Proton currents constrain structural models of voltage sensor activation
Figures
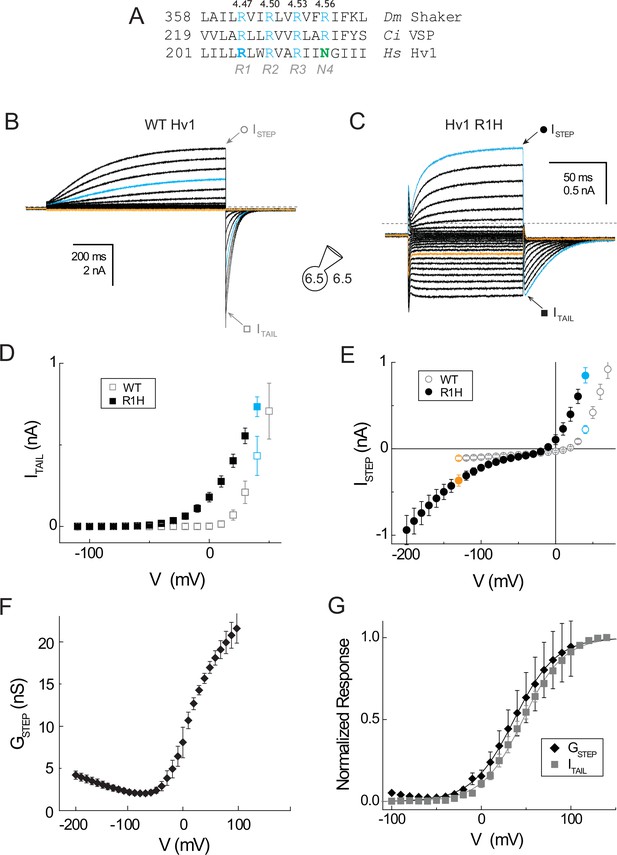
A resting-state H+ ‘shuttle’ conductance in Hv1 R1H.
(A) A multiple sequence alignment of the S4 helix in Drosophila melanogaster Shaker (GI:288442), Ciona intestinalis Voltage Sensitive Phosphatase (GI:76253898) and Homo sapiens Hv1 (GI:38783432) is shown. Numbering indicates amino acid position and bold typeface indicates residues that are mutated in this study. Conserved S4 Arg residues are shown in blue and Asn214 in Hv1 is green. (B, C) Whole-cell currents in a representative cell expressing Hv1 R1H are elicited by voltage steps from a holding potential of −60 mV to −130 mV through +70 mV in increments of +10 mV in a representative cell expressing WT Hv1 (B) or from a holding potential of −50 mV to −200 mV through +40 mV in increments of +10 mV (C). Tail currents measured at −90 mV and recording solutions (pHO 6.5, pHI 6.5) are indicated in the diagram. Symbols indicate the approximate times at which ISTEP (circles) and ITAIL (squares) are measured. Colored lines indicate currents measured at +40 mV (cyan) or −130 mV (orange) and dashed lines indicate the zero current amplitudes. (D, E) ITAIL-V (D) and ISTEP-V (E) relations are shown for WT Hv1 (open symbols) and R1H (filled symbols). Colored symbols indicate currents measured at +40 mV (cyan) and −130 mV (orange), as shown in B and C. Symbols represent means ± SEM from n = 4 (ISTEP, WT), n = 6 (ISTEP, R1H), n = 6 (ITAIL, WT), or n = 7 (ITAIL, R1H) cells. Linear leak currents are subtracted from the ITAIL-V relations in D. (F) The mean GSTEP-V relation calculated from data in E exhibits a ‘U’ shape in which the apparent maximal GSTEP amplitudes at positive and negative potentials are unequal. The data suggest that GAQ is open at positive potentials while the resting-state H+ shuttle conductance (GSH) is open at more negative voltages. (G) The voltage dependence of the intrinsic activated-state H+ conductance (GAQ) in Hv1 R1H is estimated from ITAIL (gray squares) measured at −90 mV in symmetrical pH 6.5 recording solutions as shown in B and C. GSTEP (black diamonds) is calculated from ISTEP (see Materials and methods). GSTEP and ITAIL are normalized to their apparent maxima in each cell and symbols represent the mean ± SEM from n = 6 (ISTEP) or n = 7 (ITAIL) cells. Solid lines represent fits of the data between −50 mV and +100 mV to a Boltzmann function (ITAIL, gray line: V0.5 = 46.5 mV, dx = 22.6; GSTEP, black line; V0.5 = 36.7 mV, dx = 23.4).
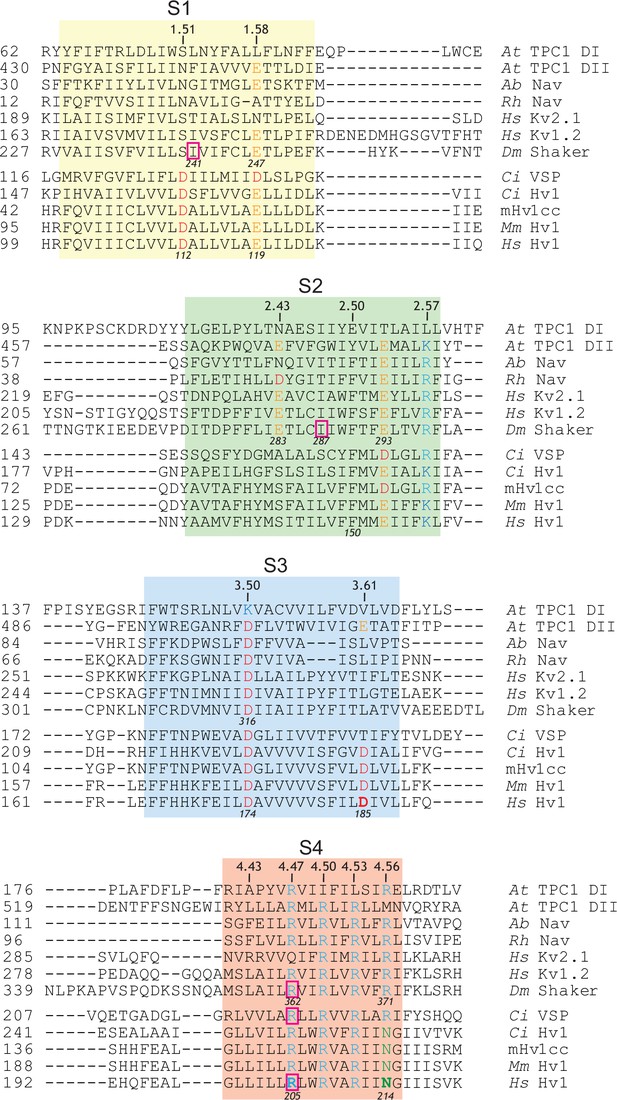
Amino acid sequence alignments of S4 helical segments in VS domain proteins.
(A) An alignment (Clustal V; DNAStar Lasergene) of amino acid sequences in VS domains from Arabidopsis thaliana TPC1 (pdb: 5E1J and 5DQQ; GI: 75166464), Arcobacter butzleri Nav (pdb: 3RVZ; GI:339961375); alpha proteobacterium HIMB114 Nav (pdb: 4DXW; GI:388326718), Homo sapiens Kv1.2 (NP_004966; GI: 4826784), Homo sapiens Kv2.1 (NP_004965; GI:4826782), Drosophila melanogaster Shaker (CAA29917; GI:288442), Ciona intestinalis VSP (NP_001028998; GI:76253898) and Hv1 channels from Ciona intestinalis (NP_001071937; GI:118344228), Mus musculus (NP_001035954; GI:109809757) and Homo sapiens (NP_115745; GI:91992155) is shown. Numbering on the left indicates amino acid position; italicized numbers indicated the positions of selected residues in Shaker and Hs Hv1 for comparison. Bold typeface indicates residues that were mutated in this study. Selected residues colored (red, acidic; blue, basic; green polar neutral).The approximate boundaries of helical segments are shown by colored boxes (S1, yellow; S2, green; S3, blue; S4, red). Residues that confer GSH when mutated to His are highlighted by pink boxes.
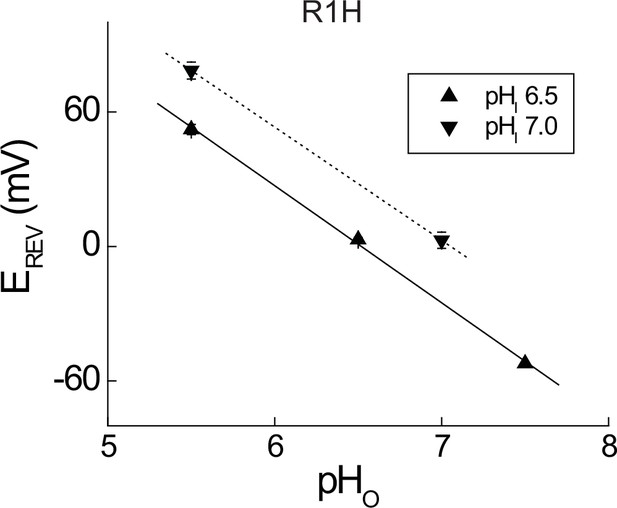
Measurement of GAQ selectivity in Hv1 R1H.
(A) Current reversal potentials (EREV) were determined from plots of the instantaneous ITAIL (estimated from mono-exponential fits) vs. the applied voltage as previously described (Ramsey et al., 2006). Data represent means ± SEM from n = 4–10 cells and lines represent linear fits to the mean EREV values with slope values of 52.2 mV/pH unit (pHI 6.5, solid line) and 50.5 mV/pH unit (pHI 7.0, dashed line), both of which are close to the expected value for a H+-selective conductance (~56 mV/pH unit under our recording conditions).
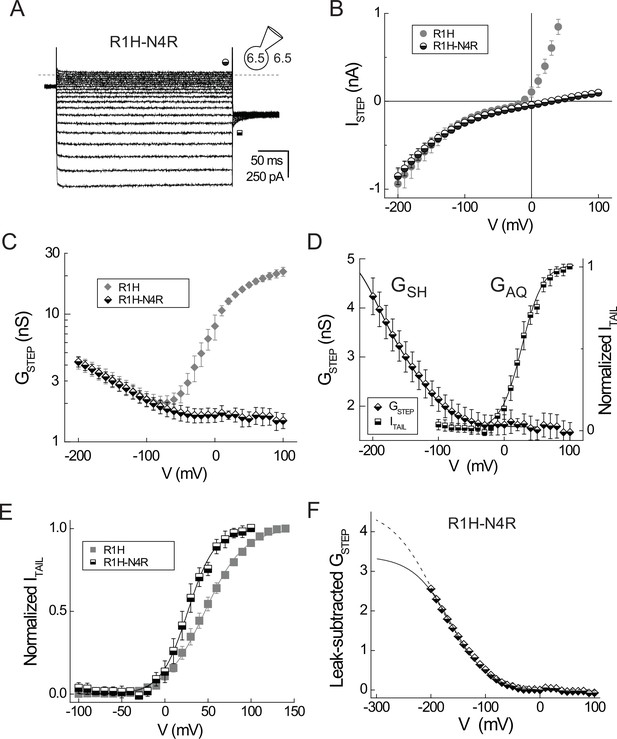
A second-site N4R mutation isolates GSH in Hv1 R1H.
(A) Representative whole-cell currents in a cell expressing Hv1 R1H-N4R are elicited by voltage steps to −150 mV through +40 mV in increments of +10 mV from a holding potential of −30 mV; ITAIL is measured at −90 mV. Recording solutions are symmetrical pH 6.5, as indicated. Symbols indicate approximate times at which ISTEP (half-filled circles) and ITAIL (half-filled squares) are measured; the dashed line indicates the zero current amplitude. (B) The ISTEP-V relations for R1H-N4R (half-filled circles) and R1H (filled gray circles; data from Figure 1) are plotted together for comparison. Note that outward ISTEP mediated by GAQ is apparently absent in R1H-N4R but inward currents mediated by GSH are similar in R1H and R1H-N4R. For R1H-N4R, symbols represent means ± SEM n = 4 cells. (C) GSTEP-V relations for R1H-N4R (half-filled diamonds) and R1H (filled gray diamonds; data from Figure 1) are shown without leak subtraction. Note the log scale for GSTEP. Data represent means ± SEM from n = 3 cells expressing R1H-N4R that exhibited similar resting-state current amplitudes. (D) GSTEP-V (half-filled diamonds) and normalized ITAIL-V (half-filled squares) relations for R1H-N4R are scaled to illustrate their relative positions on the voltage axis. Data represent means ± SEM from n = 3 cells with similar current amplitudes. ITAIL data from each cell is linear leak-subtracted and normalized to its amplitude at +100 mV prior to averaging. Lines represent fits of the data to single Boltzmann functions (GSTEP: GSTEPmin = 1.5 nS, GSTEPmax = 5.5 nS, V0.5 = −172.1 mV, dx = 40.4; ITAIL: V0.5 = 26.3 mV, dx = 16.3). (E) Leak-subtracted normalized ITAIL-V relations for R1H (filled gray squares; data from Figure 1) and R1H-N4R (half-filled squares) are plotted together for comparison. Lines represent fits to single Boltzmann functions (R1H: V0.5 = 46.5 mV, dx = 22.6; R1H-N4R: V0.5 = 26.3 mV, dx = 16.3). (F) GSH gating was estimated by fitting the leak-subtracted GSTEP-Vrelation to Boltzmann functions in which V0.5 is either free to vary (solid line: GSHmax = 3.4 nS, V0.5 = −164 mV, dx = 35.4) or constant (see Figure 4F, dashed line: V0.5 = VPEAK = −189 mV, GSHmax = 4.6 nS, dx = 42.1).
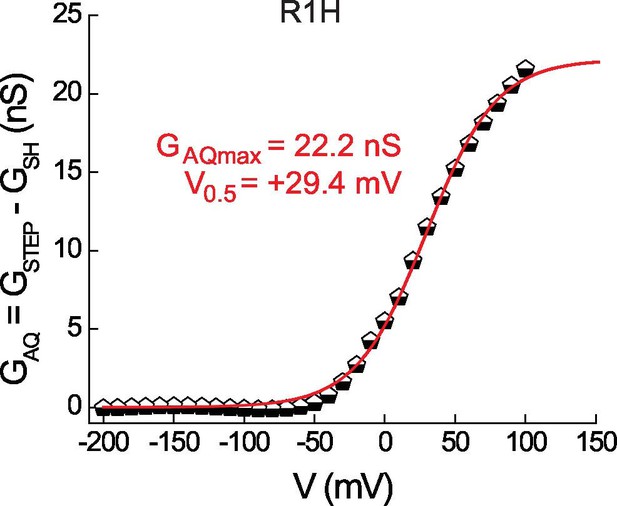
Estimating GAQ gating parameters from GSTEP-V relations in Hv1 R1H.
GAQ gating in R1H was estimated by subtracting GLEAK in R1H-N4R (data from Figure 2C) from the R1H GSTEP-V relation (data from Figure 1F) and fitting the data to a Boltzmann function (red line: GAQmax = 22.2 nS, V0.5 = +29.4 mV, dx = 24.9). Depending on the value used for GSHmax (see Figure 2F), GAQmax/GSHmax = 4.8 or 6.5.
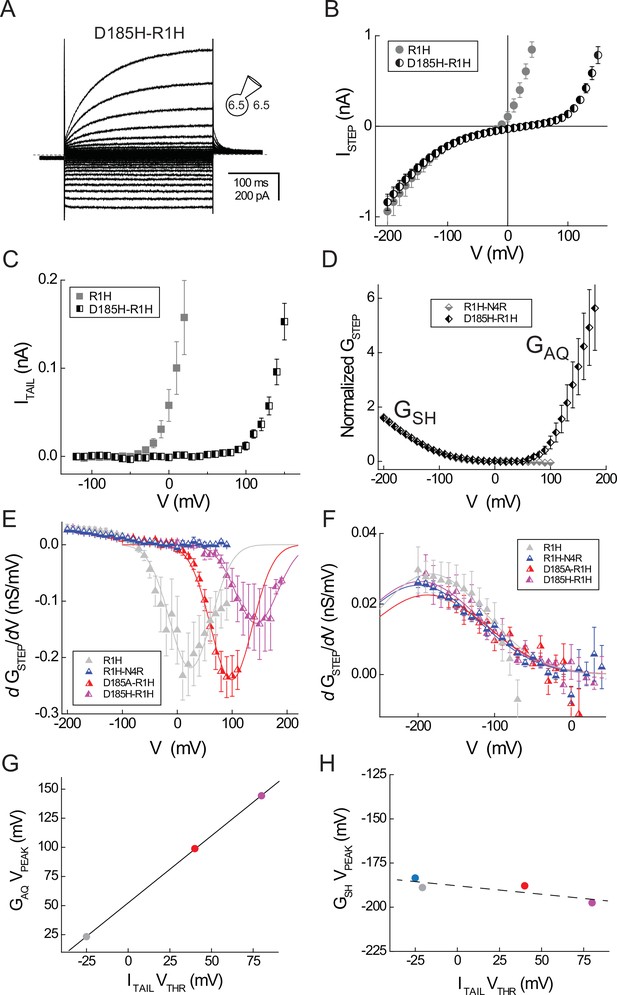
D185 mutations selectively affect GAQ gating.
(A) Representative whole-cell current records elicited by voltage steps from −120 mV to +150 mV in a cell expressing Hv1 D185H-R1H in symmetrical pH 6.5 recording solutions are shown. (B) The ISTEP-V relation for D185H-R1H (half-filled circles) is compared to R1H (filled gray circles; data from Figure 1). (C) The mean ITAIL-V relation for D185H-R1H (half-filled squares) is compared to R1H (filled gray squares; data from Figure 1). Linear leak currents are subtracted from the data. (D) The normalized leak-subtracted GSTEP-V relations for D185H-R1H (black half-filled diamonds) and R1H-N4R (gray half-filled diamonds; data from Figure 2) are compared. Linear GLEAK calculated between 0 mV and +50 mV is subtracted from the D185H-N4R data and GSTEP is normalized to its value at −140 mV. A Boltzmann fit to the mean D185H-R1H GSTEP-V relation between −200 mV and +50 mV (GMAX = 2.2, dx = 35.3, V0.5 = −164.9 mV; not shown) yields similar gating parameters to R1H-N4R (see Figure 2). (E) dGSTEP/dV-V relations are calculated from leak-subtracted GSTEP-V data measured in cells expressing R1H (gray triangles), R1H-N4R (blue triangles), D185A-R1H (red triangles), or D185H-R1H (violet triangles). For clarity, only data between −200 mV and +40 mV are shown in panel F. Lines represent Gaussian fits to the data between −100 mV and +100 mV (R1H, gray line: A = −20.8, ω = 76.2, VPEAK = +23.3 mV), −20 mV and +110 mV (D185A-R1H, red line: A = −22.9, ω = 76.2, VPEAK = +98.9 mV), or 0 mV and +190 mV (D185H-R1H, violet line: A = −13.3, ω = 76.2, VPEAK = +144.3 mV). For Gaussian fits to R1H and D185A-R1H data, ω is constrained to the value determined from a fit to D185H-R1H data (ω = 76.2). D185A-R1H and D185H-R1H data represent means ± SEM from n = 3 cells; R1H data are replotted from Figure 2 and R1H-N4R data is replotted from Figure 4. (F) Symbols represent dGSTEP/dV-V relations (panel E) between −200 mV and +40 mV only and lines represent Gaussian fits to the data (R1H, gray line: A = 5.1, ω = 143.8, VPEAK = −183.5 mV; R1H-N4R, blue line: A = 5.6, ω = 155.5, VPEAK = −188.9 mV; D185A-R1H, red line: A = 4.4, ω = 155.5, VPEAK = −187.9 mV; D185H-R1H, violet line: A = 5.1, ω = 155.5, VPEAK = −197.5 mV). (G, H) VPEAK values for GAQ (from E) and GSH (from F) gating are plotted against VTHR for GAQ-mediated ITAIL (data from Table 2) in cells expressing R1H (gray circle), R1H-N4R (blue circle), D185A-R1H (red circle) and D185H-N4R (violet circle). Note that GAQ VPEAK is not measured for R1H-N4R (G). Effects of D185 mutations on GAQ gating estimated from VPEAK and VTHR are strongly correlated (solid black line in G, R = 0.99) whereas the effects of mutations on GSH gating are weakly correlated with their effects GAQ gating (dashed black line in H, R = −0.84).
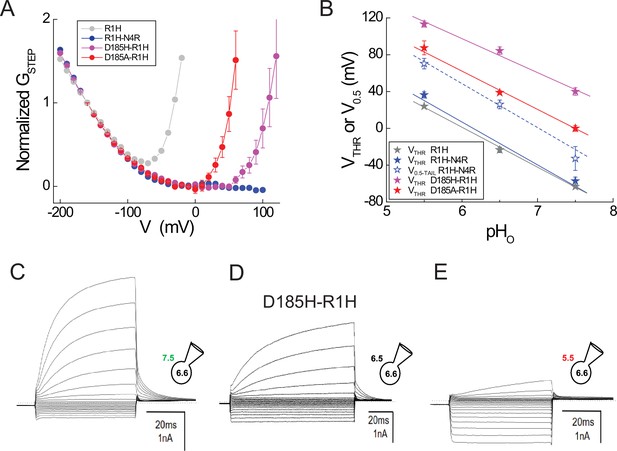
Effects of D185 mutations on GAQ and GSH gating in R1H.
(A) GSTEP-V relations in R1H (gray circles), R1H-N4R (blue circles), D185H-R1H (violet circles) and D185A-R1H (red circles) normalized to GSTEP measured at −150 mV. Data represent means from n = 6 (R1H; data from Figure 2), n = 4 (R1H-N4R; data from Figure 4), n = 3 (D185H-R1H) or n = 5 (D185A-R1H) cells. Error bars (S.E.M.) are shown for D185H-R1H and D185A-R1H only. (B) VTHR (filled stars) or V0.5 (open stars) for GAQ gating is plotted in function of pHO for R1H (gray stars), R1H-N4R (blue stars), D185H-R1H (violet stars) and D185A-R1H (red stars). Lines indicate linear fits to the data (gray line, R1H VTHR: −43.7 mV/pH unit; blue line, R1H-N4R, VTHR: −46.7 mV/pH unit; dashed blue line, R1H-N4R V0.5, −41.0 mV/pH unit; violet line, D185H-R1H VTHR: −38.0 mV/pH unit; red line, D185A-R1H, VTHR: −43.8 mV/pH unit). (C–E) Representative currents elicited by voltage steps (−200 mV to +100 mV in 20 mV increments) at pHO 7.5 (C), pHO 6.5 (D) or pHO 5.5 (E).
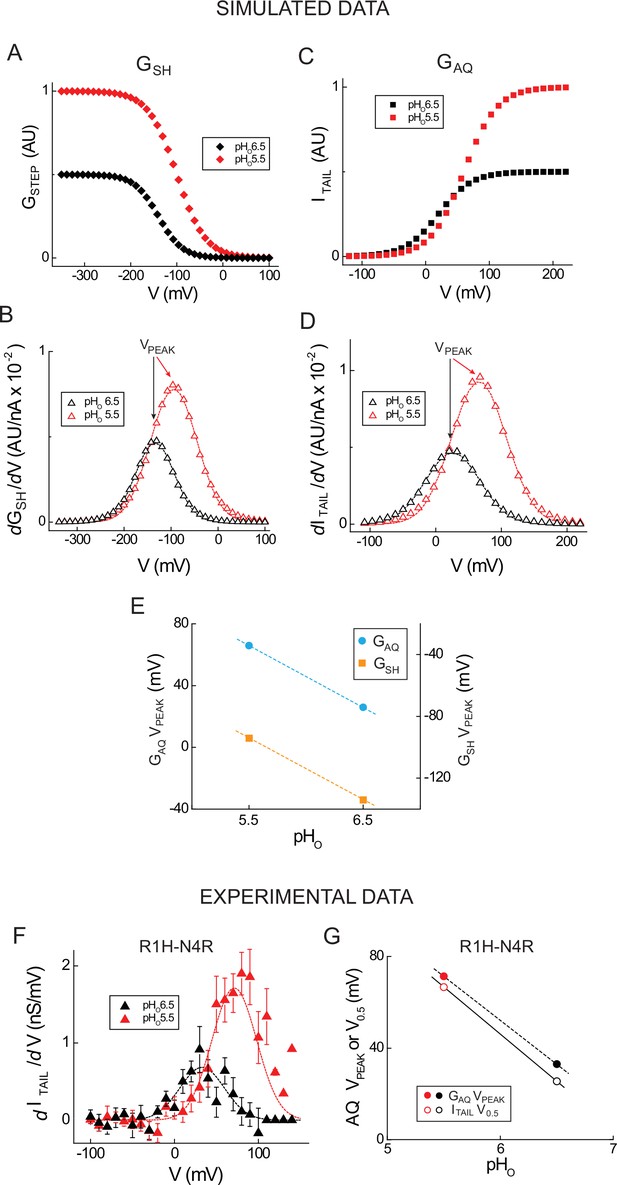
Estimating GAQ and GSH gating parameters from the first derivative of GSTEP-V.
(A, C) GSTEP-V relations for GSH (A, pHO 5.5: red diamonds, V0.5 = −140 mV, dx = 31.0, GMAX = 1.0; pHO 6.5: black diamonds, V0.5 = −100 mV, dx = 31.0, GMAX = 0.5) and ITAIL for GAQ (C, pHO 5.5: red squares, V0.5 = 60 mV, dx = 26.0, GMAX = 1.0; pHO 6.5: black squares, V0.5 = 20 mV, dx = 26.0, GMAX = 0.5) at different pHO are based on Boltzmann functions. The positions of the GSH-V curves are chosen such that GSTEP is submaximal at −200 mV; V0.5 values for simulated GAQ-V relations at pHO 5.5 and pHO 6.5 are based on experimental data shown in panel F and separated by 40 mV. (B, D) The absolute values of the GSHdGSTEP-V/dV (B) and GAQdITAIL-V/dV (D) relations are plotted (open red triangles, pHO 5.5; open black triangles, pHO 6.5). Dashed lines represent fits of the simulated data to Gaussian functions (B, dashed red line, pHO 5.5: A = 0.97, ω = 99.1, VPEAK = −94.2 mV; B, dashed black line, pHO 6.5, A = 0.49, ω = 83.2, VPEAK = −134.2 mV; D, dashed red line, pHO 5.5: A = 0.97, ω = 83.2, VPEAK = 65.9 mV; D, dashed black line, pHO 6.5: A = 0.48, ω = 83.2, VPEAK = 25.9 mV). (E) VPEAK values from the Gaussian fits in B (GSH, orange squares) and D (GAQ ITAIL, cyan circles) are shown in function of pHO. Lines represent linear fits to the data (slope = 40 mV/pH unit). (F) Experimental GAQdITAIL-V/dV relations calculated from linear leak-subtracted data in Hv1 R1H-N4R (Figure 2E) are shown (filled red triangles, pHO 5.5; filled black triangles, pHO 6.5). Dashed lines represent fits to Gaussian functions (red line, pHO5.5: A = 114.3, ω = 53.3, VPEAK = +71.3 mV; black line, pHO 6.5: A = 45.7, ω = 53.3, VPEAK = +33.0 mV). (G) V0.5 values (open circles) determined from the Boltzmann fits to the GAQ ITAIL-V relations (Figure 2E) and VPEAK (filled circles) determined from Gaussian fits to GAQdITAIL-V/dV (panel G) are plotted in function of pHO (red circles, pHO 5.5, black circles, pHO 6.5). Lines represent slopes determined from linear fits to the data (GAQ VPEAK = −38.3 mV/pH unit; ITAIL V0.5 = −41.1 mV/pH unit).
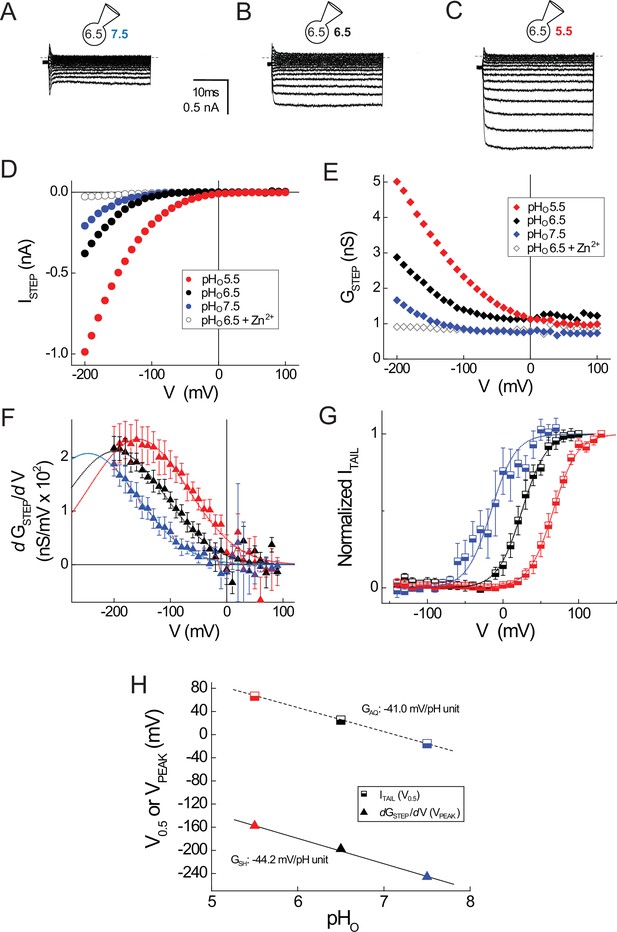
GSH and GAQ gating in R1H-N4R are similarly sensitive to changes in pHO.
(A–C) Representative whole-cell currents elicited by voltage steps (−200 mV to +100 mV in 10 mV increments) in a cell expressing R1H-N4R that was superfused with pHO 7.5 (A), pHO 6.5 (B) and pHO 5.5 (C) recording solutions are shown. (D) ISTEP-V relations at pHO 7.5 (filled blue circles), pHO 6.5 (filled black circles) or pHO 5.5 (filled red circles) are shown for the records in A–C. Open black circles represent ISTEP measured at pHO 6.5 + 1 mM Zn2+ in the same cell (raw traces not shown). (E) GSTEP-V relations at pHO 7.5 (filled blue diamonds), pHO 6.5 (filled black diamonds), pHO 5.5 (filled red diamonds) or pHO 6.5 + 1 mM Zn2+ (open black diamonds) calculated from the data in D are shown. (F) dGSTEP/dV is plotted in function of the membrane potential at which GSTEP was measured at pHO 5.5 (red triangles), pHO 6.5 (black triangles) or pHO 7.5 (blue triangles) in R1H-N4R. Data points represent means ± S.E.M. from n = 8 (pHO 5.5), n = 12 (pHO 6.5) or n = 10 (pHO 7.5) cells. Dashed lines represent fits of the mean dGSTEP/dV-V relations between −200 mV and +100 mV to Gaussian functions: red line, pHO 5.5: A = 5.2, ω = 176.4, VPEAK = −157.4 mV; black line, pHO 6.5: A = 4.7, ω = 176.4, VPEAK = −197.6 mV; blue line, pHO 7.5: A = 4.6, ω = 176.4, VPEAK = −245.7 mV. (G) ITAIL-V relations after steps to the indicated voltages at pHO 7.5 (half-filled blue squares), pHO 6.5 (half-filled black squares), or pHO 5.5 (half-filled red squares) are shown. ITAIL is normalized to the apparent maximum current at each pHO. Data points represent means ± S.E.M. in n = 9 (pHO 5.5), n = 10 (pHO 6.5) or n = 4 (pHO 7.5) cells. Lines represent fits of the mean data to Boltzmann functions (red line, pHO 5.5: V0.5 = +66.3 mV, dx = 16.2; black line, pHO 6.5: V0.5 = +25.0 mV, dx = 16.2; blue line, pHO 7.5: V0.5 = −15.7 mV, dx = 16.2). (H) The pHO dependence of GSH (estimated from fitted VPEAK values in mean dGSTEP/dV-V relations; triangles) and GAQ (estimated from fitted V0.5 values in mean ITAIL-V relations; squares) is compared. Lines represent linear fits of the data (GSH: −44.2 mV/pH unit; GAQ: −41.0 mV/pH unit).
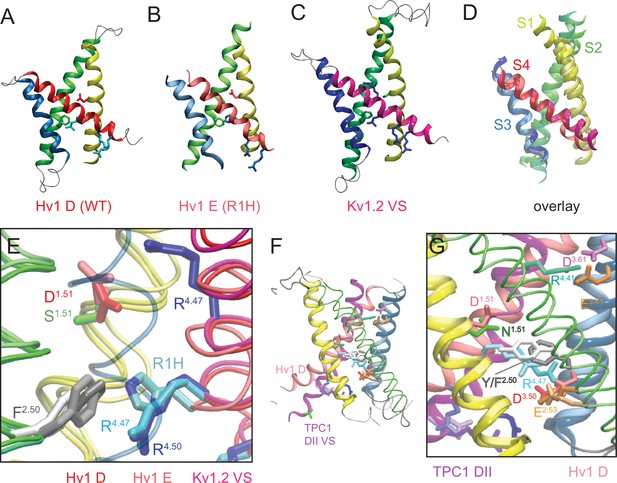
New Hv1 D (WT) and Hv1 E (R1H) VS domain resting-state model structures.
(A–D) Ribbon diagrams represent backbone structures in snapshots taken from MD simulations of the Hv1 D (WT) VS domain resting-state model structure (A), Hv1 E R1H mutant model structure (B), resting-state Kv1.2 VS domain Rosetta model structure (Pathak et al., 2007) that was used as the template for construction of Hv1 D (C). The model structures in A–C are overlain in D to illustrate their overall structural similarity. Transmembrane helical backbones in A–D are color coded: S1, yellow; S2, green; S3, blue; S4 red. Video 1 shows similar representations of Hv1 D, Hv1 E and Kv1.2 resting-state model structures rotated about the vertical axis. (E) An overlay of the Hv1 D, Hv1 E and Kv1.2 resting-state Rosetta VS domain model structures illustrates the relative positions of S1-S4 helical backbones (tubes colored as in A–D). Selected side chains (Hv1 D: D112/D1.51, red; F150/F2.50, light gray; R1/R205/R4.47, cyan; Hv1 E: D112/D1.51, light red; F150/F2.50, white; R1H/R205H, cyan/blue; Kv 1.2: S176/S1.51, green; F233/F2.50, dark gray; R1/R4.47 and R2/R4.50, blue) are shown in colored licorice. For clarity, only the S3 helix from Hv1 D (transparent blue tube) is shown. (F) The backbone structures of Hv1 D model and At TPC1 DII X-ray (pdb: 5EJ1) VS domains are overlain. S1, S3 and S4 helices are shown as ribbons and S2 helices are shown as tubes. Helical segments are colored as in A–D and loop regions are gray; lighter shades represent Hv1 D and darker shades represent TPC1. Selected side chains in Hv1 D/TPC1 (D/N1.51, red/green; F/Y2.50, gray/white; D/E3.61, magenta/orange) are shown in colored licorice. (G) A magnified view of the overlain Hv1 D and TPC1 structures illustrates the similar positions of selected side chains, which are shown in colored licorice (Hv1 D: D112/D1.51, pale red; F150/F2.50, light gray; E153/E2.53, pale orange; D174/D3.50, pale red; D185/D3.61, pale magenta; R1/R205/R4.47, pale cyan; TPC1: N443/N1.51, green; Y475/Y2.50, white; E478/E2.53, orange; D500/D3.50, red; E511/E3.61, orange; R531/R4.41, aqua; R537/R1/R4.47, cyan).
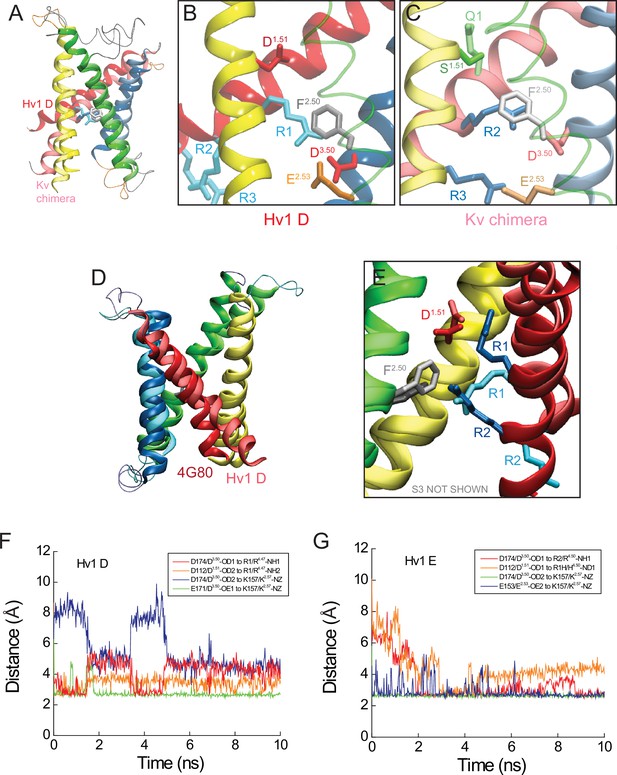
Comparison of Hv1 D to Kv1.2–2.1 chimera model and Ci VSP ‘down’ X-ray VS domain resting-state structures.
(A) The backbone structures of Hv1 D and a putative resting conformation of the Kv1.2–2.1 chimera (Jensen et al., 2012) VS domain are superimposed. Helical backbones are shown as colored ribbons (as in Figure 5; lighter shades represent Kv chimera). (B, C) Magnified views of Hv1 D (B) and Kv chimera (C) highlight the relative positions of selected side chains shown in colored licorice and labeled (Hv1 D: D112/D1.51, red; F150/F2.50, gray; E153/E2.53, orange; D174/D3.50, red; R1-R3, cyan; Kv chimera: S180/S1.51, green; F237/F2.50, white; E240/E2.53, orange; D263/D3.50, red; Q294/Q1/Q4.47, green; R297/R2/R4.50 and R300/R3/R4.53, blue). For clarity the S2 helix is represented as a transparent green tube in B and C. (D) The backbone structures of Hv1 D and Ci VSDD (pdb: 4G80) are superimposed. Helical backbones are represented as ribbons and colored as in A–C; S4 is pink in Hv1 D and red in Ci VSDD. (E) Magnified view of the superimposed Hv1 D and Ci VSDD structures shown in D (S3 helices are omitted for clarity). Selected side chains are shown in colored licorice and labeled (Hv1 D: D112/D1.51, pale red; F150/F2.50, white; R1/R4.47 and R2/R4.50, cyan; Ci VSDD: D129/D1.51, red; F161/F2.50, gray; R1/R4.47 and R2/R4.50, blue). (F, G) Distances between indicated atoms in Hv1 D (F) and Hv1 E (G) that are predicted to participate in stable salt bridges (cutoff distance = 3.5 Å, VMD 1.9.2) are plotted in function of time during the last 10 ns of an all-atom MD simulation.
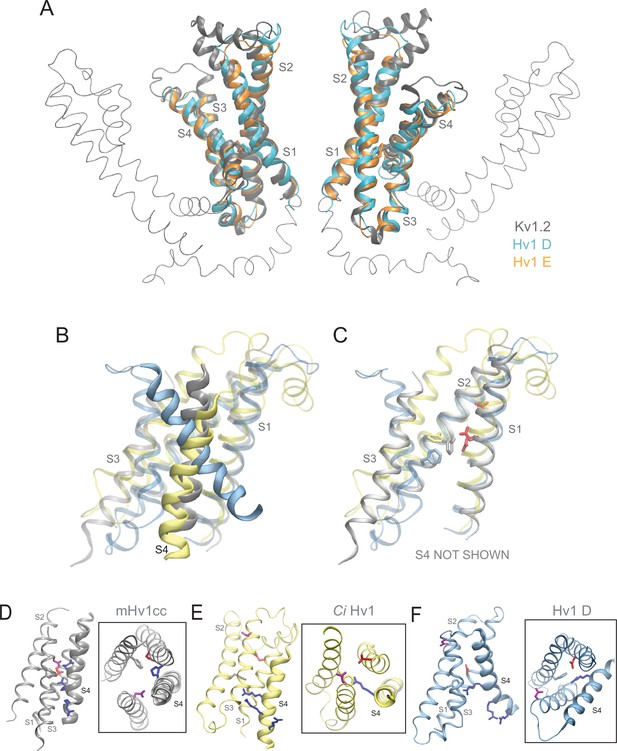
Comparison of Hv1 D and Hv1 E to Kv1.2 resting-state Rosetta model, Ci Hv1 resting-state model, and mHv1cc closed-state X-ray VS domain structures.
(A) Ribbon diagrams represent backbone structures of the VS and pore domains in a resting-state Kv1.2 VS domain Rosetta model (gray) superimposed with Hv1 D (cyan) and Hv1 E (orange) VS domains. The pore domain selectivity filter is oriented parallel to membrane normal. (B–C) An overlay of mHv1cc (gray), Ci Hv1 (yellow) and Hv1 D (cyan) backbone ribbon diagrams illustrates how S4 exhibits a more pronounced tilt (B) relative to S1-S3, which are similarly oriented in each of the three structures (C). D112/D1.51 (red) and F150/F2.50 (backbone color) side chains are shown in colored licorice. S4 is omitted for clarity in C. (D–F) Backbone structures of mHv1cc (D, gray), Ci Hv1 (E, yellow) and Hv1 D (F, cyan) resting-state VS domain models are represented by colored ribbons viewed side-on or from the extracellular space (boxed insets). The locations of S1-S4 helices are indicated by labels and S4 is rendered as a thicker ribbon. Selected side chains corresponding to D112/D1.51 (red), F150/F2.50 (backbone color), D185/D3.61 (violet) and R1/R4.47 (blue) are shown in colored licorice.
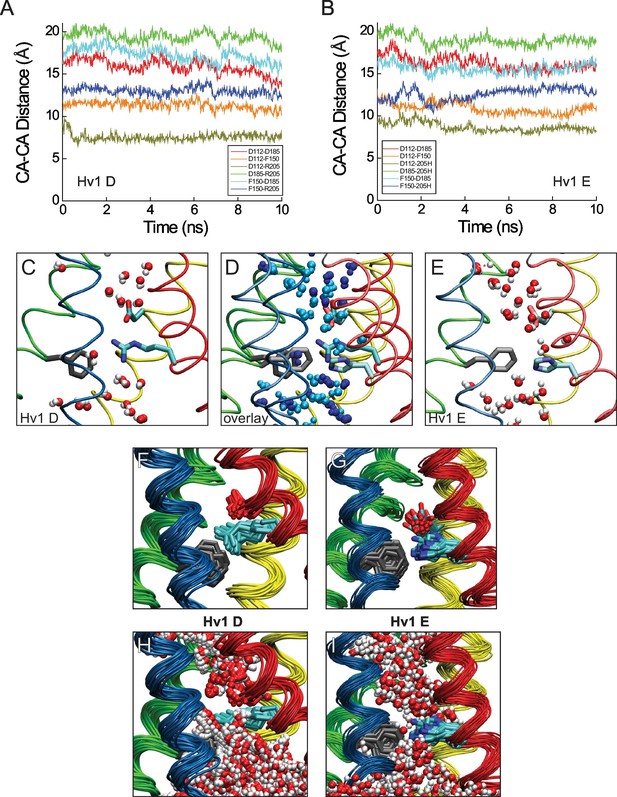
Atomic distances and central crevice hydration in Hv1 D and Hv1 E MD simulations.
(A, B) Distances between Cα (CA) atoms of selected residues (D112/D1.51, F150/F2.50, D185/D3.61 and either R1/R205/R4.47 or R1H/R205H) are plotted in function of time during the last 10 ns of an all-atom MD simulation conducted with Hv1 D (A) or Hv1 E (B) model structures. (C–E) Snapshots of the Hv1 D (C) and Hv1 E (E) model structures selected at t = 1 ns from the MD simulation trajectories are shown. Hv1 D and Hv1 E models are superimposed in panel D. Helices are shown as colored tubes (S1, yellow; S2, green; S3, blue; S4, red), F150/F2.50 is shown in gray licorice and D112/D1.51 and R1/R205/R4.47 are shown in colored licorice where carbon atoms are cyan, nitrogens are blue, and oxygens are red (hydrogens are not shown). Water molecules within 3.5 Å of protein are represented in CPK format, where oxygen is red and hydrogens are white. In the overlay (D), waters in the Hv1 D system are colored blue and waters in the Hv1 E system are colored cyan and backbone and side chains are represented by darker or lighter color shades in Hv1 D or Hv1 E, respectively. (F–I) Snapshots selected from 30 frames of the Hv1 D (F, H) and Hv1 E (G, I) MD simulation trajectories corresponding to (0.7−1.0 ns) shown in A an11 B are superimposed. For clarity, water molecules (represented as in C and E) are shown only in panels H (Hv1 D) and I (Hv1 E).
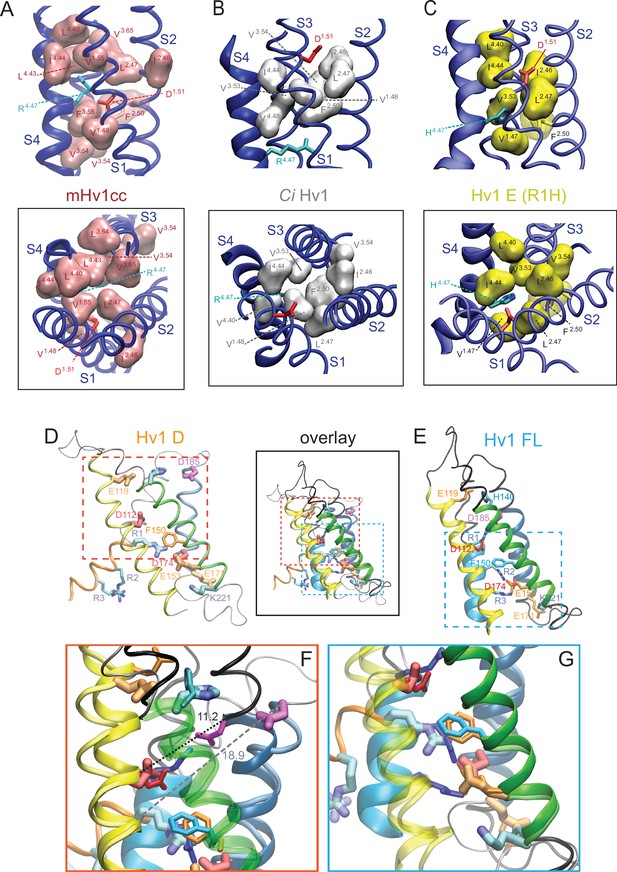
Structure of the resting-state GSH permeation pathway.
(A–C) Magnified views of mHv1cc (A), Ci Hv1 (B) and Hv1 E (C) resting-state VS domain X-ray and model structures viewed either side-on from the plane of the membrane (top panels) or from the extracellular space (bottom panels). Helical segments are shown as colored ribbons (S1: X1.42-X1.58, yellow; S2: X2.40-X2.57, green; S3: X3.47-X3.66, blue; S4: X4.39-X4.56, red). Selected ionizable side chains are shown in colored licorice representations: D12/D1.51 (red), D185/D3.61 (magenta), R1/R4.47 (cyan), R2/R4.50 (blue) and R3/R4.53 (violet). With the exception of F150/F2.50 (licorice, backbone color), hydrophobic residues listed in Table 1 are shown as space-filling representations; wireframe colors refer to residues X1.52 (cyan), V1.55 (red), L2.47 (blue) and V3.53 (green). mHv1cc I173/I3.53 was transferred from Ci VSP into the mHv1cc chimera sequence. L189/L3.65 is not represented in Hv1 E because it is above of the plane of view. Videos 2 and 3 show mHv1cc, Ci Hv1 and Hv1 E in motion viewed from the plane of the membrane (Video 2) or from the extracellular space (Video 3). (D, E) Hv1 D (D) and Hv1 FL (E) VS domain model structures are superimposed and backbone structures are shown as tubes (Hv1 D) or ribbons (Hv1 FL) colored as in Figure 5, except that S4 is orange in Hv1 D and cyan in Hv1 FL. Selected side chains are shown in colored licorice (D112/D1.51 and D174/D3.50, red; E119/E1.58, E153/E2.53 and E171/E3.47, orange; F150/F2.50, orange; D185/D3.61, magenta; H140/H2.40, R1/R4.47, R2/R4.50 and K221/K4.63, cyan/blue) as indicated by labels. Selected Hv1 D side chains are shown in thicker licorice representations and lighter color shades to facilitate comparison with Hv1 FL. Dashed lines in E indicate areas that are magnified in F and G. (F) Magnified view of the extracellular networks in Hv1 D and Hv1 FL VS domain model structures illustrate similarities and differences in the positions of selected residue side chains. Atomic distances (in Å) between the Cα atoms of R1 and D185 are indicated by black (Hv1 FL) or gray (Hv1 D) dashed lines. (G) Residues in the intracellular side of the Hv1 D and Hv1 FL VS domains are shown. In F and G, the S2 and S1 helices, respectively, are transparent. Video 5 shows Hv1 B and Hv1 FL resting-state model structures in rotation.
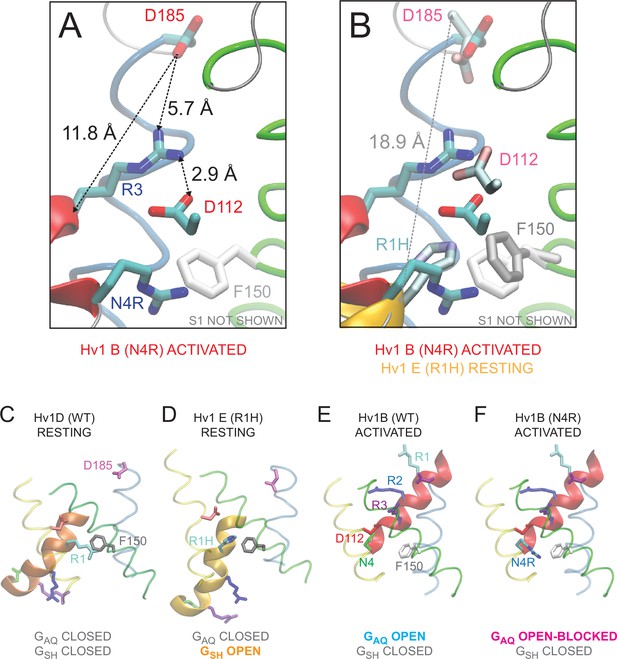
Comparison of resting- and activated-state Hv1 VS domain model structures.
(A) Hv1 B was mutated (N4R) in silico and subjected to energy minimization to demonstrate the possible position of the N4R side chain in a VS-activated (GAQ-blocked) conformation. Other atomic positions are not appreciably different from Hv1 B. S2 (green) and S3 (blue) helices are represented by colored tubes; S4 is shown as a red ribbon and S1 is not shown. Side chains of D112 (D1.51), D185 (D3.61), R3 (R4.53) and N4R (N4.56R) are shown in the colored licorice ‘element’ scheme (carbon, cyan; oxygen, red; nitrogen, blue) and the F150 (F2.50) side chain is white. Distances (in Å) between selected carboxylate oxygen atoms in D112 or D185 and either R3 nitrogen atoms or the R3 Cα atom are indicated by dashed arrows. (B) Positions of selected residue side chains in the Hv1 E mutant model structure (produced by in silico R1H mutation of Hv1 D) are superimposed on Hv1 B N4R shown in A. D112, D185 and R1H side chains are represented by ‘brushed metal’ coloring of licorice element representations; F150 is gray. The S4 helix in Hv1 E is shown as a gold ribbon; other helices are as shown in A. The dashed arrow indicates the distance (in Å) between the Cα atoms of D185 and R1H in Hv1 E. (C–F) Backbones of Hv1 D (C), Hv1 E (D), Hv1 B (E) and the Hv1 B N4R mutant (F) model structures are represented by thin (S1-S3) or thick (S4) colored ribbons and inter-helical loop regions are represented by gray tubes. Selected residue side chains are shown in colored licorice (D112/D1.51, red; F150/F2.50, gray or white; D185/D3.61, magenta; R1/R4.47, cyan; R2/R4.50, blue; R3/R4.53, violet; N4/N214/N4.56, green; N4R, cyan/blue). Structures are vertically aligned by the position of the F150/F2.50 Cα atom. Labels indicate the predicted functional state of the protein that correspond to the depicted structure. In C–F, helices are colored yellow (S1), green (S2) and blue (S3) and inter-helical loop regions are not shown for clarity; S4 residues 202–214 are colored red (Hv1 B), copper (Hv1 D) or gold (Hv1 E). Video 4 shows Hv1 B activated- and Hv1 D resting-state model structures in rotation.
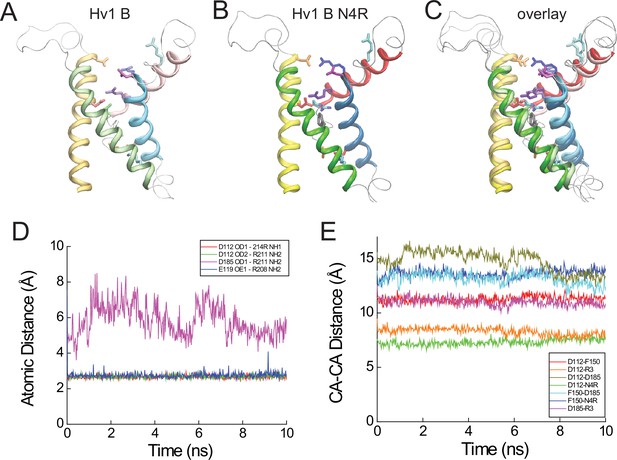
Atomic distances in resting- and activated-state Hv1 VS domain model structures.
(A–C) Hv1 B (A) and Hv1 B N4R (B) model structures are shown individually and superimposed (C). Helices are represented as colored ribbons (S1, yellow; S2, green; S3, blue; S4, red) and selected side chains are shown in colored licorice (D112/D1.51, red; E119/E1.58, orange; F150/F2.50, gray; D185/D3.61, magenta; R1/R4.47, cyan; R2/R4.50, blue; R3/R4.53, violet; N4/N4.56, green; N4R, cyan/blue). (D) Atomic distances between selected atoms in Hv1 D N4R that are predicted to participate in salt bridges (cutoff distance = 5 Å, VMD 1.9.2) are plotted in function of time during the final 10 ns of an all-atom MD simulation. (E) Distances between Cα (CA) atoms of selected residues (D112/D1.51, F150/F2.50, D185/D3.61 and N4R) in Hv1 B N4R are plotted in function of time during the last 10 ns of an all-atom MD simulation.
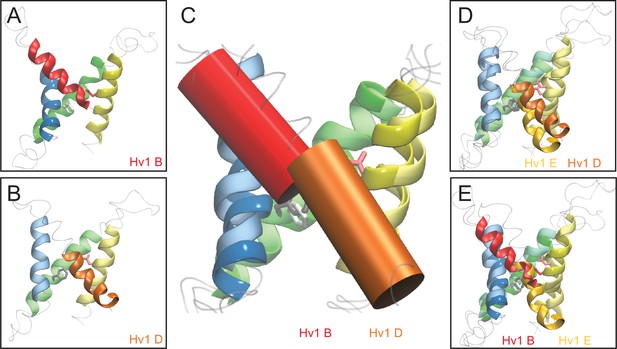
Comparison of resting- and activated-state Hv1 model structures.
(A, B) Ribbon diagrams show Hv1 B activated-state (A) and Hv1 D resting-state (B) model backbone structures in side view. (C) Hv1 B and Hv1 D backbone structures are superimposed. S1-S3 helices are represented by colored ribbons and S4 segments are shown as solid cylinders. (D, E) Side view overlays of Hv1 D (WT) and Hv1 E (R1H) resting-state model structures (D) or Hv1 B (WT) activated- and Hv1 E (R1H) resting-state model structures (E). Video 4 shows Hv1 B activated- and Hv1 D resting-state model structures in rotation.
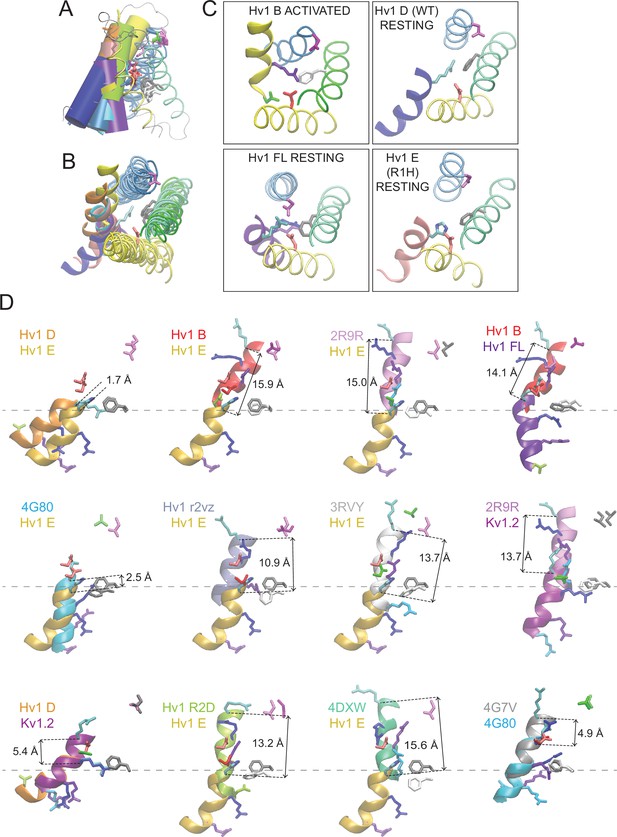
Comparisons of putative resting- and activated-state Hv1 and Ci VSD model and X-ray structures.
(A, B) Ribbon diagrams of selected VS domain model structures are viewed side-on (A) or from the extracellular space (B). S4 helices are shown as colored solid cylinders (A) or thick ribbons (B). S1-S3 helices are similarly colored in all structures (S1, yellow; S2, green; S3, blue) and S4 helices are colored differently for each structure: Hv1 B, yellow; Hv1 D, blue; Hv1 E, red; Hv1 R2D, lime green; Hv1 r2vz, orange; Hv1 FL, violet; Ci VSDU, pink; Ci VSDD, cyan. The side chains of Hv1 D112 (D1.51, red), Hv1 D185 (D3.61, magenta) and R1 (R4.47, cyan) in Hv1 D only are shown in colored licorice; Hv1 F150/Ci VSP F161 (F2.50) is colored white in activated-state structures and gray in resting-state structures. The structure of the protein backbone in inter-helical loop regions of Hv1 D only is shown as a gray line in A but omitted from B for clarity. (C) Insets show extracellular views of Hv1 B (top left), Hv1 D (top right), Hv1 FL (bottom left) and Hv1 E (bottom right). Helices and side chains are colored as in B. (D) Representations of the S4 helix (Hv1 residues 205–214; Ci VSP residues 223–232) from the indicated structures are shown as thick colored ribbons. The orientations of S4 segments relative to one another are shown as in A. S4 segments are colored as indicated by the labels: Hv1 B, red; Hv1 D, orange; Hv1 E (R1H), gold; Hv1 R2D, lime green; Hv1 r2vz, ice blue; Hv1 FL, purple; Ci VSDU, gray; Ci VSDD, cyan; 3RVY, white; 2R9R, light purple; 4DXW, green. Side chains of selected residues are shown in colored licorice: R1 (R4.47), cyan; R2 (R4.50), blue; R3 (R4.53), violet; R4 (R4.56) and N4R, aqua; N4 (N4.56), green; Hv1 D185 (D3.61), magenta; Ci VSP T201 (T3.65), green; Hv1 D112/ Ci VSP D129 (D1.51), red; Hv1 F150/ Ci VSP F161 (F2.50) side chains are white in activated- and gray in resting-state structures. Distance (in Å) measured between R1 Cα atoms in the structures shown is indicated by dashed black arrows. Dashed gray lines indicate vertical position of the indicated resting-state Hv1 F150 Cα atoms.
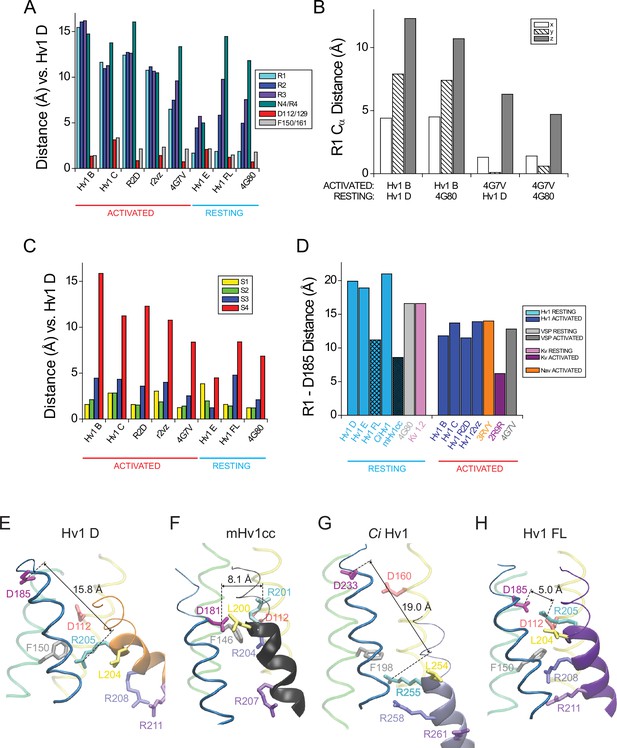
Atomic distances between backbone Cα atoms in resting and activated Hv1 model structures.
(A) Columns represent distance (in Å) measured between R1 (Hv1 R205, Ci VSP R223; cyan), R2 (Hv1 R208, VSP R226; blue), R3 (Hv1 R211, Ci VSP R229; violet), N4/R4 (Hv1 N214, Ci VSP R232; aqua), D112/129 (Hv1 D112, Ci VSP D129; red) or F150/161 (Hv1 F150, Ci VSP F161; gray) Cα atoms in Hv1 D compared to the indicated structure. Structures were collectively aligned using MultiSeq prior to RMSD measurement. (B) Columns represent differences in the 3-D (x, y, z) coordinates of R1 Cα atoms (in Å) measured between the indicated resting- vs. activated-state VS domain X-ray and model structures. The x, y and z positions of the R1 Cα atoms in Hv1 B, Hv1 D, Ci VSDU and Ci VSDD were determined after a structural alignment (MultiSeq) of the indicated pair of structures. Note that differences in the position of R1 in either Hv1 D or Ci VSDD (pdb: 4G80) resting-state structures to the Ci VSDU (pdb: 4G7V) activated-state occurs mainly in the z (i.e., vertical or normal to plane of the membrane) axis, but displacements in all 3 axes are observed when comparing the Hv1 B activated-state structure to either Hv1 D or Ci VSDD resting-state structures. (C) Columns represent distance (in Å) measured between the backbone positions of S1 (yellow), S2 (green), S3 (blue) or S4 (red) helical segments in Hv1 D and the indicated model or X-ray structure. RMSD of Cα atoms in amino acid positions (S1, yellow: Hv1, 104–119; Ci VSP, 121–136; S2, green: Hv1, 140–157; Ci VSP, 151–168; S3, blue: Hv1: 172–185; Ci VSP: 188–201; S4, red: Hv1, 205–214; Ci VSP: 223–232) are compared for Hv1 D and the indicated structures. (D) Columns represent distances (in Å) between D185 (Hv1 D3.61) or T201 (Ci VSP T3.65) and R1 Cα atoms in the indicated structure. Note that with the exception of Hv1 FL and mHv1cc, the R1-D185 distance is shorter in activated-state structures. (E–H) Hv1 D (E), mHv1cc (F), Ci Hv1 (G) and Hv1 FL (H) backbone structures are represented by colored tubes (S1, yellow; S2, green; S3, blue) or ribbons (S4: Hv1D, orange; mHv1cc, black; Ci Hv1 ice blue; Hv1 FL, purple). Selected residue side chains are shown in colored licorice using human Hv1 numbering, as indicated by labels: D112/D1.51, red; F150/F2.50, gray; D185/D3.61, magenta; R1/R4.47, cyan; R2/R4.50, blue; R3/R4.53, violet; L204/L4.46, yellow. Atomic distances (in Å) between D185 Cα (CD) and R1 Cα (CE) atoms are indicated by solid lines. Video 4 shows superimposed Hv1 D and Hv1 FL model structures in rotation.
Videos
Rotating side view of superimposed Kv1.2 resting-state, Hv1 D and Hv1 E model structures.
Protein backbone and side chains are as described in Figure 5A–D. The animation shows the structures in rotation about the vertical axis.
Rotating side view of Hv1 E and mHv1cc.
Protein backbone and side chains are as described in Figure 5—figure supplement 4A–C. Animation shows the structures in rotation about the vertical axis.
Rocking extracellular view of mHv1cc, Hv1 D and Hv1 E.
Protein backbone and side chains are as described in Figure 5—figure supplement 4A–C. The animation shows the structures viewed from the extracellular side in a rocking motion.
Rotating view of Hv1 D and Hv1 FL resting-state model structures.
S1-S3 helices are represented by colored tubes (S1, yellow; S2, green, S3, blue), S4 segments are shown as thick colored ribbons (Hv1 D, blue; Hv1 FL, violet) and loops (for Hv1 D only) are shown as thin gray tubes. Selected side chains are shown in colored licorice (D112, red; F150, gray; D185, magenta; R1, cyan; R2, blue; R3, violet; N4, green). The animation shows the structures in rotation about the vertical axis.
Rotating view of Hv1 B activated- and Hv1 D resting-state model structures.
S1-S3 helices are represented by thin colored ribbons (S1, yellow; S2, green, S3, blue), S4 segments are shown as thick colored ribbons (Hv1 B, yellow; Hv1 D, blue) and loops are shown as thin gray tubes. Selected side chains are shown in colored licorice (Hv1 B: D112, red; F150, gray; D185, magenta; R1, cyan; R2, blue; R3, violet; Hv1 D: D112, pink; F150, white; R1, cyan; R2, blue; R3, violet; N4, green). Animation shows the structures in rotation about the vertical axis.
Tables
Numbering of selected residues in Hv1 and Shaker VS domain sequences.
Residue position | Hs Hv1 number | mHv1cc number | Ci Hv1 number | Shaker number |
---|---|---|---|---|
1.48 | V109 | V105 | V157 | I237 |
1.51 | D112 | D108 | D160 | S240 |
1.52 | A113 | A109 | S161 | I241* |
1.54 | L115 | L111 | L163 | I243 |
1.55 | V116† | V112 | V164 | F244 |
2.44 | I144 | F140 | L192 | T284 |
2.46 | I146 | I142 | I194 | C286 |
2.47 | L147 | L143 | L195 | I287* |
2.50 | F150 | F146 | F198 | F290 |
2.51 | M151 | M147 | M199 | T291 |
3.53 | V177 | I173‡ | V225 | A319 |
3.54 | V178 | V174 | V226 | I320 |
3.58 | F182 | F178 | F230 | F324 |
3.61 | D185 | D181 | D233 | L327 |
3.65 | L189 | L185 | I237 | V331 |
4.40 | L198 | L194 | I248 | M356 |
4.43 | L201 | L197 | L251 | L358 |
4.44 | I202 | I198 | V252 | A359 |
4.46 | L204 | L200 | L254 | L361 |
4.47 | R205 | R201 | R255 | R362 |
-
*Shaker I241H (I1.52) and I287H (I2.47) confer GSH (Campos et al., 2007).
-
†Functionally substitutes for D1.51 in background of D112V (Morgan et al., 2013).
-
‡Ile3.53 was transferred from Ci VSP into mHv1cc chimera (Takeshita et al., 2014).
Effects of Hv1 mutations on GAQ gating. ITAIL was measured in cells expressing the indicated constructs and VTHR was estimated by visual inspection of raw current records as described (Materials and methods). The data represent means ± SEM from determinations in the indicated number (n) of cells.
construct | GAQ VTHR (mV) | SEM | n |
---|---|---|---|
WT* | +7 | 2 | 6 |
N4R* | +17 | 3 | 4 |
R1A* | +6 | 3 | 4 |
R1H | −25.0 | 1.9 | 20 |
R1H-N4R | −20.7 | 2.2 | 14 |
D185A-R1H | +40.0 | 1.9 | 13 |
D185H-R1H | +80.0 | 3.8 | 7 |