Mitochondria: Selective protein degradation ensures cellular longevity
Mitochondria provide cells with energy and metabolite molecules that are essential for cell growth, and faulty mitochondria cause a number of severe genetic and age-related diseases, including Friedreich's ataxia, diabetes and cancer (Held and Houtkooper, 2015; Suliman and Piantadosi, 2016). To maintain mitochondria in a fully working state, cells have evolved a range of quality control systems for them (Held and Houtkooper, 2015). For example, faulty mitochondria can be removed through a process called mitophagy. In this process, which is similar to autophagy (the process used by cells to degrade unwanted proteins and organelles), the entire mitochondrion is enclosed by a double membrane. In yeast cells this structure fuses with a compartment called the vacuole, where various enzymes degrade and destroy the mitochondrion (Youle and Narendra, 2011). In animal cells an organelle called the lysosome takes the place of the vacuole.
Now, in eLife, Adam Hughes, Daniel Gottschling and colleagues at the Fred Hutchinson Cancer Research Center and University of Utah School of Medicine report evidence for a new quality control mechanism that helps to protect mitochondria from age- and stress-related damage in yeast (Hughes et al., 2016). In this mechanism, a mitochondrion can selectively remove part of its membrane to send the proteins embedded in this region to the lysosome/vacuole to be destroyed, while leaving the remainder of the mitochondrion intact (Figure 1).
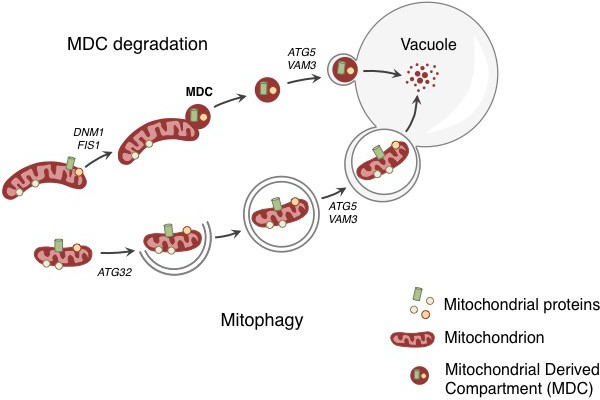
Degradation of mitochondria and selected mitochondrial membrane proteins.
Yeast cells respond to stress and aging by sending a specific set of mitochondrial membrane proteins into the vacuole for degradation (top). This pathway involves the formation of a mitochondrial derived compartment (MDC), a process that is regulated by genes that are required for mitochondrial fission (DNM1 and FIS1). The fusion of the MDC to the vacuole depends on genes that are involved in the late stages of autophagy and mitophagy (ATG5 and VAM3). The MDC degradation pathway is distinct from the process of mitophagy (bottom), which involves the whole mitochondrion being enclosed in a membrane (grey lines) as a result of the activity of a gene called ATG32. The membrane-enclosed mitochondrion is then transported to the vacuole, where it is degraded.
Hughes et al. tracked the fate of Tom70, a protein that is found in the outer membrane of mitochondria, and discovered that it accumulated in the vacuole as the yeast aged. This accumulation was not the result of mitophagy, as Tom70 was directed to the vacuole even when a gene required for mitophagy was absent. Previous reports have linked cellular aging to a decline in mitochondrial activity (McMurray and Gottschling, 2003), which is caused by an earlier loss of pH control in the vacuole (Hughes and Gottschling, 2012). For this reason, Hughes et al. tested whether a drug that disrupts the pH of the vacuole triggers the degradation of Tom70. This appears to be the case – the drug caused Tom70 to move from the mitochondria to the vacuole for degradation.
Before Tom70 ended up in the vacuole it accumulated in a mitochondrial-derived compartment (MDC) at the surface of the mitochondria, close to the membrane of the vacuole. The formation of this compartment depended on the machinery that drives the process by which mitochondria divide. However, the subsequent delivery of the contents of the MDC to the vacuole used factors that are required for the late stages of autophagy (Figure 1).
Hughes et al. found that the MDC contained Tom70 and 25 other proteins, all of which are mitochondrial membrane proteins that rely on Tom70 to import them into the mitochondrial membrane. This suggests that the MDC degradation pathway selectively removes a specific group of mitochondrial proteins. An obvious question, therefore, is: how and why does disrupting the ability of the vacuole to control its pH trigger the selective delivery and degradation of these proteins?
Hughes et al. hypothesize that MDC formation is linked to metabolite imbalance, as the loss of acidity inside the vacuole prevents amino acids from being stored there (Klionsky et al., 1990). This in turn leads to a build up of amino acids in the cytoplasm that can overburden the transport proteins that import them into the mitochondria (Hughes and Gottschling, 2012). In this scenario, the selective degradation of mitochondrial transport proteins by the MDC pathway can be seen as a response that protects the organelle against an unregulated, and potentially harmful, influx of amino acids.
While MDCs still formed in very old yeast cells, they remained in vesicle-like structures in the cytoplasm and were not delivered to the vacuole. It therefore appears possible that the accumulation of undegradable cytosolic MDCs in the cytoplasm can contribute to the decline of the aging cell, much like the accumulation of protein aggregates does (Nystrom and Liu, 2014; Hill et al., 2014).
The discovery of MDCs and their role in delivering specific mitochondrial proteins to the vacuole for degradation raises a number of questions that may shed further light on both this process and the trials of aging. Do the MDCs contain regulatory factors that are required for delivering proteins into the vacuole? Why do MDCs fail to be delivered into the vacuole in old cells? The degradative enzymes inside the vacuole do not work well under conditions of elevated pH. It will therefore also be important to learn how long the degradation of mitochondrial membrane proteins can be maintained under these conditions (Kane, 2006).
References
-
The where, when, and how of organelle acidification by the yeast vacuolar H+-ATPaseMicrobiology and Molecular Biology Reviews 70:177–191.https://doi.org/10.1128/MMBR.70.1.177-191.2006
-
The fungal vacuole: composition, function, and biogenesisMicrobiological Reviews 54:266–292.
-
Protein quality control in time and space – links to cellular agingFEMS Yeast Research 14:40–48.https://doi.org/10.1111/1567-1364.12095
-
Mitochondrial quality control as a therapeutic targetPharmacological Reviews 68:20–48.https://doi.org/10.1124/pr.115.011502
-
Mechanisms of mitophagyNature Reviews Molecular Cell Biology 12:9–14.https://doi.org/10.1038/nrm3028
Article and author information
Author details
Publication history
Copyright
© 2016, Malmgren Hill et al.
This article is distributed under the terms of the Creative Commons Attribution License, which permits unrestricted use and redistribution provided that the original author and source are credited.
Metrics
-
- 2,699
- views
-
- 451
- downloads
-
- 0
- citations
Views, downloads and citations are aggregated across all versions of this paper published by eLife.
Download links
Downloads (link to download the article as PDF)
Open citations (links to open the citations from this article in various online reference manager services)
Cite this article (links to download the citations from this article in formats compatible with various reference manager tools)
Further reading
-
- Cell Biology
The excessive cosolute densities in the intracellular fluid create a physicochemical condition called macromolecular crowding (MMC). Intracellular MMC entropically maintains the biochemical thermodynamic equilibria by favoring associative reactions while hindering transport processes. Rapid cell volume shrinkage during extracellular hypertonicity elevates the MMC and disrupts the equilibria, potentially ushering cell death. Consequently, cells actively counter the hypertonic stress through regulatory volume increase (RVI) and restore the MMC homeostasis. Here, we establish fluorescence anisotropy of EGFP as a reliable tool for studying cellular MMC and explore the spatiotemporal dynamics of MMC during cell volume instabilities under multiple conditions. Our studies reveal that the actin cytoskeleton enforces spatially varying MMC levels inside adhered cells. Within cell populations, MMC is uncorrelated with nuclear DNA content but anti-correlated with the cell spread area. Although different cell lines have statistically similar MMC distributions, their responses to extracellular hypertonicity vary. The intensity of the extracellular hypertonicity determines a cell’s ability for RVI, which correlates with nuclear factor kappa beta (NFkB) activation. Pharmacological inhibition and knockdown experiments reveal that tumor necrosis factor receptor 1 (TNFR1) initiates the hypertonicity-induced NFkB signaling and RVI. At severe hypertonicities, the elevated MMC amplifies cytoplasmic microviscosity and hinders receptor interacting protein kinase 1 (RIPK1) recruitment at the TNFR1 complex, incapacitating the TNFR1-NFkB signaling and consequently, RVI. Together, our studies unveil the involvement of TNFR1-NFkB signaling in modulating RVI and demonstrate the pivotal role of MMC in determining cellular osmoadaptability.
-
- Cell Biology
The actin cytoskeleton is a ubiquitous feature of eukaryotic cells, yet its complexity varies across different taxa. In the parasitic protist Trypanosoma brucei, a rudimentary actomyosin system consisting of one actin gene and two myosin genes has been retained despite significant investment in the microtubule cytoskeleton. The functions of this highly simplified actomyosin system remain unclear, but appear to centre on the endomembrane system. Here, advanced light and electron microscopy imaging techniques, together with biochemical and biophysical assays, were used to explore the relationship between the actomyosin and endomembrane systems. The class I myosin (TbMyo1) had a large cytosolic pool and its ability to translocate actin filaments in vitro was shown here for the first time. TbMyo1 exhibited strong association with the endosomal system and was additionally found on glycosomes. At the endosomal membranes, TbMyo1 colocalised with markers for early and late endosomes (TbRab5A and TbRab7, respectively), but not with the marker associated with recycling endosomes (TbRab11). Actin and myosin were simultaneously visualised for the first time in trypanosomes using an anti-actin chromobody. Disruption of the actomyosin system using the actin-depolymerising drug latrunculin A resulted in a delocalisation of both the actin chromobody signal and an endosomal marker, and was accompanied by a specific loss of endosomal structure. This suggests that the actomyosin system is required for maintaining endosomal integrity in T. brucei.