Craniosynostosis: An epistatic explanation
The skull consists of several bony plates that are joined together at cranial sutures, and progenitor cells that reside in these sutures have a crucial role in supporting the rapid growth of new bone to accommodate the growing infant brain. However, in approximately 1 in 2500 babies the cranial sutures fuse together prematurely (either just before birth or shortly afterwards), resulting in a skull deformity known as craniosynostosis (Wilkie et al., 2010). In this condition, the bones of the skull cannot grow quickly enough to keep up with the growing brain, resulting in increased pressure inside the skull. If craniosynostosis is left untreated, it can result in a loss of vision, hearing problems and chronic headaches.
Although some cases of craniosynostosis have been linked to a number of genetic mutations, 70% of observed cases still have no known genetic cause (Twigg and Wilkie, 2015). A form of craniosynostosis called non-syndromic midline (metopic and sagittal) craniosynostosis accounts for half of all reported cases (Greenwood et al., 2014; Figure 1), but very few of these cases have been associated with genetic mutations. Now, in eLife, Richard Lifton and colleagues at Yale University School of Medicine and Craniosynostosis and Positional Plagiocephaly Support, Inc. – including Andrew Timberlake as first author – report that two genetic mutations, each of which has little impact on its own, can result in devastating outcomes when they are both present (Timberlake et al., 2016).
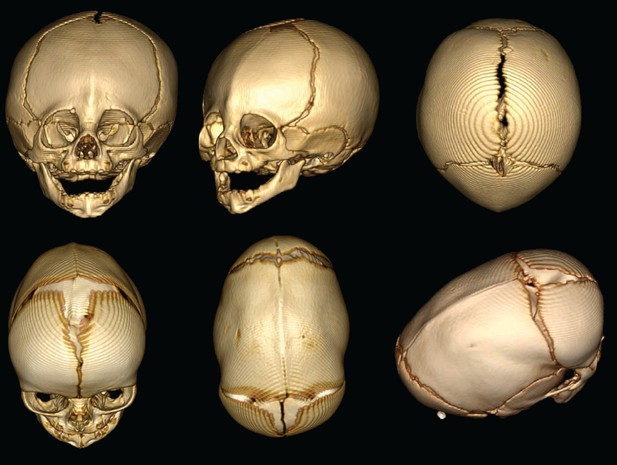
3D reconstructions of the skull shapes that result from two types of non-syndromic craniosynostosis.
Top row: In metopic craniosynostosis, the metopic suture that passes down the middle of the forehead fuses prematurely. This results in a narrow forehead that forms with a ridge down the suture. In an attempt to compensate, the skull grows in a way that pushes the forehead forward. Bottom row: sagittal craniosynostosis results from the premature fusion of the sagittal suture along the top of the skull. As a result, the skull stays narrow and extends toward the front and back to compensate.
Genome-wide association studies have identified several single nucleotide polymorphisms that are associated with non-syndromic midline craniosynostosis (Justice et al., 2012). The most significant of these mutations is located close to the gene for a bone morphogenetic protein (BMP) called BMP2, so this site might act as an enhancer to increase the production of BMP2 (Justice et al., 2012). BMP is known to be important in bone development (Urist, 1965), and previous studies have suggested that altered BMP signaling leads to craniosynostosis (Jabs et al., 1993).
It has been suggested that the sporadic occurrence of non-syndromic craniosynostosis is due to de novo (non-inherited) genetic mutations and/or because these mutations do not consistently produce the same symptoms. To identify genetic mutations associated with midline craniosynostosis, Timberlake et al. sequenced the whole exome (that is, the part of the genome formed by exons) of 191 people who were the first in their family to display the symptoms of non-syndromic midline craniosynostosis; they also sequenced the exomes of both parents of 132 of these volunteers. The sequences revealed a number of different de novo mutations in the gene that encodes SMAD6, a molecule that is known to inhibit BMP signaling. Most of the mutations prevent the SMAD6 protein from working.
Timberlake et al. found that SMAD6 mutations produce craniosynostosis in only 9% of cases. However, when an individual has both the SMAD6 mutation and the BMP2 risk allele (which on its own leads to the disorder in just 0.08% of cases), craniosynostosis occurs 100% of the time. Thus, these findings clearly indicate that epistatic interactions – interactions where the effect produced by a given gene depends on the presence of other genes – of rare (SMAD6) and common (BMP2) gene variants are a defining feature of the cause of midline craniosynostosis. As a result, these genetic mutations are likely to account for as many cases of craniosynostosis as the single mutation in a gene called FGFR2 that is currently known as the most frequent cause of craniosynostosis.
Timberlake et al. also show that there is no epistatic interaction between the mutant SMAD6 variant and the other mutation that is known to be associated with midline craniosynostosis (a single nucleotide polymorphism in BBS9; Justice et al., 2012). This finding further supports the hypothesis that an increase in BMP signaling is likely to underlie the symptoms of craniosynostosis.
BMPs can cause bone to form in abnormal places (Urist, 1965), so it is possible that the symptoms of craniosynostosis are caused by increased bone formation "filling in" the cranial suture and depleting the numbers of progenitor cells found there. Bone forms as a result of these osteoprogenitor cells developing into cells called osteoblasts, which secrete the materials that form bone. However, the story may not be so simple, because if increased BMP signaling occurs only in osteoblasts, mice do not develop the symptoms of craniosynostosis (Komatsu et al., 2013). Instead, an increased rate of cell death in the osteoprogenitor cell population seems to be the primary cause of craniosynostosis in mutant mice that exhibit excessive BMP signaling. To support this notion, mutant mice treated in the womb with drugs that prevent cell death do not develop skull deformities (Hayano et al., 2015). The obvious next steps are to understand the cellular mechanisms that cause cranial sutures to fuse prematurely, and to confirm whether human patients with mutations in the Smad6 and BMP2 genes experience increased cell death in the sutures.
At present the only option for treating craniosynostosis is repeated reconstructive surgery: however, the work of Timberlake et al. and other groups suggest that an alternative therapeutic approach could be to prevent cell death in sutures. Developing such an approach will require researchers to pool together all the knowledge that has been gained from a wide range of genetics studies in both mice and humans. This is therefore a crucial moment in the search for the best treatment strategy for a large proportion of people with non-syndromic craniosynostosis.
References
-
Augmentation of Smad-dependent BMP signaling in neural crest cells causes craniosynostosis in miceJournal of Bone and Mineral Research 28:1422–1433.https://doi.org/10.1002/jbmr.1857
-
A genetic-pathophysiological framework for craniosynostosisThe American Journal of Human Genetics 97:359–377.https://doi.org/10.1016/j.ajhg.2015.07.006
Article and author information
Author details
Publication history
Copyright
© 2016, Komatsu et al.
This article is distributed under the terms of the Creative Commons Attribution License, which permits unrestricted use and redistribution provided that the original author and source are credited.
Metrics
-
- 2,256
- views
-
- 133
- downloads
-
- 8
- citations
Views, downloads and citations are aggregated across all versions of this paper published by eLife.
Download links
Downloads (link to download the article as PDF)
Open citations (links to open the citations from this article in various online reference manager services)
Cite this article (links to download the citations from this article in formats compatible with various reference manager tools)
Further reading
-
- Cell Biology
- Chromosomes and Gene Expression
During oncogene-induced senescence there are striking changes in the organisation of heterochromatin in the nucleus. This is accompanied by activation of a pro-inflammatory gene expression programme – the senescence-associated secretory phenotype (SASP) – driven by transcription factors such as NF-κB. The relationship between heterochromatin re-organisation and the SASP has been unclear. Here, we show that TPR, a protein of the nuclear pore complex basket required for heterochromatin re-organisation during senescence, is also required for the very early activation of NF-κB signalling during the stress-response phase of oncogene-induced senescence. This is prior to activation of the SASP and occurs without affecting NF-κB nuclear import. We show that TPR is required for the activation of innate immune signalling at these early stages of senescence and we link this to the formation of heterochromatin-enriched cytoplasmic chromatin fragments thought to bleb off from the nuclear periphery. We show that HMGA1 is also required for cytoplasmic chromatin fragment formation. Together these data suggest that re-organisation of heterochromatin is involved in altered structural integrity of the nuclear periphery during senescence, and that this can lead to activation of cytoplasmic nucleic acid sensing, NF-κB signalling, and activation of the SASP.
-
- Chromosomes and Gene Expression
- Evolutionary Biology
Gene regulation is essential for life and controlled by regulatory DNA. Mutations can modify the activity of regulatory DNA, and also create new regulatory DNA, a process called regulatory emergence. Non-regulatory and regulatory DNA contain motifs to which transcription factors may bind. In prokaryotes, gene expression requires a stretch of DNA called a promoter, which contains two motifs called –10 and –35 boxes. However, these motifs may occur in both promoters and non-promoter DNA in multiple copies. They have been implicated in some studies to improve promoter activity, and in others to repress it. Here, we ask whether the presence of such motifs in different genetic sequences influences promoter evolution and emergence. To understand whether and how promoter motifs influence promoter emergence and evolution, we start from 50 ‘promoter islands’, DNA sequences enriched with –10 and –35 boxes. We mutagenize these starting ‘parent’ sequences, and measure gene expression driven by 240,000 of the resulting mutants. We find that the probability that mutations create an active promoter varies more than 200-fold, and is not correlated with the number of promoter motifs. For parent sequences without promoter activity, mutations created over 1500 new –10 and –35 boxes at unique positions in the library, but only ~0.3% of these resulted in de-novo promoter activity. Only ~13% of all –10 and –35 boxes contribute to de-novo promoter activity. For parent sequences with promoter activity, mutations created new –10 and –35 boxes in 11 specific positions that partially overlap with preexisting ones to modulate expression. We also find that –10 and –35 boxes do not repress promoter activity. Overall, our work demonstrates how promoter motifs influence promoter emergence and evolution. It has implications for predicting and understanding regulatory evolution, de novo genes, and phenotypic evolution.