Microtubules: May I check your cap?
Microtubules are hollow cylindrical polymers that have important roles in chromosome segregation, organelle transport and other processes inside cells. Microtubules are built from protein subunits called αβ-tubulin and abruptly switch between growing and shrinking. This switching property is known as "dynamic instability", and has captivated scientists since it was discovered over 30 years ago (Horio and Hotani, 1986; Mitchison and Kirschner, 1984).
The switch from the growing state to the shrinking state is known as catastrophe, and is essential for microtubules to work correctly. Catastrophe occurs when the microtubule loses its stabilizing cap, which is a biochemically distinct region near the growing end. Despite substantial efforts, both the size of this stabilizing cap and its relationship to microtubule growth rates have remained obscure. Now, in eLife, Thomas Surrey and co-workers at the Francis Crick Institute and the London Centre for Nanotechnology – including Christian Duellberg as first author – use state-of-the-art methods to resolve these longstanding conundrums (Figure 1; Duellberg et al., 2016).
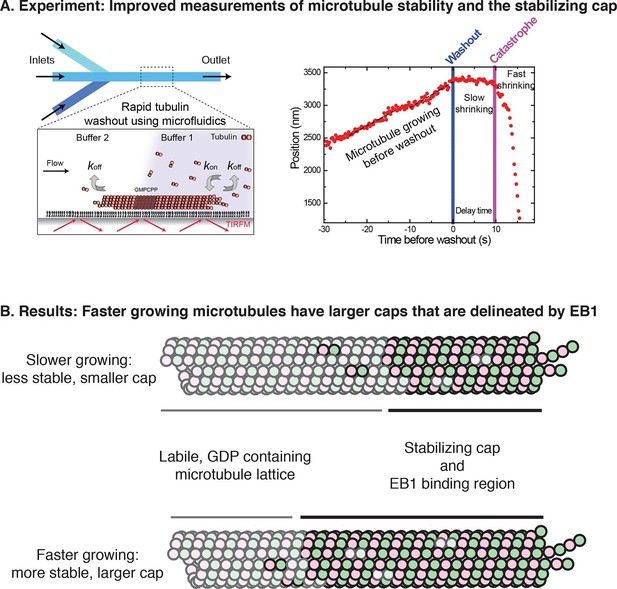
A modernized form of a classic technique enables the growth and stabilization of microtubules to be studied.
(A) Left: Duellberg et al. used microfluidics to abruptly stop microtubule growth via the "washout" approach. Right: Sample data showing microtubule length versus time. Before washout, the microtubule grows steadily; after washout, it shrinks slowly for a time; and after catastrophe, it shrinks rapidly (Panel adapted from Figures 1A and 2A, Duellberg et al.). (B) Duellberg et al. observed correlations between the microtubule growth rate and the size of the stabilizing cap, which consists of GTP-bound αβ-tubulin subunits (indicated by the non-faded circles). The caps are marked by EB1 proteins (not shown explicitly).
In a simple sense, microtubule catastrophe results from a race between two competing processes. Microtubules grow by capturing αβ-tubulin subunits that are bound to a molecule called GTP. However, shortly after a new subunit is added to the polymer, its GTP molecule is hydrolyzed: this destabilizes the polymer and provides the driving force for catastrophe.
The time interval between the addition of the subunit and the hydrolysis of the GTP should create a cap that protects the growing microtubule against catastrophe. This simple view predicts that faster growing microtubules should have larger caps. Over the years, however, experiments to test this prediction have yielded conflicting results (Caplow and Shanks, 1996; Schek et al., 2007), resulting in a proliferation of different models that attempt to describe microtubule stabilization (reviewed in Bowne-Anderson et al., 2013).
“Washout” experiments are a classic way to measure microtubule stability and estimate cap size by suddenly diluting the solution around growing microtubules to stop their growth (Walker et al., 1991). After washout, the microtubule shrinks slowly for a short period of time before it begins to shrink rapidly. The time delay before rapid shrinking occurs is related to the size of the stabilizing cap. Using this approach, a classic early paper failed to find a relationship between the rate at which microtubules grow and their stability (Walker et al., 1991).
Duellberg et al. have now revitalized this washout approach by developing a new microfluidics-based method that enables much faster dilution (Figure 1A). This method also incorporates high-precision microtubule end tracking and averaging techniques previously developed by Surrey and co-workers (Maurer et al., 2014).
The new approach allowed Duellberg et al. to demonstrate that microtubules that are growing faster at the time of dilution experience a longer delay before they begin to rapidly shrink (Figure 1B). The cap size could also be estimated from the length of the slow-shrinking phase. Thus, microtubule growth rate and microtubule stability are correlated.
To provide more direct insight into the size of the cap and how it is lost, Duellberg et al. turned to the EB1 family of microtubule regulatory proteins. These proteins form ‘comets’ by binding to an extended region near the microtubule end (Bieling et al., 2007), and are thought to recognize unique structural features of the stabilizing cap (Zhang et al., 2015).
To determine whether microtubule stability is related to the size of EB comets, Duellberg et al. simultaneously monitored microtubule ends and fluorescently tagged EB proteins. They observed that faster growing microtubules recruited more EB protein at the growing end (as shown by more intense fluorescent ‘comets’). The number of high-affinity EB binding sites decreased exponentially after washout, and rapid shrinking began when the density of EB binding sites was reduced to about 20% of its maximal value.
Consistent with these findings, microtubules with more EB binding sites (brighter comets) at the time of dilution exhibited a longer time delay before rapid shrinking began. This time delay can also be predicted if the rates of three processes are known: microtubule growth, slow shrinkage and EB binding site loss.
By demonstrating that EB proteins label the cap region and therefore provide a way to visualize it, and by resolving a longstanding ambiguity about the relationship between the cap and microtubule growth rate, Duellberg et al. advance our understanding of microtubule stabilization. However, we cannot currently explain Duellberg et al.’s findings in terms of the conformations and biochemical properties of individual αβ-tubulin subunits. More generally, microtubules normally undergo catastrophe without the sudden dilution used by Duellberg et al.: defining the mechanisms that normally trigger catastrophe therefore remains another important challenge.
The high-quality data that Duellberg et al. report set a new standard for studies of other microtubule regulatory proteins. Their pioneering quantitative analyses will undoubtedly contribute to future advances in the understanding of dynamic instability and its regulation.
References
-
Evidence that a single monolayer tubulin-GTP cap is both necessary and sufficient to stabilize microtubulesMolecular Biology of the Cell 7:663–675.https://doi.org/10.1091/mbc.7.4.663.
-
Microtubule assembly dynamics at the nanoscaleCurrent Biology 17:1445–1455.https://doi.org/10.1016/j.cub.2007.07.011
Article and author information
Author details
Publication history
Copyright
© 2016, Geyer et al.
This article is distributed under the terms of the Creative Commons Attribution License, which permits unrestricted use and redistribution provided that the original author and source are credited.
Metrics
-
- 1,360
- views
-
- 117
- downloads
-
- 0
- citations
Views, downloads and citations are aggregated across all versions of this paper published by eLife.
Download links
Downloads (link to download the article as PDF)
Open citations (links to open the citations from this article in various online reference manager services)
Cite this article (links to download the citations from this article in formats compatible with various reference manager tools)
Further reading
-
- Biochemistry and Chemical Biology
- Structural Biology and Molecular Biophysics
The two identical motor domains (heads) of dimeric kinesin-1 move in a hand-over-hand process along a microtubule, coordinating their ATPase cycles such that each ATP hydrolysis is tightly coupled to a step and enabling the motor to take many steps without dissociating. The neck linker, a structural element that connects the two heads, has been shown to be essential for head–head coordination; however, which kinetic step(s) in the chemomechanical cycle is ‘gated’ by the neck linker remains unresolved. Here, we employed pre-steady-state kinetics and single-molecule assays to investigate how the neck-linker conformation affects kinesin’s motility cycle. We show that the backward-pointing configuration of the neck linker in the front kinesin head confers higher affinity for microtubule, but does not change ATP binding and dissociation rates. In contrast, the forward-pointing configuration of the neck linker in the rear kinesin head decreases the ATP dissociation rate but has little effect on microtubule dissociation. In combination, these conformation-specific effects of the neck linker favor ATP hydrolysis and dissociation of the rear head prior to microtubule detachment of the front head, thereby providing a kinetic explanation for the coordinated walking mechanism of dimeric kinesin.
-
- Structural Biology and Molecular Biophysics
The canonical chemokine receptor CXCR4 and atypical receptor ACKR3 both respond to CXCL12 but induce different effector responses to regulate cell migration. While CXCR4 couples to G proteins and directly promotes cell migration, ACKR3 is G-protein-independent and scavenges CXCL12 to regulate extracellular chemokine levels and maintain CXCR4 responsiveness, thereby indirectly influencing migration. The receptors also have distinct activation requirements. CXCR4 only responds to wild-type CXCL12 and is sensitive to mutation of the chemokine. By contrast, ACKR3 recruits GPCR kinases (GRKs) and β-arrestins and promiscuously responds to CXCL12, CXCL12 variants, other peptides and proteins, and is relatively insensitive to mutation. To investigate the role of conformational dynamics in the distinct pharmacological behaviors of CXCR4 and ACKR3, we employed single-molecule FRET to track discrete conformational states of the receptors in real-time. The data revealed that apo-CXCR4 preferentially populates a high-FRET inactive state, while apo-ACKR3 shows little conformational preference and high transition probabilities among multiple inactive, intermediate and active conformations, consistent with its propensity for activation. Multiple active-like ACKR3 conformations are populated in response to agonists, compared to the single CXCR4 active-state. This and the markedly different conformational landscapes of the receptors suggest that activation of ACKR3 may be achieved by a broader distribution of conformational states than CXCR4. Much of the conformational heterogeneity of ACKR3 is linked to a single residue that differs between ACKR3 and CXCR4. The dynamic properties of ACKR3 may underly its inability to form productive interactions with G proteins that would drive canonical GPCR signaling.