Magnetogenetics: Problems on the back of an envelope
Many biophysicists have in their library a little book by Howard Berg called Random Walks in Biology. In this book Berg applies principles one learns in an introductory physical chemistry class to analyze processes ranging from the migration of proteins on gels to the motility of whole living cells (Berg, 1993). In addition to exploring a specific set of phenomena, the book promotes the ethos of using simple models and calculations – often so simple that they could be performed on "the back of the envelope" – to bring order to otherwise messy biological systems. This ethos gives life scientists a quantitative alternative to the qualitative empiricism that is found in many fields of modern biology.
Now, in eLife, Markus Meister of Caltech reports how the results of such calculations have led him to question a number of recent papers about the use of magnetic fields to manipulate proteins, cells and organisms (Meister, 2016). Because biological tissue normally interacts only weakly with magnetic fields, it might be possible to use magnetically-active molecules that interface with biological systems to remotely control specific physiological processes. Given the wealth of research made possible by optogenetics – in which light is used to control cells that have been genetically modified to make them sensitive to light (Adamantidis et al., 2015) – one can only imagine the possibilities afforded by an analogous “magnetogenetic” approach. The papers that Meister critiques all claim to harness iron-binding proteins to realize various aspects of magnetogenetics. However, using arguments from elementary physics, he shows that key results reported in these articles cannot be explained by the magnetic phenomena the authors say they have exploited (Figure 1).
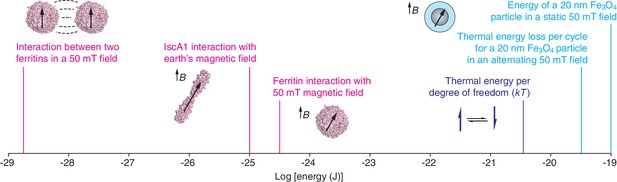
Molecular-scale magnetic interaction energies.
The energies associated with various magnetogenetic components, as estimated optimistically by Meister, are labeled in magenta. Each is orders of magnitude smaller than the thermal energy per degree of freedom at room temperature (dark blue). In contrast, based on our calculations, interactions involving magnetite particles (Fe3O4; light blue) can exceed the thermal energy (kT) at room temperature. These calculations assume that the magnetization of magnetite is 480 emu/cm3 and that the specific loss power for an individual particle is 24 fW. Note that the x-axis is logarithmic. The strength of the earth's magnetic field varies between about 25 and 65 microtesla at the earth's surface. mT: millitesla; B: magnetic field; k: Boltzmann’s constant; T: temperature.
In the first paper, Can Xie of Peking University and co-workers report that Isca1 – an iron-sulfur cluster protein that is found in Drosophila – acts like a compass needle and aligns with the Earth’s magnetic field (Qin et al., 2015). Such a "biocompass" could be a potential molecular building block for magnetogenetics. However, Meister’s calculations show that Isca1 (Cózar-Castellano et al., 2004) is not capable of acting like a compass needle. The iron content of Isca1 was proposed as a basis for magnetic effects. Certain configurations of iron give rise to molecular-scale magnetic dipoles, which interact with magnetic fields in roughly the same way a miniscule bar magnet would. But Meister points out that the smallest iron-containing species with dipoles strong enough to spontaneously orient in magnetic fields at room temperature are mineral particles with diameters of ~20 nm, each of which contains hundreds of thousands of iron atoms in very close proximity to each other. Both the quantity and closeness of the iron atoms in such nanoparticles are necessary in order for the magnetic contributions of the individual atoms to cooperate with each other sufficiently to override the randomizing effects of thermal energy (Cullity and Graham, 2008), and the 40 iron atoms in Isca1 are too few and too far apart to achieve this. Even if the atoms were close enough, Meister calculates that the interaction between the protein and the earth's magnetic field would be much too weak (by a factor of about 105) to overcome thermal fluctuations at room temperature.
Rather than searching for naturally occurring building blocks for magnetogenetics, other researchers have used genetic techniques to produce hybrid protein assemblies that contain the iron storage protein ferritin conjugated to an ion channel. Ali Güler of the University of Virginia and co-workers (Wheeler et al., 2016) studied neurons in which the ferritin was conjugated to an ion channel called TRPV4 that is activated by mechanical force (Liedtke, 2008). Güler and co-workers wanted to use magnetic fields to rotate or pull on the ferritin components, thus exerting a force that could be used to trigger the opening of the ion channel. The researchers reported that they observed electrophysiological and behavioral changes when they applied magnetic field gradients to various systems, including in vitro systems, brain slices and freely moving mice. However, Meister’s analysis indicates that the magnetic field conditions used in the study could produce forces that were, at most, nine orders of magnitude lower than the forces needed to open known mechanically-sensitive ion channels. Indeed, the forces are too weak (by several orders of magnitude) to overcome random thermal fluctuations at room temperature, and are thus unlikely to mediate magnetogenetics.
Jonathan Dordick (Rensselaer Polytechnic Institute), Jeffrey Friedman (Rockefeller University) and co-workers (Stanley et al., 2015) studied insulin-secreting cells in which the ferritin was conjugated to a temperature-sensitive ion channel called TRPV1 (Caterina et al., 1997). The basic idea was that applying an oscillating magnetic field would heat the ferritin and that the resulting increase in temperature would open the ion channel, triggering insulin release. The approach is inspired by a similar strategy, called magnetic hyperthermia, that uses oscillating fields to heat much more strongly magnetic particles, and can be used to treat cancer (Pankhurst et al., 2009). Stanley et al. reported that ion channels in mice could be activated by oscillating magnetic fields. However, Meister estimated the specific loss power – the amount of heat dissipated by a particle per gram of iron – for the ferritin nanoparticles (Fantechi et al., 2014) used in the experiments and found that it was too low to support a magnetogenetic approach.
Where does magnetogenetics go from here? Two ways forward seem plausible. The first is to continue efforts to control biological systems using magnetic species that are far more potent than Isca1 or ferritin. Indeed, two groups have already demonstrated that nanoparticles with magnetic moments ~1000 times higher than those of ferritin can be used to manipulate neural activity (Huang et al., 2010; Chen et al., 2015). Using magnetic fields to heat such nanoparticles in close proximity to temperature-sensitive ion channels should lead to a physically practical form of the technique proposed by Stanley et al. Another challenge is to deliver these nanoparticles to where they are needed: in the future, it might be possible to do this by repurposing the genetic machinery that enables certain bacterial and vertebrate species to produce their own magnetite (Johnsen and Lohmann, 2005). A second way forward might involve a critical re-examination of the experiments performed by Wheeler et al. and Stanley et al. If their cellular-level findings prove reproducible, the true mechanism that explains them might even fulfill some of the promise that magnetogenetics seemed to offer before it met the back of Professor Meister’s envelope.
References
-
Optogenetics: 10 years after ChR2 in neurons--views from the communityNature Neuroscience 18:1202–1212.https://doi.org/10.1038/nn.4106
-
Wireless magnetothermal deep brain stimulationScience 347:1477–1480.https://doi.org/10.1126/science.1261821
-
BookIntroduction to Magnetic Materials. 2nd EdnWiley: IEEE Press.https://doi.org/10.1002/9780470386323
-
hIscA: a protein implicated in the biogenesis of iron–sulfur clustersBiochimica Et Biophysica Acta 1700:179–188.https://doi.org/10.1016/j.bbapap.2004.05.004
-
The physics and neurobiology of magnetoreceptionNature Reviews Neuroscience 6:703–712.https://doi.org/10.1038/nrn1745
-
Molecular mechanisms of TRPV4-mediated neural signalingAnnals of the New York Academy of Sciences 1144:42–52.https://doi.org/10.1196/annals.1418.012
-
Progress in applications of magnetic nanoparticles in biomedicineJournal of Physics D: Applied Physics 42:224001.https://doi.org/10.1088/0022-3727/42/22/224001
-
Genetically targeted magnetic control of the nervous systemNature Neuroscience 19:756–761.https://doi.org/10.1038/nn.4265
Article and author information
Author details
Publication history
- Version of Record published: September 8, 2016 (version 1)
Copyright
© 2016, Anikeeva et al.
This article is distributed under the terms of the Creative Commons Attribution License, which permits unrestricted use and redistribution provided that the original author and source are credited.
Metrics
-
- 4,914
- views
-
- 576
- downloads
-
- 17
- citations
Views, downloads and citations are aggregated across all versions of this paper published by eLife.
Download links
Downloads (link to download the article as PDF)
Open citations (links to open the citations from this article in various online reference manager services)
Cite this article (links to download the citations from this article in formats compatible with various reference manager tools)
Further reading
-
- Physics of Living Systems
During locomotion, soft-bodied terrestrial animals solve complex control problems at substrate interfaces, but our understanding of how they achieve this without rigid components remains incomplete. Here, we develop new all-optical methods based on optical interference in a deformable substrate to measure ground reaction forces (GRFs) with micrometre and nanonewton precision in behaving Drosophila larvae. Combining this with a kinematic analysis of substrate-interfacing features, we shed new light onto the biomechanical control of larval locomotion. Crawling in larvae measuring ~1 mm in length involves an intricate pattern of cuticle sequestration and planting, producing GRFs of 1–7 µN. We show that larvae insert and expand denticulated, feet-like structures into substrates as they move, a process not previously observed in soft-bodied animals. These ‘protopodia’ form dynamic anchors to compensate counteracting forces. Our work provides a framework for future biomechanics research in soft-bodied animals and promises to inspire improved soft-robot design.
-
- Physics of Living Systems
Despite long-running efforts to increase gender diversity among tenured and tenure-track faculty in the U.S., women remain underrepresented in most academic fields, sometimes dramatically so. Here, we quantify the relative importance of faculty hiring and faculty attrition for both past and future faculty gender diversity using comprehensive data on the training and employment of 268,769 tenured and tenure-track faculty rostered at 12,112U.S. PhD-granting departments, spanning 111 academic fields between 2011 and 2020. Over this time, we find that hiring had a far greater impact on women’s representation among faculty than attrition in the majority (90.1%) of academic fields, even as academia loses a higher share of women faculty relative to men at every career stage. Finally, we model the impact of five specific policy interventions on women’s representation, and project that eliminating attrition differences between women and men only leads to a marginal increase in women’s overall representation—in most fields, successful interventions will need to make substantial and sustained changes to hiring in order to reach gender parity.