Unconventional secretory processing diversifies neuronal ion channel properties
Figures
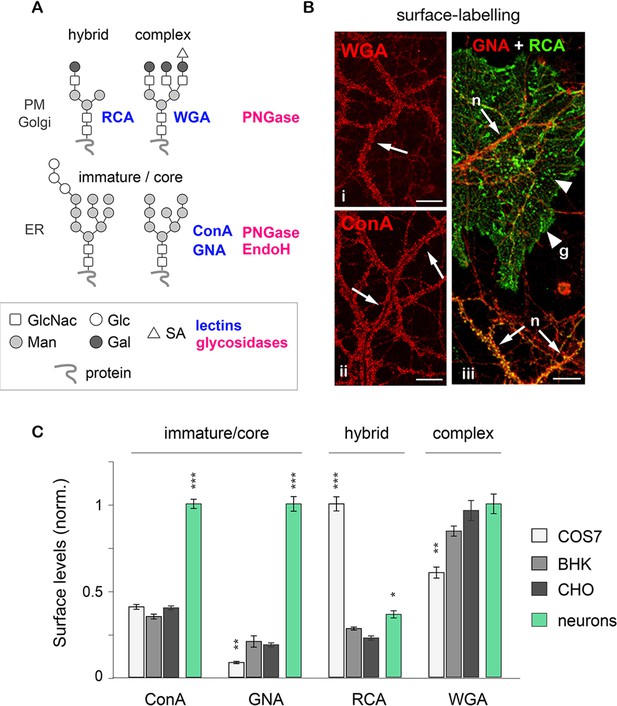
Core-glycosylated proteins are abundant at the neuronal surface.
(A) The binding to specific lectins and the sensitivity to distinct glycosydases distinguish different N-glycans. Hybrid and complex N-glycans detected at the plasma membrane (PM) and the Golgi apparatus (Golgi) bind ricin agglutinin (RCA) and wheat germ agglutinin (WGA), respectively, and are hydrolyzed by PNGase. In contrast, core-glycosylated N-glycans are usually specifically localized to the endoplasmic reticulum (ER), bind concanavalin A and galanthus nivalis agglutinin (GNA) and are hydrolyzed by both PNGase and EndoH. (B) Cultured hippocampal neurons (DIV 40) after surface labeling with ConA, GNA, WGA (red) and RCA (green) to identify core-glycosylated (ConA, GNA), complex (WGA) and hybrid (RCA) glycans, respectively. In iii, 'n' and 'g' mark neurons and glial cells, respectively. Note the high reactivity of the neuronal surface to ConA, WGA, GNA and RCA (arrows). In contrast, in iii note the low and high reactivity of glial cells to GNA and RCA, respectively, resulting in a 'green-only' appearance (arrowheads). Scale bars 25 µm. (C) Surface levels of ConA, GNA, RCA and WGA reactive glycans in fibroblasts (COS, white; BHK, light grey; and CHO, dark grey) and in neurons (green). Mean ± SEM. N=27 to 132 cells in 2 experiments. *p<0.05; **p<0.01, ***p<1x10−4; Kruskal-Wallis’s multiple comparison test.
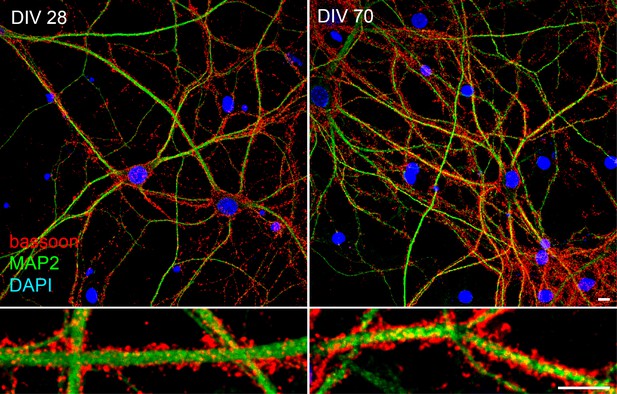
Viable hippocampal neurons can be maintained in culture for more than two months.
Labeling of dendrites (MAP2, green), synapses (bassoon, red) and nuclei (DAPI, blue) in DIV28 and DIV70 neurons documenting the viability of DIV 40 and older neurons as in Figure 1. Scale bar 10 μm.
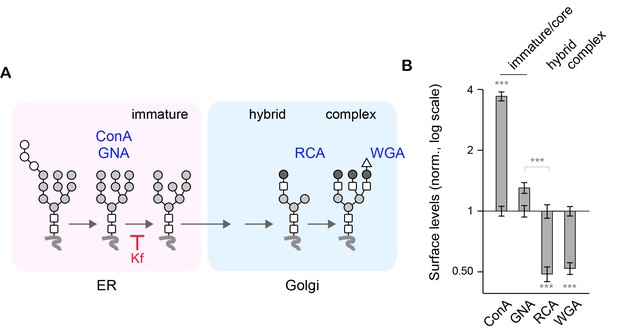
Blocking the maturation of core-glycans with kifunensine increases the surface levels of ConA- and GNA-reactive glycans but decreases RCA- and WGA-reactive glycans.
(A) Simplified representation of the N-glycosylation pathway and site of action of kifunensine (Kf). (B) Surface levels of ConA-, GNA-, RCA- and WGA-reactive glycans in neurons (DIV 14–18) treated with Kf (5 μM) for two or three days. Shown are values in neurons treated with Kf normalized to control values (log 2 scale). Kf increases ConA and GNA levels but decreases RCA and WGA. N=53–81 cells in 2 experiments for each drug. ***p<1x10−4; Dunn’s multiple comparison test.
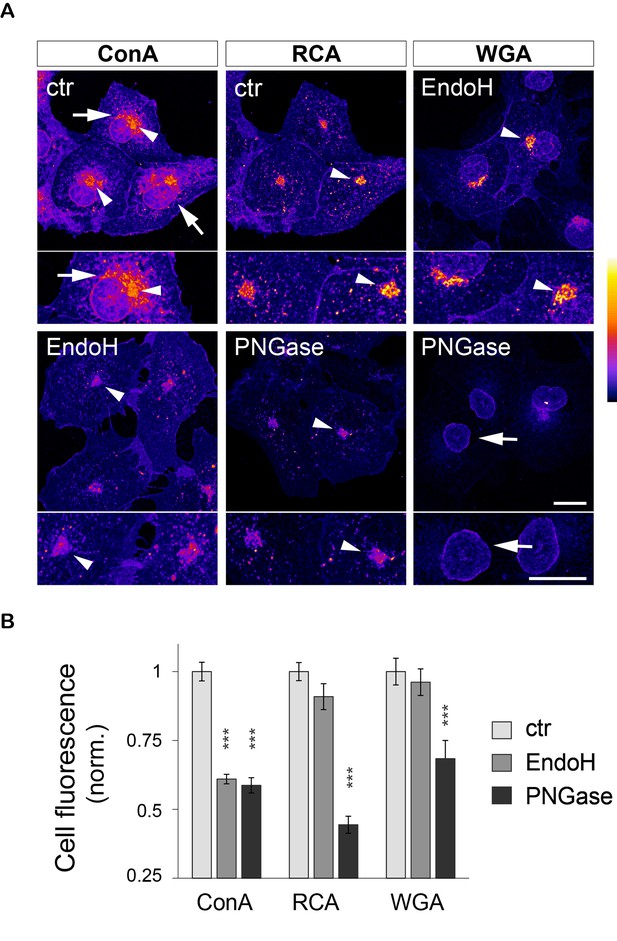
Intracellular ConA-, RCA- and WGA-reactive glycans are localized in distinct organelles in neurons.
Images (single confocal planes) of neurons (DIV 28) after labeling with ConA or WGA (red), RCA (green), DAPI (blue) and anti-MAP2 antibodies (cyan). ConA labels structures that have the morphological characteristics of endoplasmic reticulum (ER, arrows). In contrast, WGA labels the nuclear envelope (NE, crossed arrow) and Golgi-like compartments (arrowhead). RCA labels pleiomorphic structures (arrowheads), which are distinct from ConA-reactive structures but partly colocalize with some of theWGA-reactive compartments (other than the NE). Scale bar 10 or 5 μm.
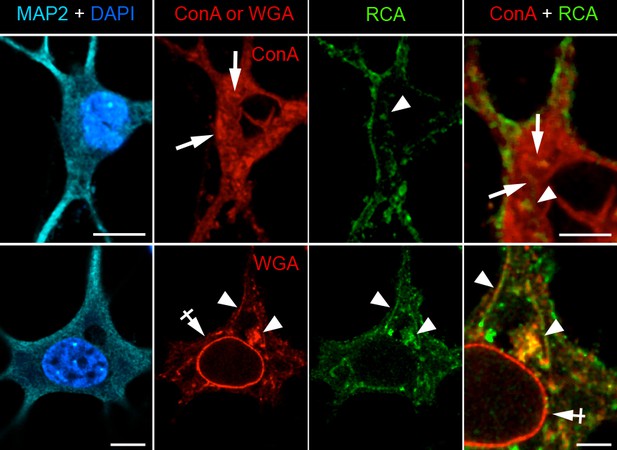
ConA-, RCA- and WGA-reactive glycans are localized in distinct organelles and display contrasting sensitivities to EndoH and PNGase in COS cells.
(A) Subcellular distribution (maximal intensity projections of confocal stacks) and (B) average levels of ConA-, RCA- and WGA-reactive glycans in COS 7 cells in control conditions (light grey) or after glycan removal by EndoH (darker grey) or PNGAse (darkest grey). ConA labels the endoplasmic reticulum (ER, arrows in A) and to a lesser extent the Golgi apparatus (GA, arrowheads in A). Pretreatment with PNGase or EndoH (only shown for Endo H in A) strongly reduces the labeling of the ER, resulting in a marked decrease of total ConA levels (B). RCA and WGA label the GA (RCA and WGA, arrowheads in A) and the nuclear envelope (WGA, arrows in A). EndoH has no effect on the intensity of the labeling with these two lectins (compare RCA and WGA labeling in control cells and in cells treated with EndoH, respectively, and see B). In contrast, PNGase strongly reduces the labeling of the GA (arrowheads in A), resulting in decreased levels of RCA- and WGA-reactive glycans. Note that the quantification in B was done without fluorescence thresholding, partly explaining the relatively high background signal remaining after enzymatic digestion. In A, scale bar = 10 μm. Shown in B is mean ± SEM, N=19–36 cells. ***p<1x10−4; Dunn’s multiple comparison test.
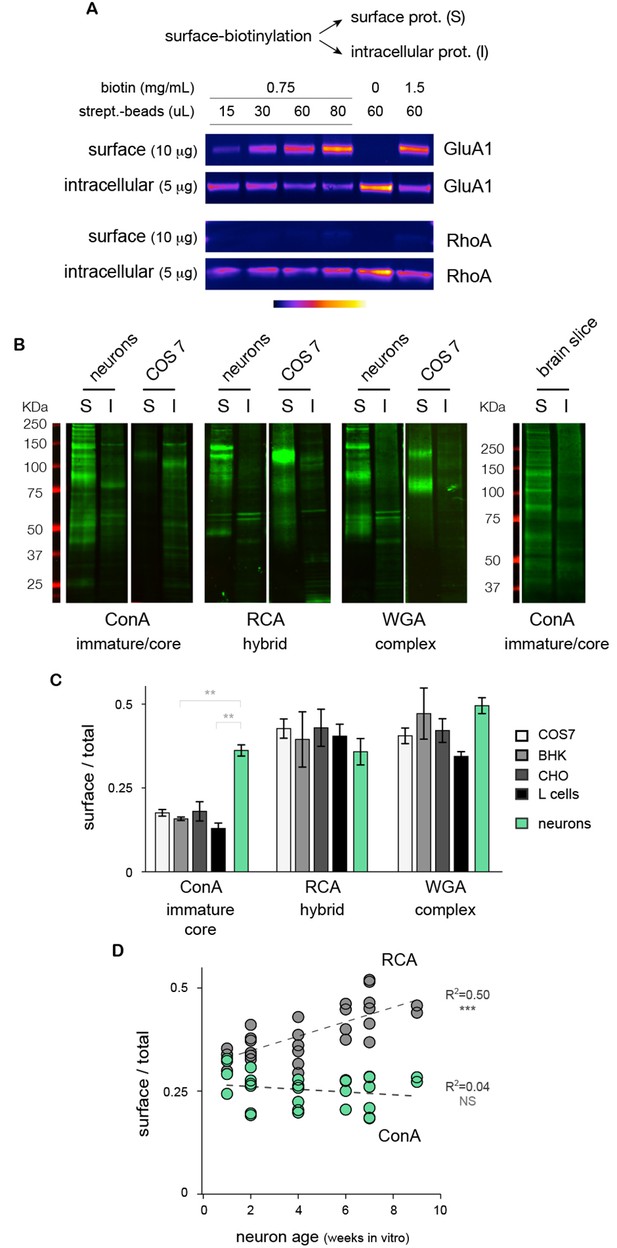
Core-glycosylated proteins are expressed at the neuronal surface at levels similar to hybrid and complex N-glycosylated proteins.
(A) Isolation of surface ion channel (GluA1) versus cytoplasmic proteins (RhoA) after surface biotinylation and purification with varying amounts of NHS-SS-biotin and streptavidin-beads. (B) Surface (S) and intracellular (I) proteins prepared from neurons, COS7 cells and acute brain slices after labeling (far western blots) with ConA, RCA and WGA. For each condition, 15 and 5 μg of proteins were loaded for S and I fractions, respectively. Note the high levels of ConA reactive species in cultured neurons and in brain slices. (C) Relative surface expression (surface / total signal) of ConA, RCA and WGA reactive species in cultured neurons (green) and different non-neuronal cell types (COS, white; BHK, light grey; CHO, darker grey; L-cells, black) showing that the high surface expression of core N-glycans is specifically observed in neurons while other cell types show low levels of core glycosylation but higher levels of hybrid and complex glycosylation. Mean ± SEM and individual data points. N=4 in 2 experiments. * p<0.05; ** p<0.01, Dunn’s multiple comparison test. (D) Relative surface expression of ConA- and RCA-reactive glycans in neurons from one to nine weeks in vitro, showing stable levels of surface core-glycosylation and a developmental increase in the surface expression of hybrid glycans. N= 27–28 dishes in 3 independent experiments. ***p<1x10−4; Pearson’s r test.
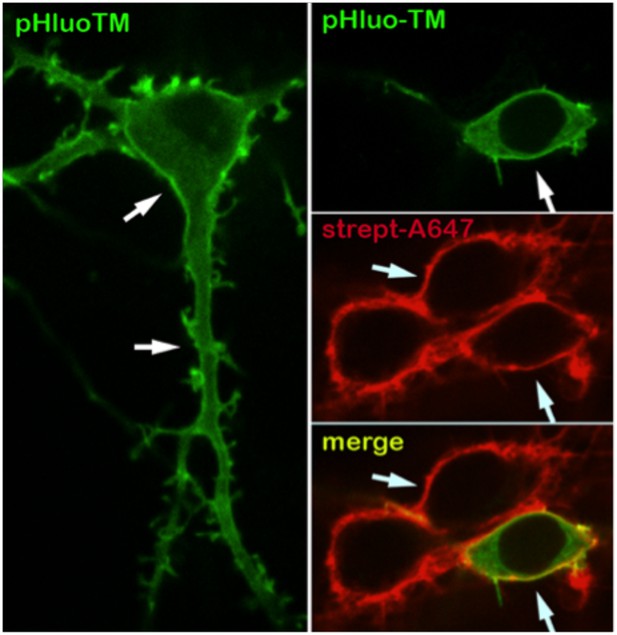
The neuronal plasma membrane is intact after surface biotinylation.
Intrinsic fluorescence (single confocal plane image) of pHluo-TM (green), a transmembrane protein marker that selectively exhibits fluorescence at the plasma membrane (and to a lesser extent the endoplasmic reticulum), and streptavidin-Alexa647 fluorescence (red) after surface biotinylation of 15 DIV neurons, showing the selective biotinylation and the integrity of the neuronal surface (arrows).
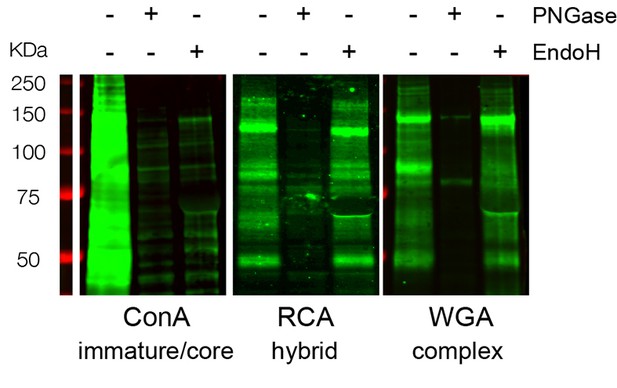
ConA-, RCA- and WGA-reactive glycans assessed by far-western blotting display distinct and specific sensitivities to PNGAse and EndoH.
Total neuronal extracts labeled with ConA, RCA or WGA after or without pretreatment with PNGase or EndoH showing the specificity of the labeling. In contrast to ConA reactivity that is abolished by either EndoH or PNGase treatment, RCA and WGA reactivities are only abolished by PNGase.
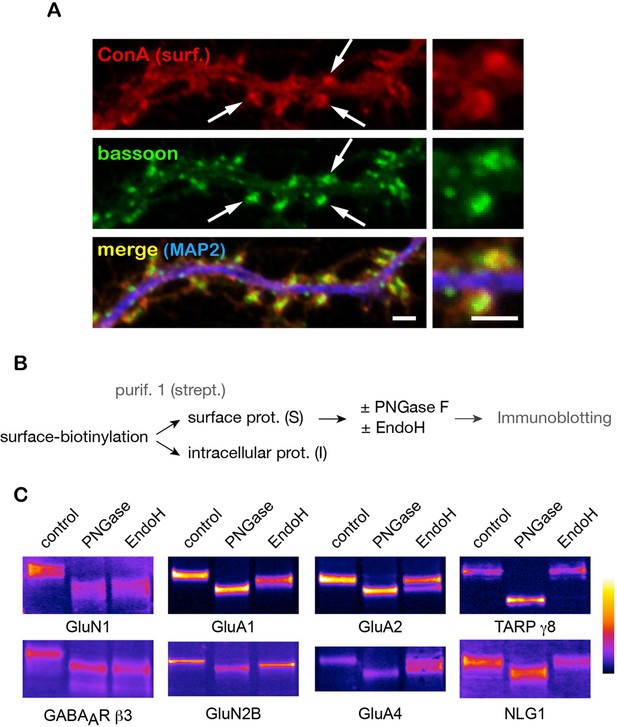
A number of key neuronal surface proteins display unconventional N-glycosylation profiles.
(A–C) Synaptic localization of ConA reactive species and core-glycosylation of surface excitatory neurotransmitter receptors. (A) Fluorescent micrographs of cultured hippocampal neurons (DIV 26) after labeling of surface core-glycosylated proteins (ConA, red) and presynaptic terminals (bassoon immunoreactivity, green, shown together with MAP2-IR in blue) showing the presence of core-glycosylated proteins at synapses. Scale bar 5 μm. (B,C) Purification scheme (B) and migration profiles (C, immunoblots) of a group of surface glutamate receptors and associated proteins after PNGase and EndoH treatment. Note the complete EndoH sensitivity of GluN1 and GluA1, the partial sensitivity of GluA2 and GluA4 and the lack of sensitivity of TARP γ8 and neuroligin 1 (NLG1).
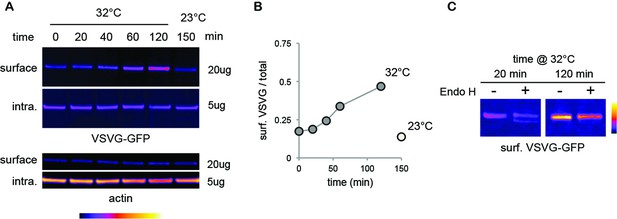
Standard mature surface N-glycans are typically insensitive to EndoH.
9AB) Immunoblots (A) and relative surface expression (B, surface / total protein) of thermosensitive vesicular stomatitis virus glycoprotein (VSVG-GFP) (Presley et al., 1997) in COS 7 cells at various times after release (39–32°C shift) of a temperature-dependent blockade of VSVG exit from the endoplasmic reticulum. Note the progressive accumulation of VSVG at the cell surface at permissive temperature (32°C), but not after a temperature-dependent blockade (23°C)[(Matlin and Simons, 1983)] of post-Golgi transport. (C) Contrasting sensitivity to EndoH of surface VSVG at an early (20 min) or later (120 min) phase after ER-exit release. Note the complete lack of sensitivity of VSVG as mature proteins accumulate at the cell-surface.
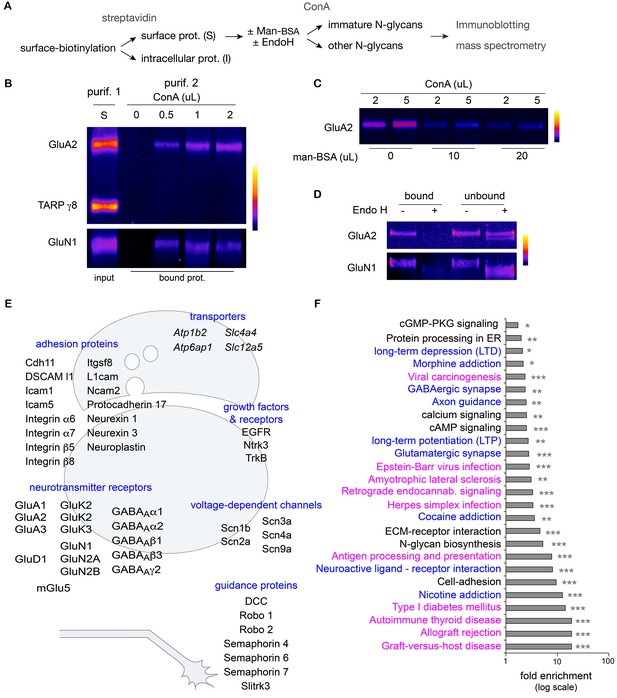
Hundreds of surface neuronal membrane proteins are core-glycosylated.
Purification scheme (A) and immunoblots (B–D) showing the isolation of core-glycosylated N-glycans of the neuronal plasma membrane. (B) Presence of GluA2 and GluN1, but not TARP γ8, among proteins binding to ConA (vol. in µL). The lane 'S' shows GluA2, GluN1 and TARP γ8 immunoreactivity in the surface fraction that was used for the experiment. (C) Incubation of surface proteins with ConA in the presence of mannose-BSA (vol. in µL) abolishes GluA2 binding to ConA. (D) Bound and unbound fractions after purification with ConA in control conditions or after protein digestion with EndoH. Core-glycan removal with EndoH abolishes GluA2 and GluN1 binding to ConA. (E,F) Mass spectrometry of proteins purified as in A. (E) Schemes of a synapse and a growth cone highlighting examples of core-glycosylated surface neuronal proteins of particular physiological relevance for synaptic function and neuron development. (F) Fold enrichment of N-glycoproteins included in the indicated pathways in core-glycosylated surface proteins versus total proteins. Highlighted in blue and in purple are proteins of particular relevance for neuronal functions or autoimmune diseases and cancer, respectively. *p<0.05, **p<0.01, ***p<1x10−4, hypergeometric test.
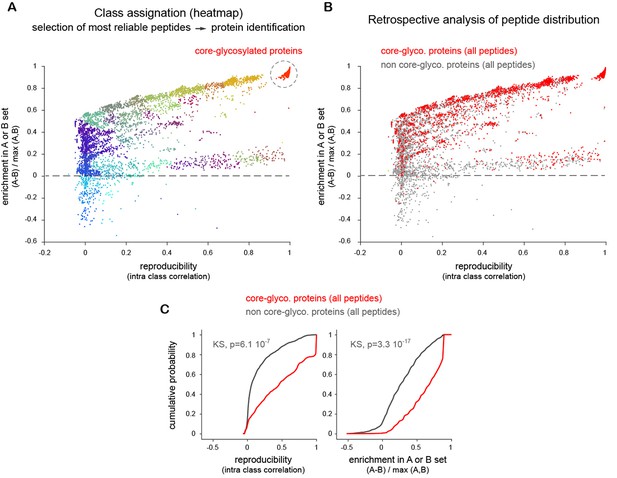
Evaluation of mass spectrometry data part 1.
Enrichment of peptides from proteins in the A (w/o EndoH) and B (w EndoH) sets as a function of repeatability across experiments and technical replicates (see Materials and methods). (A) Identification of high confidence core-glycosylated proteins. Plot of normalized peptide intensities (label free quantification) in samples without ('A', target core-glycosylated) or with prior treatment with Endo H ('B', background) as a function of detection reproducibility (intra class correlation) across multiple technical replicates and independent experiments. Shown in colors are the various groups detected after hierarchical clustering. Note the clear separation of peptides close to y=0 (equally distributed in A and B) and y=1 (only found in A) as reproducibility increases. Shown in red are peptides with the highest enrichment and reproducibility which were used to identify 'core-glycosylated' proteins. (B–C) Retrospective analysis of all the peptides originating from proteins identified in high-confidence core-glycosylated group. (B) Core-glycosylated protein peptides (red) versus all the other peptides (grey), plotted as in A. (C) Distribution (cumulative probability) of core-glycosylated protein peptides (red) versus all the other peptides (grey) showing the more reliable occurrence of core-glycosylated proteins and their sensitivity to EndoH. KS, Kolmogorov-Smirnov’s test.
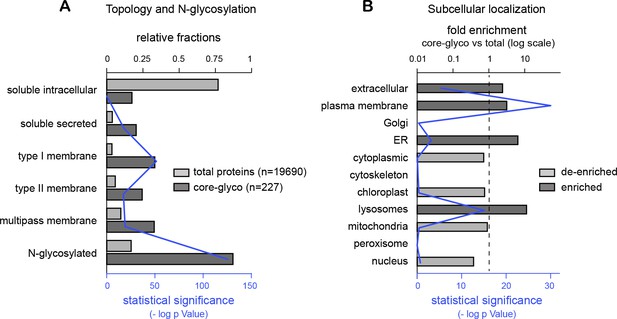
Evaluation of mass spectrometry data part 2.
(A) Enrichment for secreted and transmembrane proteins among identified surface core-glycosylated proteins. Relative frequencies (solid bars) of soluble (intracellular or secreted), transmembrane and predicted N-glycoproteins among 'total' hippocampal proteins and 'core-glycosylated' surface proteins. Shown in blue (thin line) is the statistical significance (-log p value) of the difference between the two groups for the indicated category. (B) Fold enrichment of core-glycosylated proteins with annotations associated to the indicated subcellular compartments, and statistical significance (-log p value). Note the enrichment of cell-surface, secreted, lysosomal and endoplasmic reticulum proteins, but not Golgi proteins, among the core-glycosylated proteins. ***p<1x10−4; Hypergeometric test.
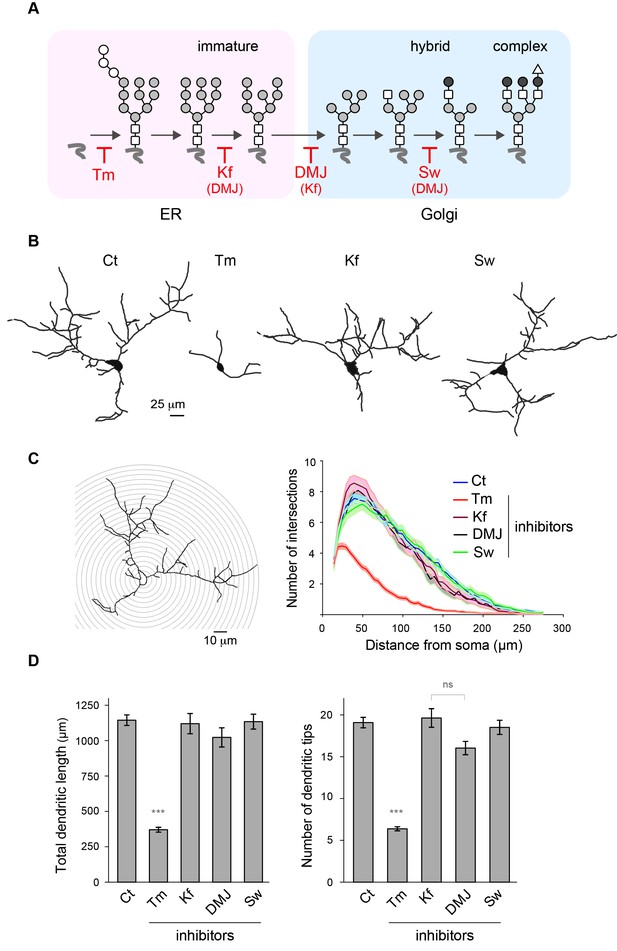
Mature N-glycans are not required for normal dendritic development.
(A) Simplified representation of the N-glycosylation pathway and site of action of the glycosylation inhibitors tunicamycin (Tm), kifunensine (Kf), 1-deoxymannojirimycin (DMJ) and swainsonine (Sw). (B–C) Camera lucida drawings (B) and/or Sholl analysis (C) of the dendrites of hippocampal neurons (DIV 11) after a three day exposure to vehicle (DMSO, Ct), Tm (1.2 µM), Kf (5 μM), DMJ (75 μM) or Sw (88 μM). (D) Total dendritic length (left panel) and branch tip numbers (right panel) in neurons treated with vehicle (Ct), Tm, Kf, DMJ or SW. Mean ± SEM. N=31 to 137 cells in 2–6 independent experiments. ***p<1x10−4; Kruskal-Wallis/Dunn’s multiple comparison test. In B–D, note that while Tm strongly impairs dendritic growth and branching, blocking the maturation of N-glycans in the ER or the GA has no detectable effect on dendritic development.
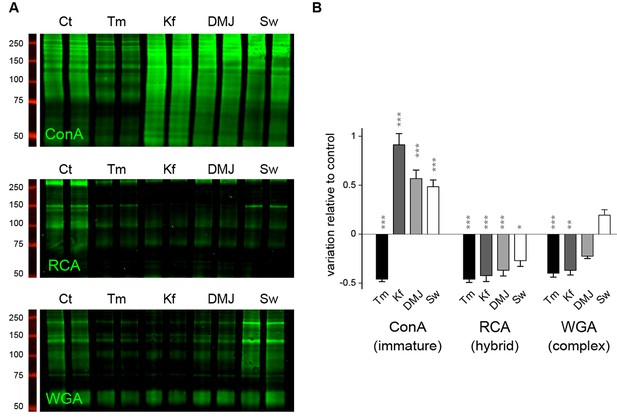
Other examples of dendrites after treatment with the drugs described in Figure 5A.
https://doi.org/10.7554/eLife.20609.017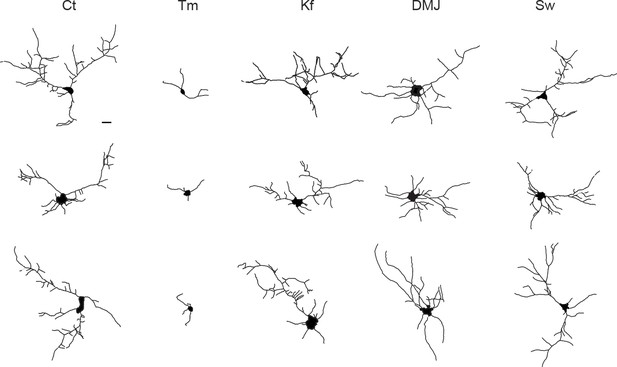
The lack of effect of specific N-glycosylation inhibitors on dendritic development is not due to a lack of activity or to non-specific effects on the glycosylation pathways.
(A) Blots and (B) surface levels of immature/core-glycans (ConA), hybrid glycans (RCA) and complex/mature glycans (WGA) in neurons (DIV 7–10) treated for three days with Tm, Kf, DMJ or Sw as in Figure 5. Core-glycans are decreased by Tm but are increased by Kf and, to a lesser extent, DMJ and Sw. In stark contrast, hybrid glycans are decreased by the 4 drugs. Mature N-glycans are decreased by Tm, Kf and DMJ, but slightly increased by Sw. N=8–12 dishes in 4–6 experiments. *p<0.05; **p<0.01, ***p<1x10−4; Dunn’s multiple comparison test.
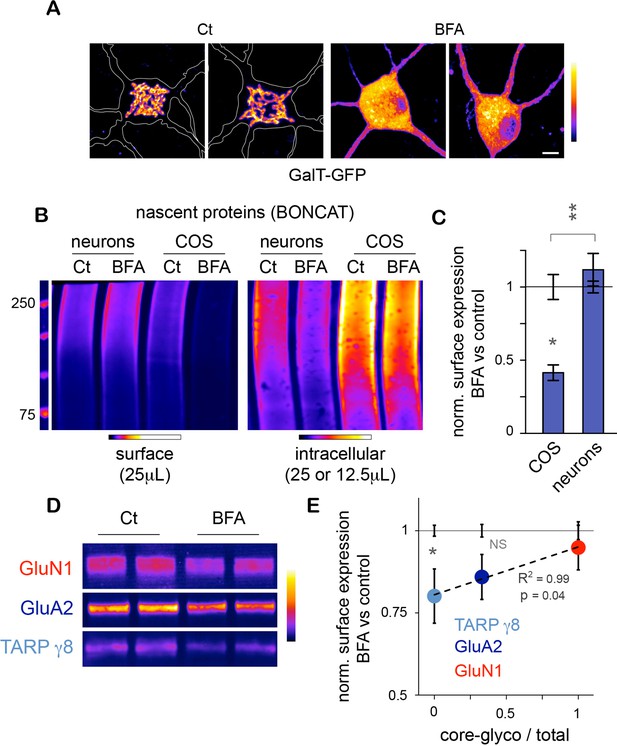
Unconventional secretory processing of core-glycosylated surface proteins.
(A) GalT-GFP expression in neurons showing the morphology of the somatic Golgi apparatus after a 2 hr long exposure to vehicle (DMSO; control, Ct) or 2.5 μg/mL brefeldin A (BFA). Scale bar 5 μm. (B–C) Immunoblots (B) and surface or intracellular levels (C) of nascent proteins metabolically labeled for 120 to 150 min by bioorthogonal non-canonical aminoacid tagging (BONCAT) in COS cells and in neurons (DIV 25–26) in the absence (control or Ct) or in the presence of 2.5 μg/mL BFA. Note the strong effect of BFA on the surface expression of nascent proteins in COS cells and the relative lack of effect in neurons. N=5 to 7 dishes in 2–3 experiments. *p<0.05, **p<0.01; Dunn’s multiple comparison test. See also Figure 6—figure supplement 1. (D) Immunoblot of surface GluN1, GluA2 and TARP γ8 after a 6–7 hr exposure to vehicle (Ct) or BFA, showing the reduced surface levels of TARP γ8, GluA2 and to a lesser extent GluN1 to the cell surface after disruption of the Golgi apparatus. (E) Sensitivity to BFA (BFA / control) as a function of core-glycosylation levels (core-glycosylated fraction / total). Note the selective effect of BFA on mature proteoglycans (low core glycosylation / total ratio). Mean ± SEM. N=10–11 in 4 experiments. *p<0.05, ANOVA/Tukey’s multicomparison test.
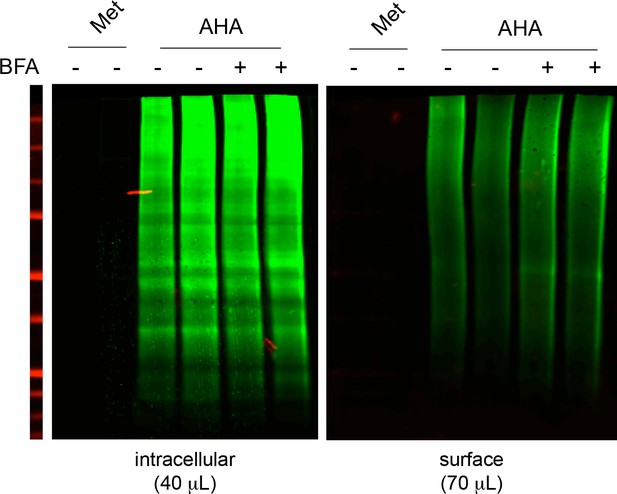
Nascent neuronal intracellular and surface proteins (BONCAT) after a 5-hr metabolic labeling with AHA or Methionine (Met) in the presence or absence of BFA.
DIV19 neurons. Note the absence of signal in neurons incubated with Methionine (which for surface proteins demonstrates the absence of residual biotin after elution from streptavidin beads during their purifcation) and the lack of detectable effect of BFA on the levels of surface nascent proteins.
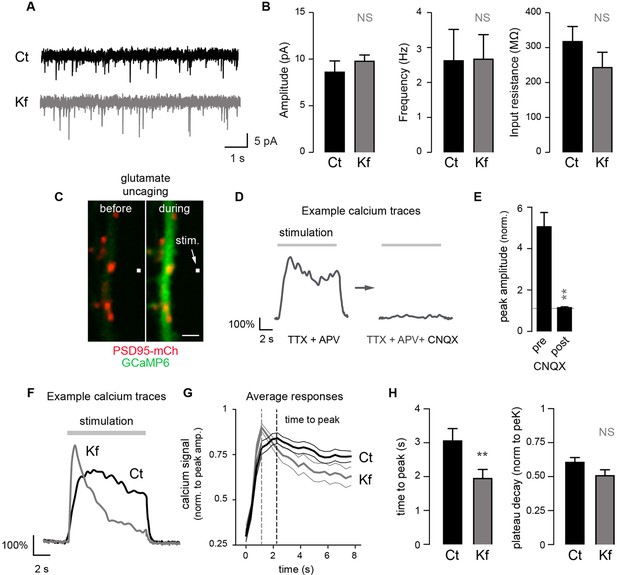
Blocking the maturation of N-glycosylation in the ER does not impair synaptic transmission and modulates the properties of postsynaptic AMPA receptors.
(A) Example traces of miniature excitatory postsynaptic currents (mEPSCs) and (B) average mEPSC amplitude and frequency and input resistance (Mean ± SEM) in control (Ct) neurons (DIV 20–21) and in neurons exposed to Kf (48 hr prior to recording) showing the lack of effect of Kf on synaptic transmission. N = 10 and 7 cells for Ct and Kf, respectively, in 3 independent experiments. NS, not significant, Mann-Whitney’s test. (C) Pictures of a synaptic marker (PSD-mCh, red) and a calcium indicator (GCaMP6, green, DIV14 neuron) before and after local glutamate uncaging at the position indicated by a white box (arrow). (D) Representative calcium traces and (E) average responses (peak amplitude normalized to baseline) induced by repetitive glutamate uncaging in individual dendrites (14 DIV neurons) before and after inhibition of AMPA receptors with CNQX. Note the complete block of the calcium signal by CNQX. N= 5 in 2 experiments. **p<10–1; Mann-Whitney’s test. (F) Example calcium trace, (G) average responses ± SEM (normalized to peak amplitude) and (H) time-to-peak and plateau decay in control neurons or in neurons exposed to Kf (48 hr prior to recording). In G and H, note the faster onset of postsynaptic responses in neurons treated with Kf. N= 29–31 (time to peak) or 22 (plateau decay) in 3 experiments. **p<10–1; Mann-Whitney’s test.
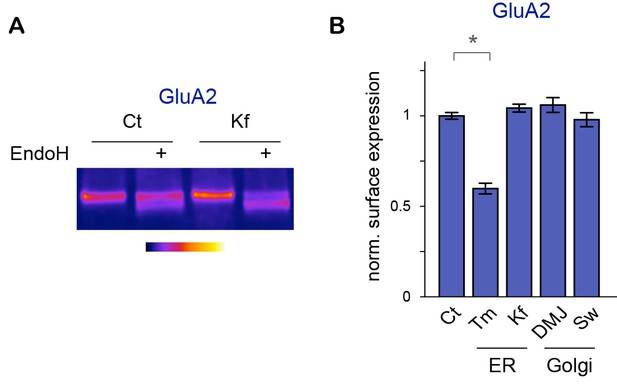
Core-glycosylation regulates GluA2 surface expression.
Effects of ER (TM, Kf, DMJ) or Golgi (DMJ, Sw) N-glycosylation inhibitors (see Figure 5A) on the glycosylation profile and surface expression of GluA2. (A) GluA2 before or after digestion with EndoH in control neurons (Ct) and in neurons (DIV 24–27) exposed to Kf for two days, showing the effect of Kf on the glycosylation profile of surface proteins. Note the decreased intensity of the EndoH-insensitive (upper) band and the converse increased intensity of the EndoH-sensitive (lower) band. (B) Average surface levels of these proteins in neurons after a two-days-exposure to DMSO (control, Ct), tunicamycin (Tm, 1,2 μM), Kifunensine (Kf, 5 μM), 1-deoxymannojirimycin (DMJ, 75 μM) or swainsonine (Sw, 88 μM). Tm reduces the surface expression of GluA2. In contrast, Kf, DMJ and SW have no detectable effect on the surface expression of the protein. Mean ± SEM and individual data points; N=4–8 samples from 2–5 independent experiments. *p<0.05; Kruskal-Wallis/Dunn’s multiple comparison test.
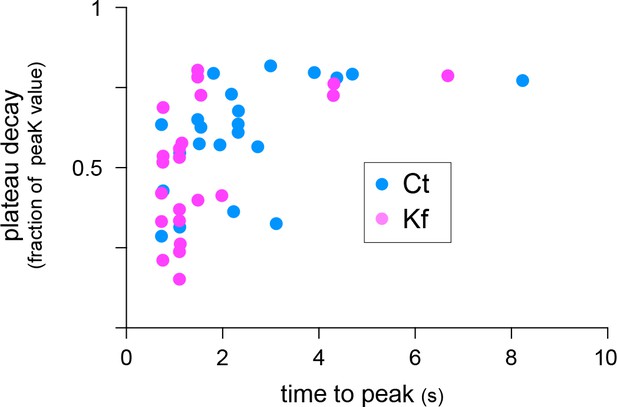
Decay plateau (fraction of peak amplitude) as a function of time to peak (s) for synapses in control neurons (Ct, blue) or in neurons treated with Kf (pink).
Note higher proportions of synapses with short time to peak and rapid decay in Kf. N=22 synapses in 3 experiments.
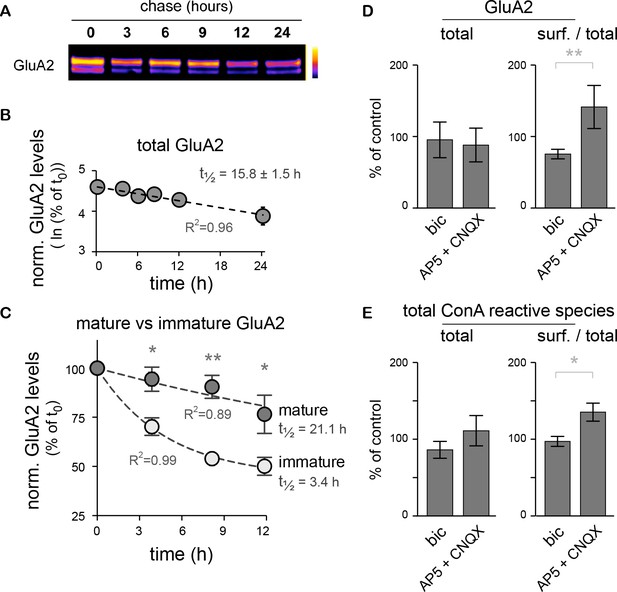
The core-glycosylation of surface proteins may accelerate their turnover and is regulated by synaptic activity.
(A) Immunoblot of biotinylated-GluA2 at various chase-times (hours) after surface biotinylation. Surface proteins were deglycosylated with EndoH to allow the distinction of mature versus core-glycosylated receptors. (B) Decay of total biotinylated GluA2 levels (log scale, n=2–4 experiments). (C) Decay of mature versus core-glycosylated GluA2. Mean ± SEM; N=6–8 in 4 experiments. Note the shorter lifetime of core-glycosylated receptors. Protein half-lifes are indicated in hours. *p<0.05, **p<0.01, ANOVA/Tukey’s multiple comparison test. (D–E) Total and relative surface expression (surf. / total) of GluA2 (D) or ConA-reactive glycans (E) after treatment with bicuculline (bic) or CNQX plus AP5 (CNQX + AP5). Bic and CNQX + AP5 levels normalized to control values. Mean ± SEM. N=7 in 4 experiments. *p<0.05, **p<0.01, Mann-Whitney test.
Additional files
-
Supplementary file 1
Tables.
(A) Core-glycosylated surface proteins (protein groups and protein IDs). (B) Core-glycosylated surface proteins (protein groups cross-referenced with typical LFQ). (C) Core-glycosylated surface proteins (protein IDs, predicted topology, subcellular localization and N-glycosylation sites). (D) Input proteins. Proteins in total hippocampal extracts and/or in A or B groups displayed as in Table 3. (E) KEGG pathways. Top 25 functional classes for all proteins or predicted glycoproteins in core-glycosylated surface proteins versus total proteins. (F) Statistical reporting. Results of the statistical tests used for the indicated figures.
- https://doi.org/10.7554/eLife.20609.025