or xMutSαΔN. The depletion efficiencies were estimated to be >98% for both Msh2 and Mlh1.
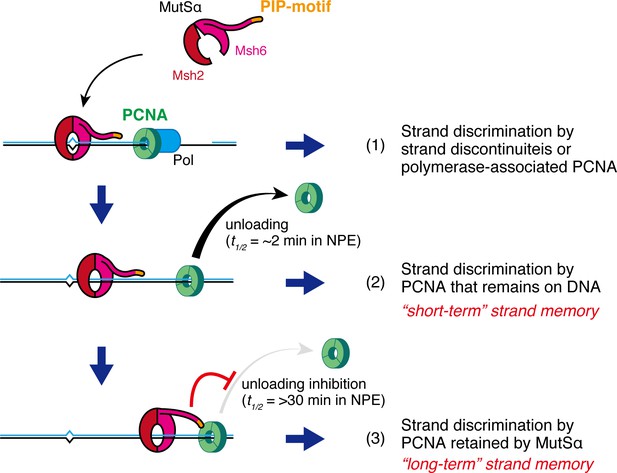
Two modes of strand memory maintain the MMR capability.
Three mechanisms, including two 'strand-memory' mechanisms, may ensure eukaryotic MMR. (1) During ongoing DNA synthesis, strand discontinuities or polymerase-associated PCNA are directly used for strand discrimination. (2) After sealing of local strand discontinuities, PCNA that remains on DNA provides strand information until they are unloaded (t1/2 = ~2 min in NPE; 'short-term' strand memory). (3) MutSα inhibits PCNA unloading to maintain the strand discrimination capability, largely through the PIP-motif on the NTR of Msh6 (t1/2 = >30 min in NPE; 'long-term' strand memory). Black and blue lines represent the template and the newly synthesized DNA strands, respectively.
Tables
Fitting parameters for the gap-derived strand memory experiment.
%gap [95% Confidence Interval] | %A→G repair [95% Confidence Interval] | ||||||
---|---|---|---|---|---|---|---|
t1/2 (min) | %gap at 0 min | R2 | t1/2 (min) | %repair at 0 min | R2 | ||
ΔMutS/MutL | 0.60 [0.51−0.73] | 100 [96.7−103.3] | 0.99 | 1.87 [1.52−2.45] | 74.8 [68.6−80.7] | 0.97 | |
ΔMutS | 0.68 [0.58−0.81] | 100 [96.4−103.5] | 0.99 | 2.00 [1.76−2.31] | 82.8 [79.0−86.6] | 0.99 | |
ΔMutL | 0.75 [0.66−0.88] | 100 [96.5−103.5] | 0.99 | 39.6 [12.3− +∞] | 72.8 [63.6−81.9] | 0.80 |
-
Parameters for the one-phase exponential decay fitting of the data described in Figure 7B and D are presented. %gap: percentage of remaining gaps (100 - %closed), t1/2: half-life, R2: coefficient of determination. n = 3. Curve fitting was carried out using the GraphPad Prism 6 software (GraphPad Software, CA, USA)
Fitting parameters for the gap-derived strand memory experiment with MutSα mutants
%gap [95% Confidence Interval] | %A→G repair [95% Confidence Interval] | |||||
---|---|---|---|---|---|---|
t1/2 (min) | %gap at 0 min | R2 | t1/2 (min) | %repair at 0 min | R2 | |
+buffer | 1.44 [0.51−0.73] | 100 [95.2−104.7] | 0.99 | 2.94 [2.32−3.98] | 75.7 [69.2−82.2] | 0.97 |
+xMutSαWT | 1.35 [0.58−0.81] | 100 [95.1−104.9] | 0.99 | 25.5 [17.6−46.1] | 84.8 [80.5−89.2] | 0.98 |
+xMutSαPIP | 1.48 [1.18−2.00] | 100 [95.4−104.6] | 0.99 | 12.5 [10.9−14.7] | 81.9 [78.5−85.4] | 0.99 |
+xMutSαΔN | 1.43 [1.14−1.93] | 100 [95.6−104.4] | 0.99 | 12.3 [11.3−13.6] | 81.9 [79.8−84.0] | 1.00 |
-
Parameters for the one-phase exponential decay fitting of the data described in Figure 9B and D are presented. %gap: percentage of remaining gaps (100 - %closed), t1/2: half-life, R2: coefficient of determination. n = 3.