Real-time imaging of Huntingtin aggregates diverting target search and gene transcription
Figures
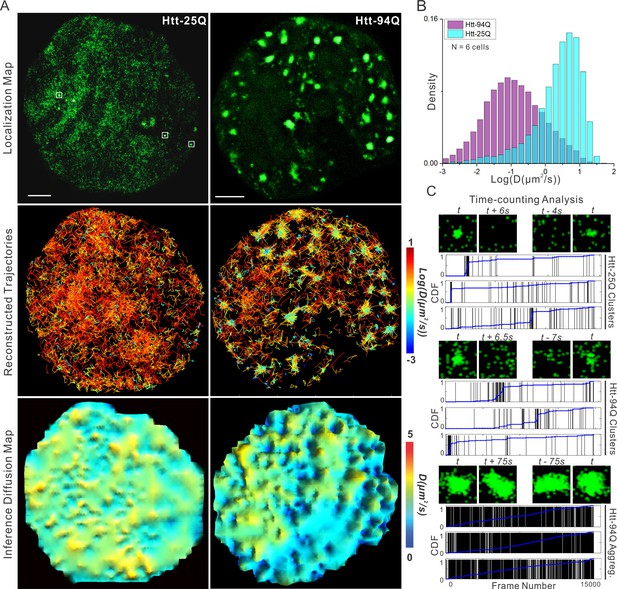
mHtt protein displays three distinct states in living cells.
(A) Top, localization map of live-cell PALM data set for the indicated Htt fragment. The PALM data were recorded at 20 ms per frame using imaging conditions specified in Materials and methods. Diffraction-limited Htt-25Q aggregates are indicated by square boxes (White). Middle, reconstructed single-molecule trajectories reflecting sub-cellular molecular diffusion. Each trajectory is color-colored using the diffusion coefficient calculated by the linear regression of the MSD curve with a R2 > 0.9. 2000 trajectories are shown for the Htt-25Q condition. Twice as many trajectories are shown for the mHtt-94Q condition to ensure relatively equal sampling and representation in regions without mHtt-94Q aggregates. Bottom, diffusion map calculated by a Bayesian inference- based method (El Beheiry et al., 2015). Trajectories with minimal 2 connected localizations were considered in this analysis. Each pixel in the image is color-coded with the most probable diffusion coefficient for that pixel calculated by InferenceMap. Scale bars, 2 µm. (B) Histogram of diffusion coefficients calculated same as in the middle panel of (A) for the indicated condition. Htt-25Q, 7266 trajectories; mHtt-94Q, 19,975 trajectories. N = 6 cells. (C) The temporal history of localizations in each selected region: vertical line (Black) indicates the frame from which the localization is detected. The blue trend line represents the cumulative distribution function of the total localizations detected before a given frame number. Three representative regions are shown for each condition. The images above each plot are sliding-window localization maps from Video 3, showing the temporal dynamics of Htt protein from each category. t indicates when the cluster/aggregate is clearly visible. Scale bars, 2 µm
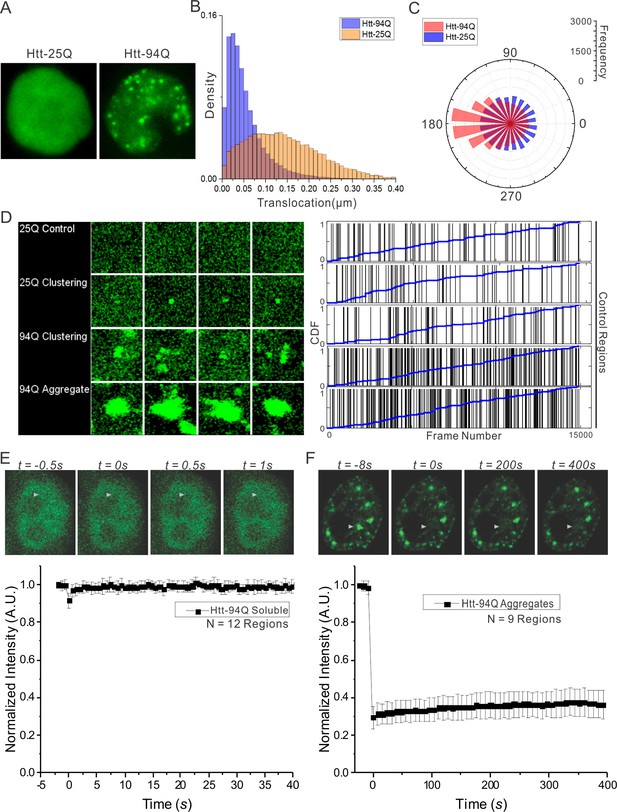
Dynamics of Htt protein in live cells.
(A) Representative Epi-illumination Images for Htt-25Q-mEOS3.2-NLS and mHtt-94Q-mEOD3.2-NLS containing cells. (B) Density histogram of single-molecule translocation distances between two adjacent imaging frames (For calculation, see details in Materials and methods). 60,702 (Htt-25Q, yellow) and 149,970 (mHtt-94Q, blue) translocations were considered in this analysis. N = 6 cells. (C) Rose histogram of three-point jumping angles for the indicated Htt fragment (See details in Materials and methods). For normalization purposes, 30,000 randomly selected angles are binned and showed for each condition. N = 6 cells. (D) Left, Maximal intensity projection image for Video 4 showing final static localization maps for each type of regions indicated on the left. Right, the temporal history of Htt-25Q localizations in control regions with no visible clusters (presented same as in Figure 1C). (E) FRAP analysis on mHtt-94Q containing cells without visible clusters or aggregates. After initial focused bleaching at the Region of Interest (white arrow), confocal images were acquired at 2 fps for 40 s (Video 1, Left). Average gray-scale intensity recovery curve from 12 measurements is plotted as the function of time. Error bars represent Standard Deviation. (F) FRAP analysis on mHtt-94Q aggregates. After initial focused bleaching at the Region of Interest (white arrow), time-lapse confocal images were acquired with 8 s intervals for 400 s (Video 1, Right). Average gray-scale intensity recovery curve from 9 measurements is plotted as the function of time. Error bars represent Standard Deviation.
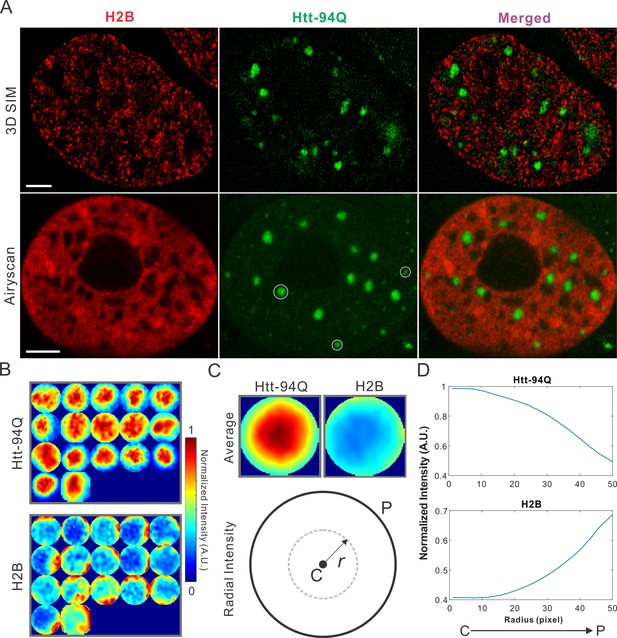
mHtt aggregates exclude chromatin.
(A) Top, live-cell 3D SIM imaging of chromatin (H2B-HaloTag-JF549, red) and mHtt aggregates (mHtt-94Q-CFP, Green). See Video 5 for 3D rendering. Bottom, Airyscan imaging of fixed cells with the same two-color labeling. (B) Size-normalized (100 pixel diameter) fluorescence intensity maps for 17 mHtt aggregate containing regions as circled in (A). The intensity maps for corresponding regions in the H2B channel are represented in the bottom panel. (C) Intensity maps for different regions shown in (B) are summed and averaged for each channel. The mean radial intensity trend line for each channel is calculated as the function of the radius from the center (C) to the peripheral (P). (D) Radial intensities are plotted as the function of the radius for mHtt-94Q (Top) and H2B (Bottom) channel. N, 17 regions from 8 cells. Scale bars, 2 µm
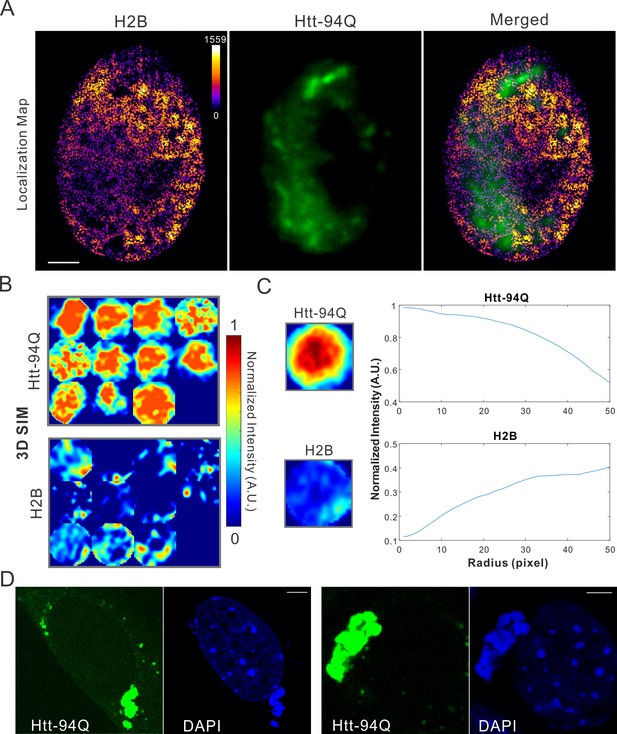
Map spatial relationship between chromatin and mHtt aggregates.
(A) live-cell H2B density map generated from single-molecule localizations acquired with the imaging condition specified in Materials and methods. 145,976 localizations were utilized for the reconstruction.The localization map (left) is overlaid with the mHtt-94Q-CFP-NLS aggregate channel (Middle), producing the merged image (right). (B) Size-normalized intensity maps for different mHtt aggregate regions for live-cell 3D-SIM imaging experiments. N, 11 regions from 6 cells. (C) Radial intensities are plotted as the function of the radius for mHtt-94Q (Top) and H2B (Bottom) channel. (D) Enrichment of DAPI staining signals at cytoplasmic mHtt aggregates. Scale bars, 2 µm
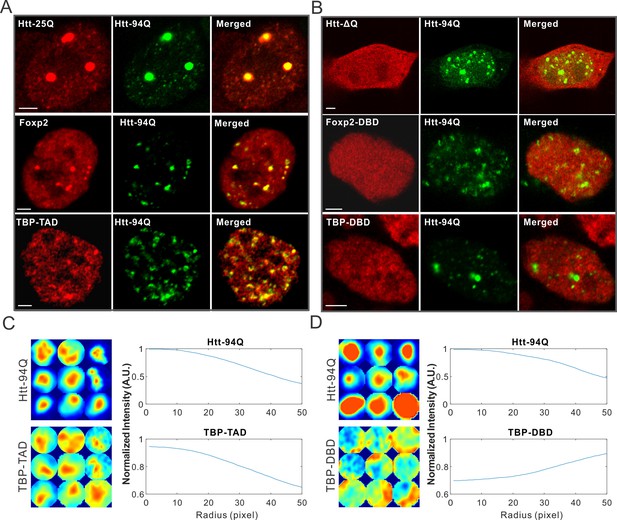
mHtt aggregates non-specifically interact with Htt-25Q, Foxp2, and TBP via PolyQ containing domain.
(A) Airyscan images showing enrichment of Htt-25Q-HaloTag, HaloTag-Foxp2 and HaloTag-TBP-TAD domain (labeled with JF646) at mHtt aggregate regions (mHtt-94Q-mEOS3.2-NLS). (B) Deletion of 25Q in Htt-25Q or PolyQ enriched TAD in Foxp1 and TBP abolishes the recruitment of these factors to mHtt aggregates. (C) And (D) Radial intensity curves as plotted in Figure 2D for mHtt-94Q (Top) and TBP-TAD (C) or DBD (D) (Bottom) channel. N, 9 regions from 5 cells. Scale bars, 2 µm
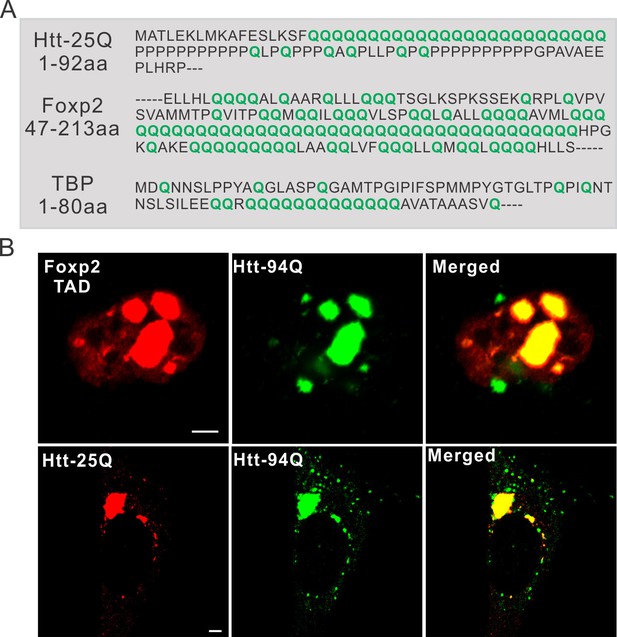
PolyQ tracts are important for aggregate binding.
(A) Protein sequence information for PolyQ enriched domains in wild-type Htt, TBP and Foxp2. Q is highlighted in green. (B) Airyscan Images showing specific enrichment of Foxp2-TAD (in ES cells) and Htt-25Q (in STHdh cells) to mHtt aggregates. Scale bars, 2 µm
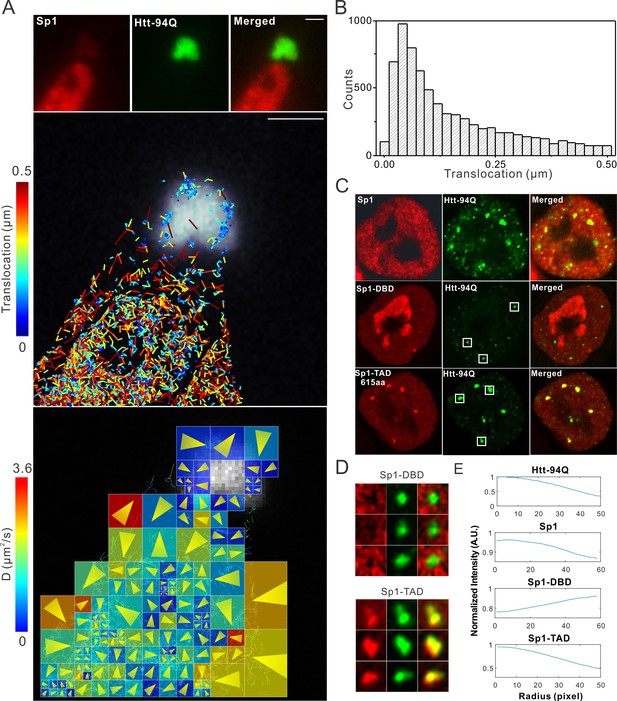
Single-molecule imaging of Sp1 binding to mHtt aggregates.
(A) Single-molecule imaging of cytoplasmic Sp1-mHtt aggregate interaction Top, Epi-fluorescence images of JF549-HaloTag-Sp1 (red) and mHtt-94Q-CFP (green) before single-molecule imaging Middle, 2D molecular interaction map of Sp1 binding to mHtt aggregates. Two-point translocations are color-coded according to the jumping distance shown in (B). 7399 translocations are included in this analysis. See Video 6 for single molecule tracks overlaid on raw data. A total of 215 (trajectories) Sp1 binding events were detected on the surface of the aggregate. Bottom, Diffusion map generated by InferenceMap as shown in Figure 1A. Each pixel in the image is color-coded with the most probable diffusion coefficient for that pixel. (B) Histogram for jumping distances shown in the middle panel of (A). (C) Two-color Airyscan images of ES cells expressing indicated Sp1 fragment (red, JF646) and mHtt-94Q-mEOS3.2-NLS (green). (D) Zoom-in view of mHtt aggregate regions boxed in (C) shows that Sp1-TAD is recruited to mHtt aggregates while Sp1-DBD is excluded. Radial intensity analysis of HaloTag-Sp1, HaloTag-DBD and HaloTag-TAD (Imaging data are shown in Figure 4—figure supplement 1B) shows specific enrichments of Sp1 and Sp1-TAD but depletion of Sp1-DBD at mHtt aggregate regions. Scale bars, 2 µm
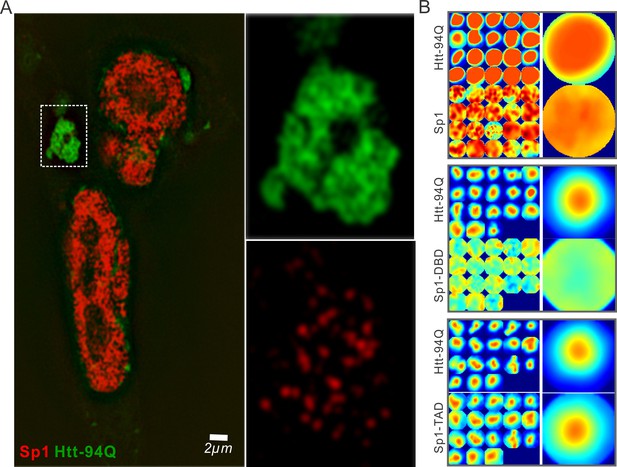
Sp1 non-specifically interacts with mHtt aggregates in live cells.
(A) Live-cell SIM imaging shows nonspecific association of Sp1 (red) to the mHtt aggregates (green) in the cytoplasm. Zoom-In view of the boxed region is on the right. (B) Size-normalized (100 pixel diameter) fluorescence intensity maps for Sp1, Sp1-TAD and Sp1 DBD at mHtt aggregate regions (Imaging data for Figure 4E). For each condition, the intensity maps for identical mHtt aggregate regions are on the top. Average intensity maps for each channel are shown in the left. N for Sp1; 20 regions in 12 cells. N for Sp1-DBD; 18 regions in 10 cells. N for Sp1-TAD; 18 regions in 8 cells. Scale bars, 2 µm
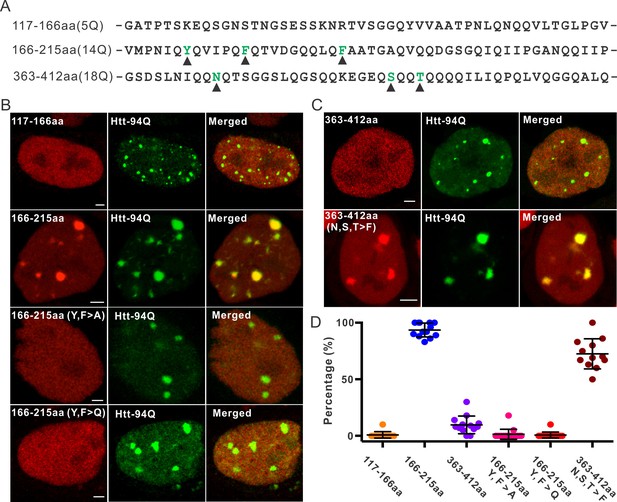
Aromatic acids are critical for Sp1 binding to mHtt aggregate.
(A) Protein sequence information for Sp1 fragments shown in (B). The number of glutamines in each sequence is in the parenthesis on the left. The amino acids mutated in the panel (B) are highlighted by black triangles below. (B) And (C) – upper panel, Airyscan images show that out of three fragments list in panel (A), only Sp1 166-215aa (14Q) fragment is specifically recruited to mHtt aggregates. Mutation of aromatic amino acids (Y, F) to A or even Q abolishes the interaction. (C) – lower panel, Converting 3 amino acids (N, S, T) in Sp1 363-412aa (18Q) fragment to F enables this fragment to bind mHtt aggregates. (D) Co-localization dot plot for results shown in (B) and (C). Each dot denotes one cell. The percentage of mHtt aggregates displaying enrichment for the indicated Sp1 fragment (X-axis) in single cells is calculated and plotted on the Y-axis. Scale bars, 2 µm
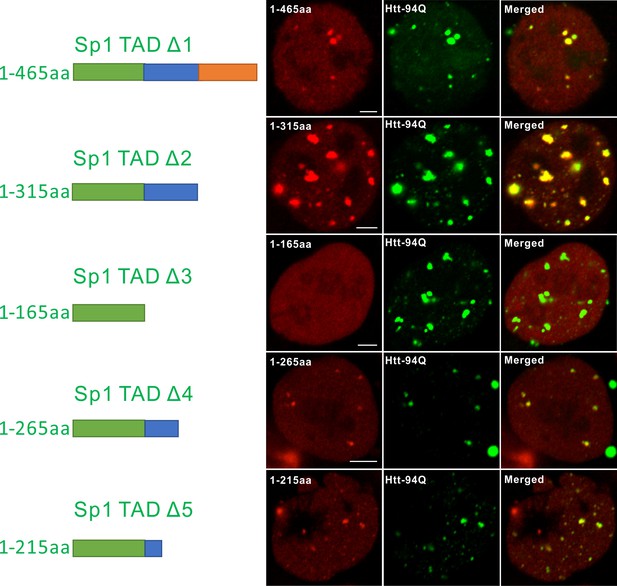
Mapping atypical Sp1:mHtt aggregate interaction domain.
Aryscan images showing that the Sp1:mHtt aggregate binding activities reside in the 166-215aa (14Q) fragment. Truncated Sp1 fragments (N-terminal HaloTag fusion, JF646 labeling, red), mHtt aggregates (mHtt-94Q-mEOS3.2, green). Left shows the schematics of the Sp1 fragment used.
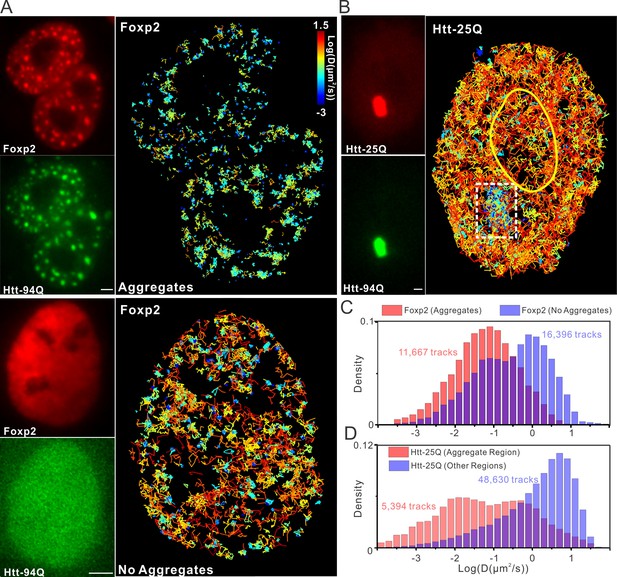
mHtt aggregates effectively slow down target search processes in living cells.
(A) Single-molecule tracking of Foxp2 in mHtt aggregate positive (upper) and negative cells (lower).The resulting single-molecule trajectories are color-coded using the diffusion coefficient calculated by the linear regression of the MSD curve with a R2 > 0.9. 6000 trajectories are shown for the Htt aggregate positive cells (upper). 2000 trajectories for the mHtt aggregate negative cell (lower). The epi-fluorescence images (red, JF646-HaloTag-Foxp2; green, mHtt-94Q-mEOS3.2-NLS) before single molecule imaging are on the left. (B) Single-molecule tracking of Htt-25Q in mHtt aggregate containing cells. The color-coding scheme is the same as in (A). 2000 trajectories are shown. The epi-fluorescence images (red, JF549-Htt-25Q-HaloTag; green, mHtt-94Q-CFP) before single molecule imaging are on the left. Trajectories are further divided to ‘In-aggregate’ and ‘out-of-aggregate’ fractions as shown in Figure 6—figure supplement 1A and B for downstream diffusion analysis in (D). mHtt aggregate region is indicated by the box with dotted line. The nucleus of the cell is labeled with the yellow contour line. See Video 7 for reconstructed trajectories overlaid on imaging data. (C) and (D) Histograms of diffusion coefficients for the indicated condition shown in (A) and (B). Foxp2 in aggregate positive cells, 11,667 trajectories (6 cells); Foxp2 in aggregate negative cells, 19,975 trajectories (8 cells). Htt-25Q at aggregation regions, 5394 tracks (6 cells); Htt-25Q at non-aggregation regions, 48,630 tracks (6 cells). Scale bars, 2 µm
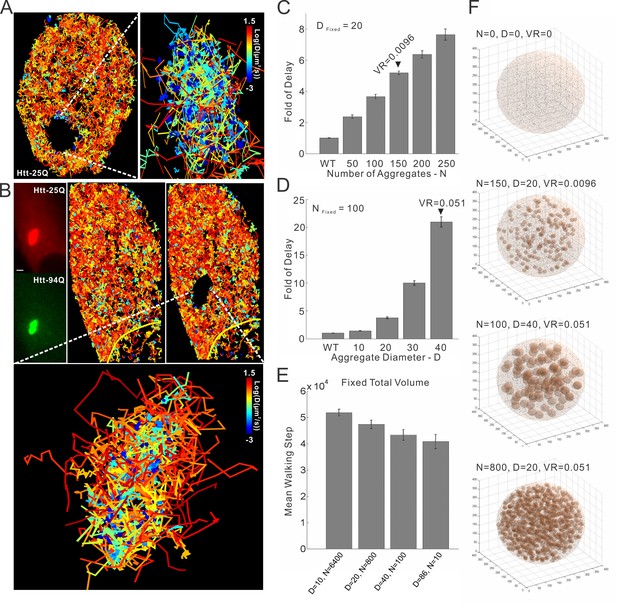
mHtt aggregates clog target search.
(A) Trajectories shown in Figure 6B are computationally divided into two populations based on the physical proximity of the trajectory to the mHtt aggregate region. Trajectories contain no localization events in the mHtt aggregate region are shown on the left. Trajectories fully or partially included in the mHtt aggregate region are shown on the right with a Zoom-in view. (B) A second example of single-molecule tracking of Htt-25Q in mHtt aggregate containing cells. The representation is the same as in Figure 6B and in the panel (A) of this figure. Scale bar, 2 µm (C) , (D) and (E). 3D Target search simulation in mHtt aggregate containing cells. See Video 9 for schematic illustration of the simulation. Detailed simulation parameters are in Materials and methods. Briefly, TF is injected into the cell at a random position. TF navigates through the cells via Brownian diffusion. The diffusion is slowed down 100 fold in spaces occupied by mHtt aggregate. The average first passage time in each condition is normalized to that in the cell without mHtt aggregates as the Fold of Delay. In the panel C, the diameter (D) of aggregates is fixed to 20 spatial units with varying Number (N) of aggregates. In the panel D, N is fixed to 100 with varying D. In the panel E, the total volume of aggregate in the cell is fixed to 5.1% volume ratio (VR, aggregate volume/the total cell volume) with varying N and D. (F) Images showing cells with different N, D and VR.
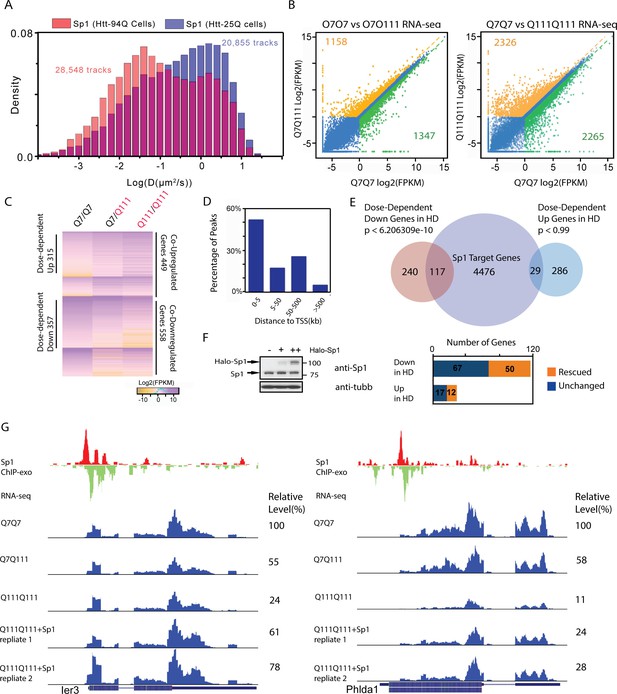
Elevated Sp1 levels rescue a subset of Htt-111Q dose-dependent down-regulated genes in cultured striatal cells.
(A) mHtt aggregates slow down Sp1 diffusion in the nucleus. Histogram (Red) of diffusion coefficients for Sp1 trajectories (28,548) in mHtt aggregate containing cells (N = 12 cells) and that for Sp1 trajectories (20,855) in Htt-25Q control cells (N = 12 cells). In this experiment, HaloTag-Sp1 is labeled with PA-JF646 (See Materials and methods for details). (B) mRNA-Seq differential gene-expression analysis for indicated sample pairs. Each dot in the plot represents one gene. The log2 scale values of FPKM (Fragments Per Kilobase of transcript per Million mapped reads) of the gene for the comparing samples are plotted. Orange dots, 1.5-fold up-regulation compared to wildtype; green dots, 1.5-fold down compared to wildtype. Orange number, total number of 1.5-fold up-regulated genes; Green number, total number of 1.5-fold down-regulated genes. (C) Heatmap showing Q111 dose-dependent gene expression changes. Genes that are up/down regulated in Q111 dose dependent or independent fashion are clustered. Co-regulated up/down genes: genes that are up/down regulated in both Q111/Q7 and Q111/Q111 striatal cells. The expression level of each gene is color-coded. For each group, genes are ranked by the expression levels in the wild-type control (Q7/Q7). (D) Percentage of Sp1 ChIP-exo peaks in core and proximal promoter (0–5 kb), distal region (5–50 kb), intergenic region (50–500 kb and > 500 kb) of annotated Refseq TSS in wild type striatal cells (Q7/Q7), the distribution was calculated by GREAT (McLean et al., 2010). (E) Venn diagram showing overlaps between Sp1 target genes and genes showing Q111 dose-dependent changes in Htt mutant striatal cells. Sp1 target genes are defined as genes with Sp1 peaks within 10 kb of their TSS. 124 of dose-dependent down-regulated genes have Sp1 binding events within 10 kb from their TSS (p<2.07e-8, hypergeometric test). (F) Sp1 overexpression in Htt homozygous mutant cells (Q111Q111) rescues the expression levels of a fraction of its direct target genes. Left panel, western blot using anti-Sp1 antibody to show expression of endogenous and Halo-tagged Sp1 in Q111/Q111 striatal cells with no (-), low (+) and high (++) expression level of HaloTag-Sp1. Q111/Q111 lines with high HaloTag-Sp1 expression level were used in RNA-seq experiments to evaluate gene expression rescue. Right panel, Bar graph showing the numbers of rescued (1.5 fold up- or down- regulated compared to Q111/Q111) and unchanged Sp1 dose-dependent targets upon HaloTag-Sp1 overexpression. (G) Representative ChIP-exo and RNA-seq tracks of Q111 dose-dependent downregulated Sp1 target genes that were rescued by HaloTag-Sp1 overexpression. Left panel: Ier3, Right panel: Phlda1. ChIP-exo tracks show 5’-end of sequence tags on the sense (red) and anti-sense (green) strands. For RNA-seq tracks, y-axle was set to the same scale (0–460 for Ier3, 0–675 for Phlda1).
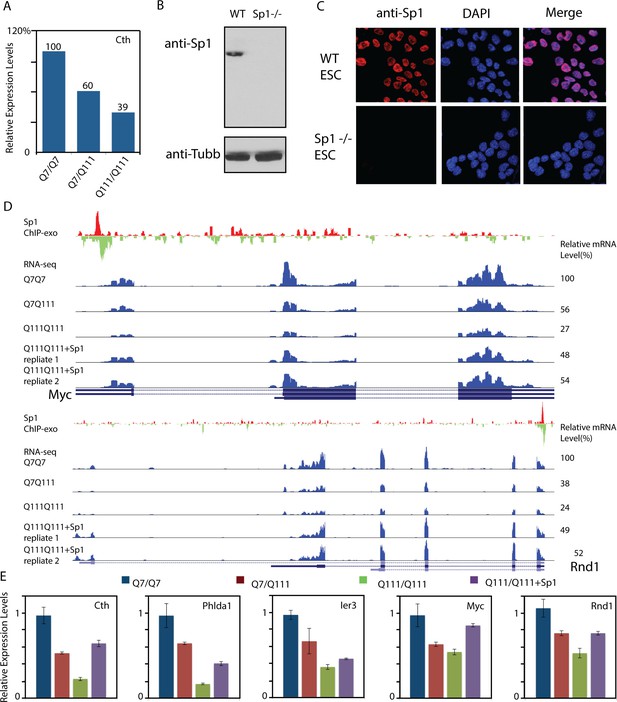
Over-expression of Sp1 restores gene expression defects in HD affected striatal cells.
(A) Cystathione gamma-lyse (Cth) expression levels in wild-type (Q7/Q7), heterozygous (Q7/Q111) and homozygous (Q111/Q111) striatal cells measured by RNA-seq. Expression levels of Cth FPKM in different samples are normalized to that in wildtype striatal cells. (B) Sp1 antibody detects a single band in western blot. Whole cell extracts of wild type and Sp1 null ES cells were analyzed by western blot with Tubulin as the loading control. (C) Sp1 immunofluorescence staining of wild-type (upper panel) and Sp1 null (lower panel) ES cells. Nuclei were counterstained with DAPI. (D) Representative ChIP-exo and RNA-seq tracks of Q111 dose-dependent downregulated Sp1 target genes that were rescued by HaloTag-Sp1 overexpression. Upper panel: Myc, Lower panel: Rnd1. ChIP-exo tracks show 5’-end of sequence tags on the sense (red) and anti-sense (green) strands. For RNA-seq tracks, y-axle was set to the same scale for different samples (0–692 for Myc, 0–125 for Rnd1). (E) Q-PCR analysis of indicated genes in different striatal cell lines, including Q7/Q7, Q7/Q111, Q111/Q111, and Q111/Q111 with Sp1 over-expression. Error bars present standard deviations.
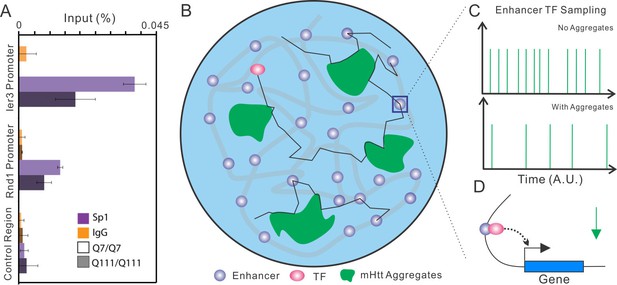
Model of Htt aggregates affecting TF dynamics.
(A) Sp1 target site occupancies in Q7/Q7 and Q111/Q111 cells assayed by ChIP-qPCR. The ChIP signals are normalized to the amount of input chromatin DNA. (B–D) Large numbers of decoy binding sites created by Htt aggregates in the cell increase trial-and-error collision steps before a TF finds its cognate target site. So, the apparent Kon of TF binding to specific sites is reduced. This leads to reduction of target site sampling frequencies (C). Presumably if the TF is a critical transcriptional activator for a gene, the target gene expression will be impeded as well (D).
Videos
FRAP experiments in mHtt aggregate negative (left) and positive (right) cells.
The time lapse confocal imaging interval for the aggregate negative cell is 0.5 s. That for the aggregate positive cell is 8 s.
FRAP experiments in mHtt aggregate negative (left) and positive (right) cells performed in the same field of view, showing that laser power is sufficient for bleaching.
The fluorescence recovery in cells with soluble mHtt-94Q is rapid, while that at aggregate regions is much slower.
Sliding-window representation for live-cell PALM datasets of Htt-25Q (Left) and mHtt-94Q (Right).
PALM Imaging is performed with a rate of 50 Hz. In each frame, single-molecule localizations from adjacent 1000 frames were pooled and plotted. The interval for the sliding window is 0.5 s (25 frames).
Zoom-In view of indicated types (Left) of region from Video 3, showing distinct local molecular dynamics.
https://doi.org/10.7554/eLife.17056.008Live-cell 3D SIM imaging with ES cells expressing H2B-HaloTag (JF549 labeling, Red) and mHtt-94Q-CFP (Green).
In the video, 2D slices in the axial direction are looped 3 times followed by a rotational 3D volume rendering.
First segment: Single-molecule trajectories superimposed on Htt-aggregate channel.
Trajectories are randomly color-coded. Second segment: Diffusion map calculated by InferenceMap superimposed on Htt-aggregate channel. Third segment: Reconstructed single-molecule trajectories of Sp1 overlaid on raw imaging data with dragon presentation (20 step delayed). Presentation software: InferenceMap
Reconstructed single-molecule trajectories of Htt-25Q (HaloTag, JF549) overlaid on imaging data in STHdh cells with dragon presentation (6 step delayed).
Magenta; Htt-25Q single-molecule imaging. Green; mHtt-94Q-CFP Epi-fluorescence image. Presentation software: Imaris.
Zoom-in view at the mHtt aggregate containing region, showing non-specific trapping of Foxp2 at the single-molecule level.
Presentation software: Fiji.
Target search simulation in Htt-aggregate containing cell.
Small black dots and semi-transparent spheres represent target sites and mHtt aggregates, respectively. TF molecule movement is slowed down (red fragments) in the aggregate regions. Presentation software: Matlab
Additional files
-
Supplementary file 1
The table lists primers, gBlocks and localization parameters used in the study.
- https://doi.org/10.7554/eLife.17056.027
-
Supplementary file 2
RNA-seq analysis of different striatal cell lines (Q7/Q7: wild-type; Q7/Q111: mHtt heterozygous mutant; Q111/Q111 mHtt homozygous mutant; Q111/Q111+Sp1: Sp1 overexpressed mHtt homozygous mutant.
- https://doi.org/10.7554/eLife.17056.028
-
Supplementary file 3
Sp1 ChIP-exo-seq analysis.
Table lists all Sp1 peaks called by MACS2 (Q-value cutoff: 5.00e-02)
- https://doi.org/10.7554/eLife.17056.029
-
Supplementary file 4
Sp1 target prediction.
Table lists genes with Sp1 binding sites within 5 kb from their TSS. Prediction was done by BETA minus
- https://doi.org/10.7554/eLife.17056.030