Mutational phospho-mimicry reveals a regulatory role for the XRCC4 and XLF C-terminal tails in modulating DNA bridging during classical non-homologous end joining
Figures
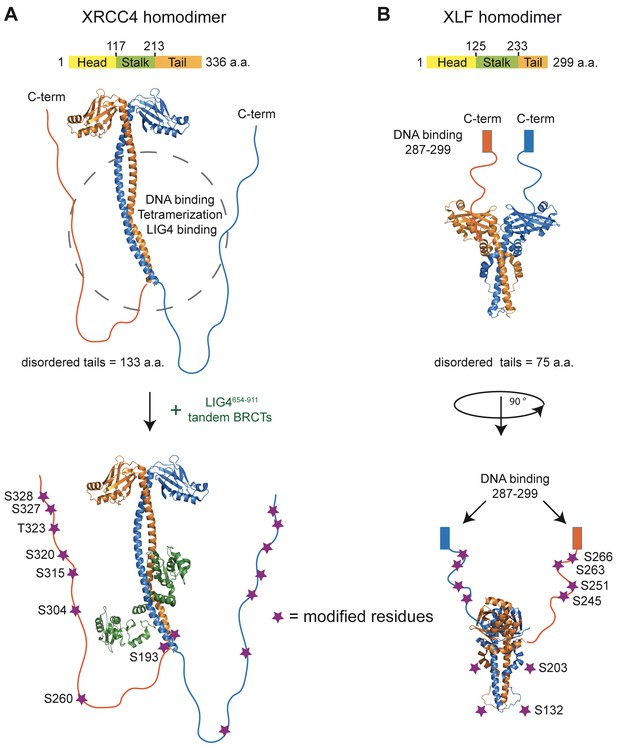
Structural organization of XRCC4 and XLF homodimers.
(A) Structure of XRCC4 homodimer based on PDB 3II6, including residues 1–203 of XRCC4 and a schematic representation of the C-terminal disordered tails. In the bottom panel, also residues 654–911 of LIG4 are shown (green). (B) Structure of XLF homodimer based on PDB 2R9A, including residues 1–224 and a schematic representation of the C-terminal tails. Purple stars indicate the residues modified for phospho-mimicking or -blocking, and all were mutated, respectively, to Asp or Ala in this study. Residues are numbered using as reference Uniprot Q13426 isoform 1 and Q9H9Q4 isoform 1 for XRCC4 and XFF, respectively.
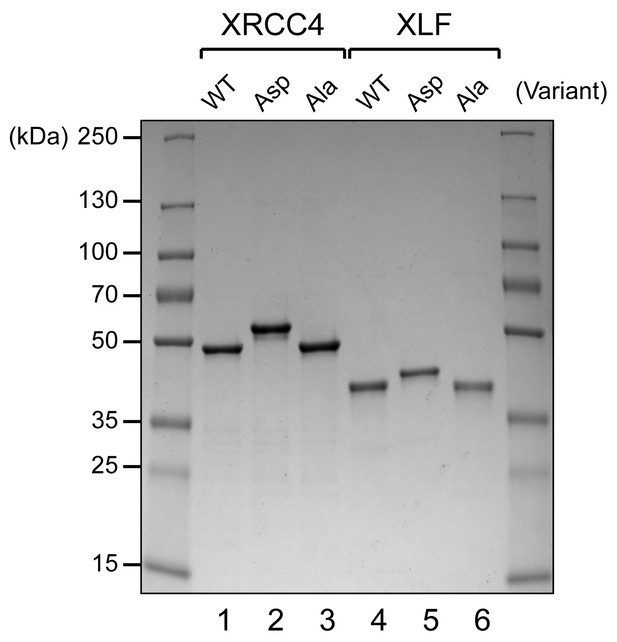
Coomassie stained reducing SDS-PAGE analysis of recombinant XRCC4 and XLF variants produced in bacteria (1 μg/lane).
https://doi.org/10.7554/eLife.22900.004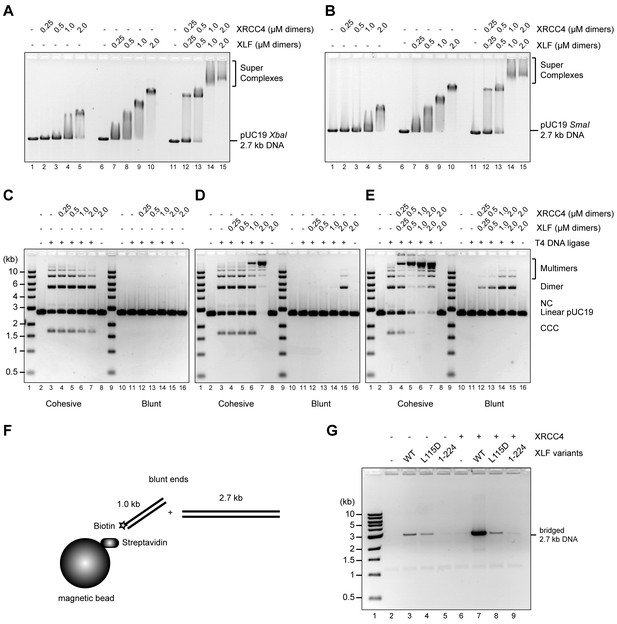
Wild-type XRCC4 and XLF form super-complexes in vitro that bridge DNA favoring molecular collision rates that enhance DNA ligation.
(A) EMSA using a 2.7 kb DNA fragment with protruding ends and the indicated proteins resolved by agarose gel electrophoresis and DNA detected by ethidium bromide staining. (B) EMSA using a blunt-ended 2.7 kb DNA fragment and the indicated proteins resolved by agarose gel electrophoresis and DNA detected by ethidium bromide staining. (C) (D) (E) T4 DNA ligase assay using a 2.7 kb DNA fragment with cohesive (left) or blunt ends (right) and the indicated proteins. Ligation products were deproteinized and resolved by agarose gel electrophoresis followed by detection by ethidium bromide staining. NC = nicked circle, CCC = covalently closed circle. (F) Schematic of the DNA pull-down/bridging assay. A one-end biotinylated 1 kb DNA fragment is first attached to streptavidin-coated magnetic beads and then incubated with a blunt-ended 2.7 kb DNA fragment in the presence of the proteins of interest. (G) Bridging assays for the indicated proteins resolved by agarose gel electrophoresis after deproteinization followed by DNA detection by ethidium bromide staining.
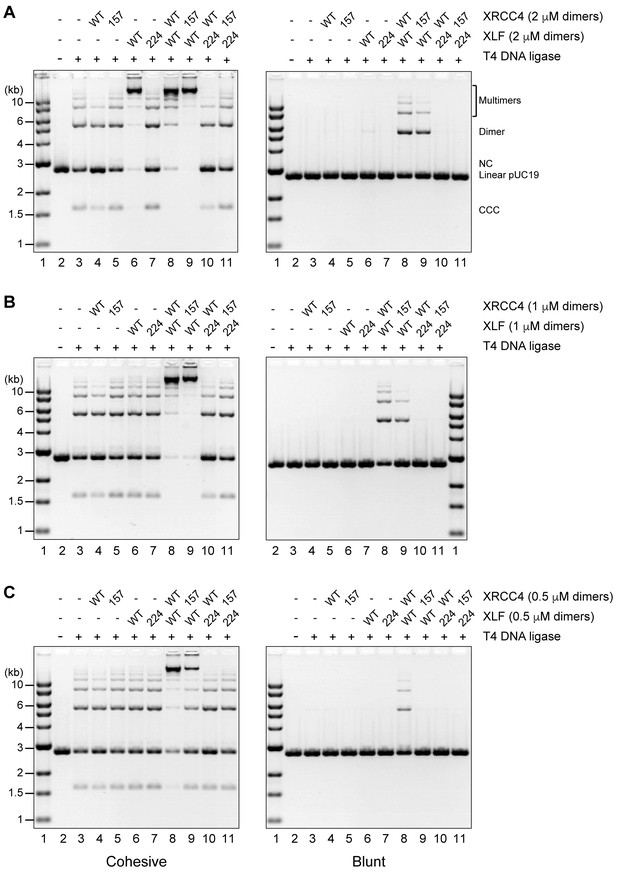
T4 DNA ligase assay using a 2.7 kb DNA fragment with cohesive (left) or blunt ends (right) at three different protein equimolar concentrations.
(A) 2 μM each, (B) 1 μM each, and (C) 0.5 μM each. Ligation products were deproteinized and resolved by agarose gel electrophoresis followed by DNA detection by ethidium bromide staining. NC = nicked circle, CCC = covalently closed circle.
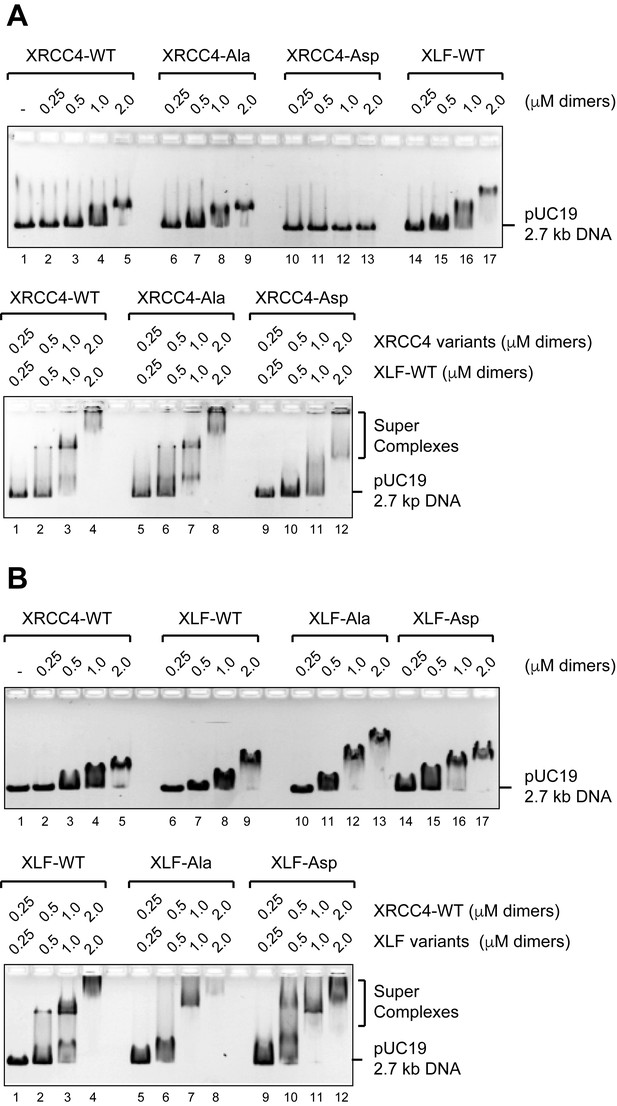
Formation of DNA/XRCC4/XLF super-complexes is not disrupted by phosphorylation site mutation.
(A) EMSAs showing DNA binding of XRCC4 variants in isolation (top panel) and cooperatively with XLF-WT (bottom panel) resolved by agarose gel electrophoresis and detected by ethidium bromide staining. (B) EMSAs showing DNA binding of XLF variants in isolation (top panel) and cooperatively with XRCC4-WT (bottom panel) resolved by agarose gel electrophoresis and detected by ethidium bromide staining.
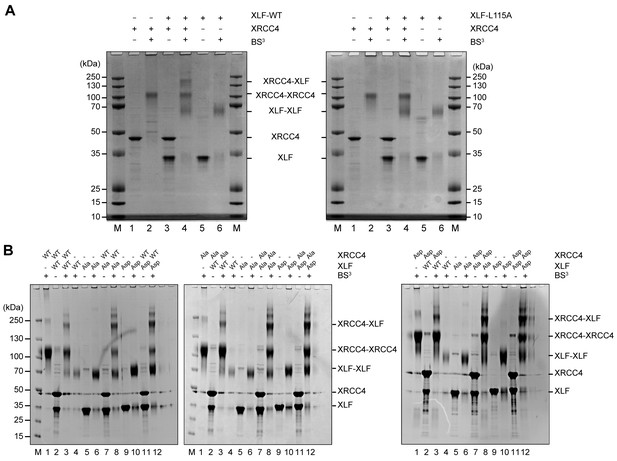
Phosphorylation site mutations do not alter XRCC4-XLF direct interaction.
XRCC4 and XLF were cross-linked in isolation or in equimolar combination with BS3. Cross-linked species were resolved by reducing and denaturing SDS-PAGE and detected by Coomassie staining. (A) XRCC4 and XLF WT-WT combination (left panel) and XRCC4-WT combined with XLF-L115A, that has a point mutation that diminished affinity for XRCC4 (right panel). (B) XRCC4-WT (left panel), XRCC4-Ala (middle panel) and XRCC4-Asp (right panel) respectively cross-linked to all the XLF variants. M = protein molecular weight size ladder.
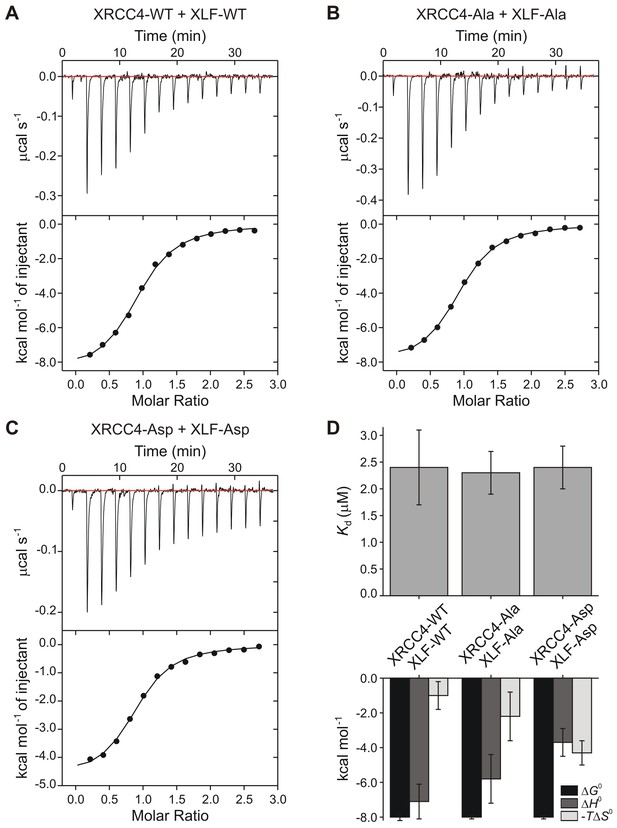
Thermodynamic analysis of wild-type and phospho-mimetic XRCC4-XLF interaction.
Isothermal titration micro-calorimetry data obtained at 37°C injecting (A) XLF-WT (154 μM) into XRCC4-WT (12 μM), (B) XLF-Ala (250 μM) into XRCC4-Ala (19 μM), (C) XLF-Asp (250 μM) into XRCC4-Asp (19 μM). Top panels display the thermogram of the heat exchanged as a function of time after baseline (red lines) subtraction. Bottom panels show the result of the (numerical) integration of the peaks as a function of the molar ratio between the titrant (XLF) and the analyte (XRCC4); black lines are non-linear least squares fits of the data. Data are representative results out of two experimental replicas each composed of a technical duplicate (see Table 1 for details). Source data are included in Table 1—source data 1. (D) Equilibrium constant (Kd) (top) and thermodynamic parameters (free energy ΔG0, black bars; enthalpy ΔH0, dark grey bars; and entropy –TΔS0, light grey bars) (bottom) obtained from sigmoidal fit (black lines in bottom panels of (A, B, C) of ITC curves. Bars show mean values and error bars represent the standard error of the mean (SEM) of the four experimental runs performed per each condition. Thermodynamic parameter values are reported in Table 1.
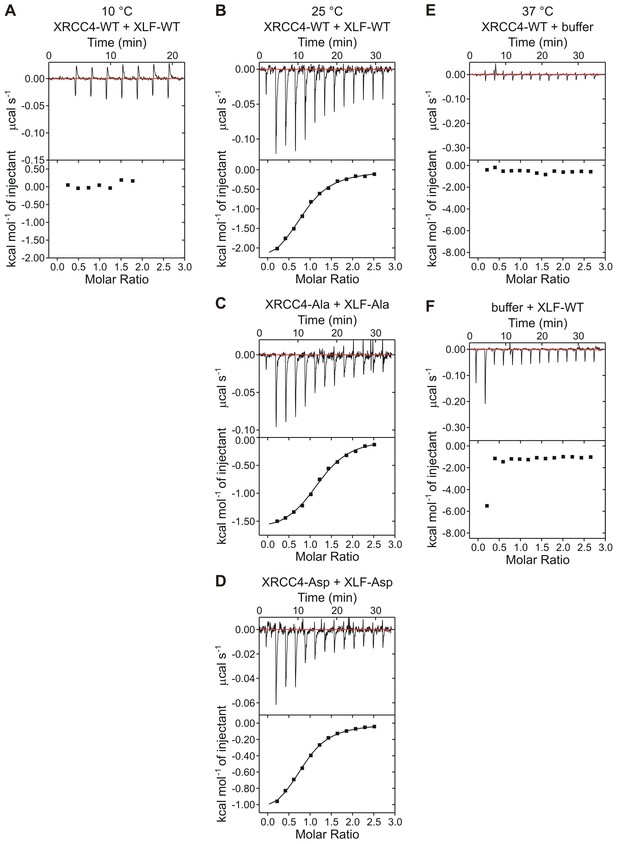
Isothermal titration micro-calorimetry thermograms.
Top panels display the heat exchanged as a function of time, after baseline (red lines) subtraction. Bottom panels show the result of the (numerical) integration of the peaks as a function of the molar ratio between the titrant (XLF) and the analyte (XRCC4). Thermograms have been obtained (A) at 10°C injecting XLF-WT (168 μM) into XRCC4-WT (17 μM) and at 25°C injecting (B) XLF-WT (330 μM) into XRCC4-WT (25 μM), (C) XLF-Ala (330 μM) into XRCC4-Ala (25 μM), and (D) XLF-Asp (330 μM) into XRCC4-Asp (25 μM). Control isothermal titration micro-calorimetry thermograms obtained at 37°C injecting (E) buffer into XRCC4-WT (12 μM) and (F) XLF-WT (154 μM) into buffer (the first large peak might represent XLF homodimer dissociation heat due to XLF dilution). Source data are included in Table 1—source data 1 (panels A–D) and reported in Figure 5—figure supplement 1—source data 1 (panels E and F).
-
Figure 5—figure supplement 1—source data 1
Thermograms of control experiments.
- https://doi.org/10.7554/eLife.22900.011
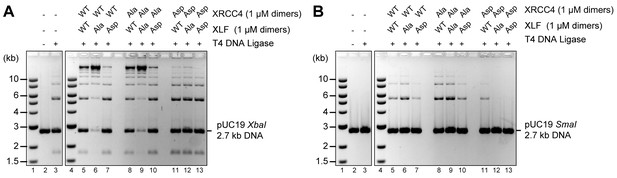
Blocking XRCC4 and XLF phosphorylation sites enhances DNA bridging; phospho-mimicking mutations abate DNA bridging.
All combinations of XRCC4 variants with XLF variants tested in ability to stimulate T4 DNA ligase cohesive end ligation (A) or blunt end ligation (B). Ligation products were deproteinized and resolved by agarose gel electrophoresis followed by detection by ethidium bromide staining.
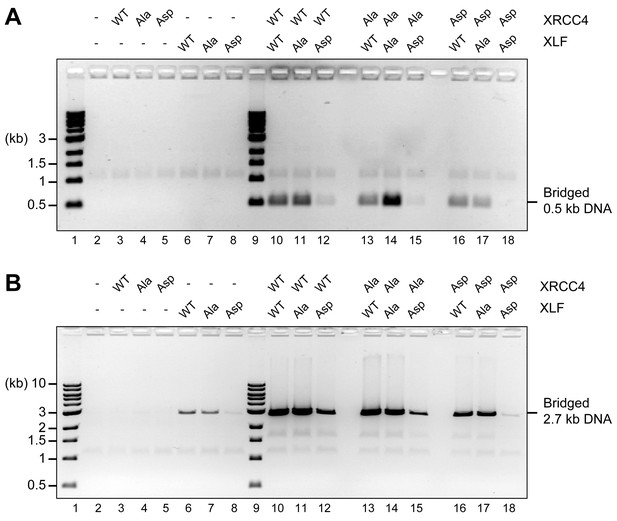
Blocking XRCC4 and XLF phosphorylation sites enhances DNA bridging; phospho-mimicking mutations abate DNA bridging.
DNA bridging assays using a short blunt ended 0.5 kb DNA (A) or a long blunt ended 2.7 kb DNA (B) and the indicated proteins. Samples were deproteinized and resolved by agarose gel electrophoresis followed by detection by ethidium bromide staining.
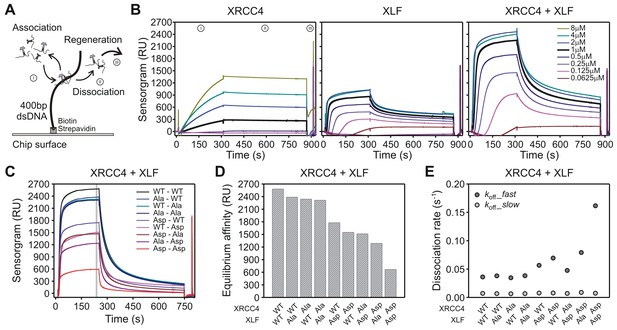
Phospho-mimicking XRCC4 and XLF mutants associate with DNA, but dissociate rapidly.
(A) Scheme of the SPR assay with 400 bp blunt end DNA anchored to the streptavidin coated chip via biotin-streptavidin linkage. (B) From left to right, sensorgrams of XRCC4-WT, XLF-WT, and equi-molar mix of XRCC4-WT and XLF-WT at the indicated concentrations. Source data are provided in Figure 8—source data 1, 2 and 3, respectively. (C) Sensorgrams of all XRCC4 and XLF variant combinations each at 1 μM concentration. Source data are provided in Figure 8—source data 4. (D) Equilibrium affinity of the different combinations of XRCC4 and XLF variants. Source data are provided in Figure 8—source data 5. (E) Dissociation rates for the different combinations of XRCC4 and XLF variants. koff_fast (dark grey symbols) and koff_slow (light grey symbols) correspond, respectively, to the initial (fast) and late (slow) phase in the biphasic dissociation curves. Error bars fall within symbol dimension. Source data are provided in Figure 8—source data 5.
-
Figure 8—source data 1
Sensorgrams of XRCC4 WT tritation.
- https://doi.org/10.7554/eLife.22900.017
-
Figure 8—source data 2
Sensorgrams of XLF WT tritation.
- https://doi.org/10.7554/eLife.22900.018
-
Figure 8—source data 3
Sensorgrams of XRCC4-XLF WT-WT tritation.
- https://doi.org/10.7554/eLife.22900.019
-
Figure 8—source data 4
Sensorgrams of all XRCC4-XLF variant combinations.
- https://doi.org/10.7554/eLife.22900.020
-
Figure 8—source data 5
Equilibrium affinity and dissociation rates of all XRCC4-XLF variant combinations.
- https://doi.org/10.7554/eLife.22900.021
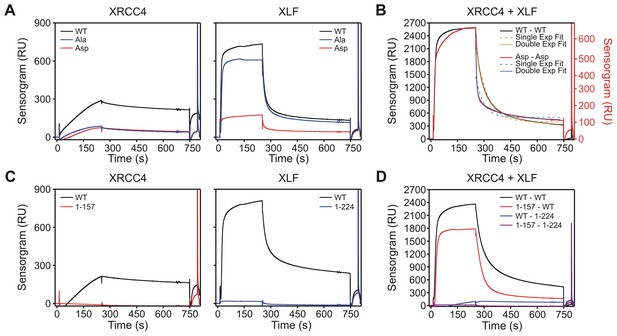
Sensorgrams of XRCC4 and XLF variants and truncations.
(A) Sensorgrams of XRCC4 variants (left) or XLF variants (right) at 1 μM concentration in isolation. Source data are provided in Figure 8—figure supplement 1—source data 1. (B) Sensorgrams comparison between XRCC4 and XLF WT-WT (black, left scale) and Asp-Asp (red, right scale) at equimolar concentration (1 μM each) showing the faster dissociation of the Asp-Asp combination and the deviation in both cases from a single exponential decay (dashed lines) and the biphasic behavior (solid lines). Source data are provided in Figure 8—figure supplement 1—source data 2. (C) Sensorgrams of XRCC4-WT and truncation XRCC4(1–157) lacking the C-terminal tail at 1 μM concentration in isolation (left); and sensorgrams of XLF-WT and truncation XLF(1-224) lacking the C-terminal tails at 1 μM concentrations in isolation (right). Source data are provided in Figure 8—figure supplement 1—source data 3. (D) Sensorgrams of equimolar combinations (1 μM each) of the WT and truncated XRCC4 and XLF proteins as indicated. Source data are provided in Figure 8—figure supplement 1—source data 3.
-
Figure 8—figure supplement 1—source data 1
Sensorgrams of XRCC4 and XLF variants in isolation.
- https://doi.org/10.7554/eLife.22900.023
-
Figure 8—figure supplement 1—source data 2
Sensorgrams and mono- and double-exponential fits of XRCC4-XLF WT-WT and Asp-Asp combinations.
- https://doi.org/10.7554/eLife.22900.024
-
Figure 8—figure supplement 1—source data 3
Sensorgrams of XRCC4-XLF full-length and truncations in isolation and in combination.
- https://doi.org/10.7554/eLife.22900.025
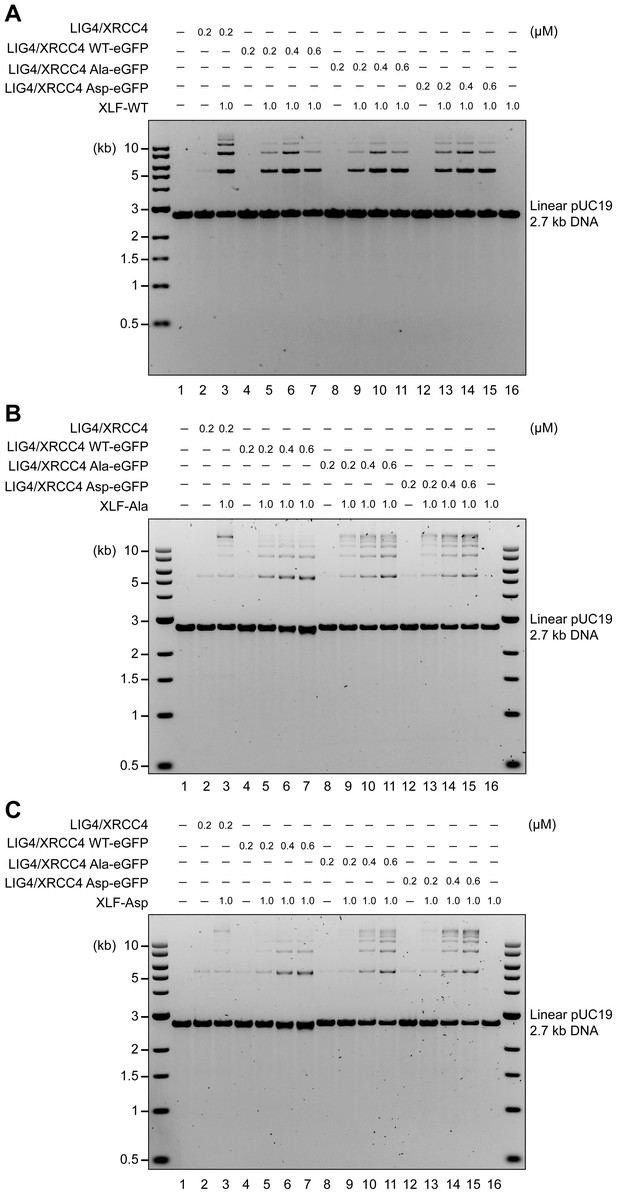
XRCC4 and XLF phospho-mimetics do not bridge DNA ends but are completely sufficient in stimulating LIG4-XRCC4 activity.
Stimulation of LIG4/XRCC4-WT, LIG4/XRCC4-WT-eGFP, LIG4/XRCC4-Ala-eGFP or LIG4/XRCC4-Asp-eGFP cohesive end ligation by XLF-WT (A), XLF-Ala (B) or XLF-Asp (C). Ligation products were deproteinized and resolved by agarose gel electrophoresis followed by detection by ethidium bromide staining.
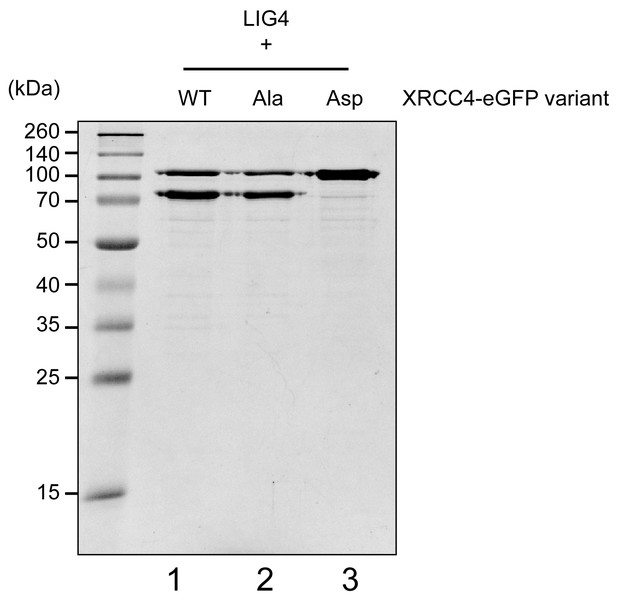
Coomassie stained reducing SDS-PAGE analysis of recombinant LIG4/XRCC4 variants purified after overexpression in bacteria (1 μg/lane).
https://doi.org/10.7554/eLife.22900.027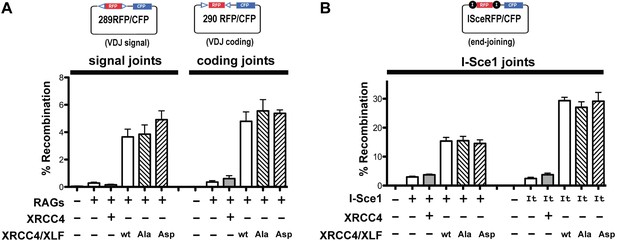
XRCC4 and XLF phosphorylation site mutation does not affect end joining of episomal substrates.
Fluorescent substrates (depicted in top panels) were utilized to detect V(D)J coding and signal joints in XRCC4/XLF-deficient 293 T cells transiently expressing full-length RAG1, RAG2, and WT, Ala, or Asp mutants of XRCC4 and XLF (A), or joining of I-Sce1 induced DSBs (B). Bottom panels show percent recombination of episomal fluorescent substrates. Error bars indicate SEM from five independent experiments.
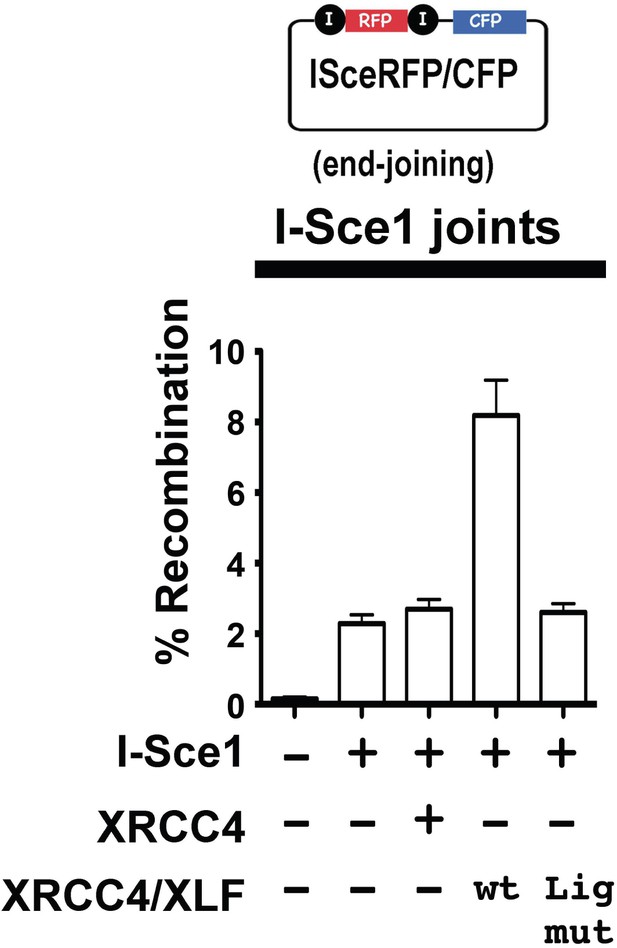
XRCC4 and XLF complexes do not promote a-NHEJ.
The fluorescent substrate (depicted in the top panel) was utilized to detect joining of I-Sce1 induced DSBs. Bottom panel shows percent recombination of episomal fluorescent I-Sce1 end joining substrate in XRCC4/XLF-deficient 293 T cells transiently expressing I-Sce1, XRCC4 alone, WT XRCC4 and XLF, or an XRCC4 mutant that is defective in LIG4 interaction and WT XLF as indicated. Error bars indicate SEM from three independent experiments.
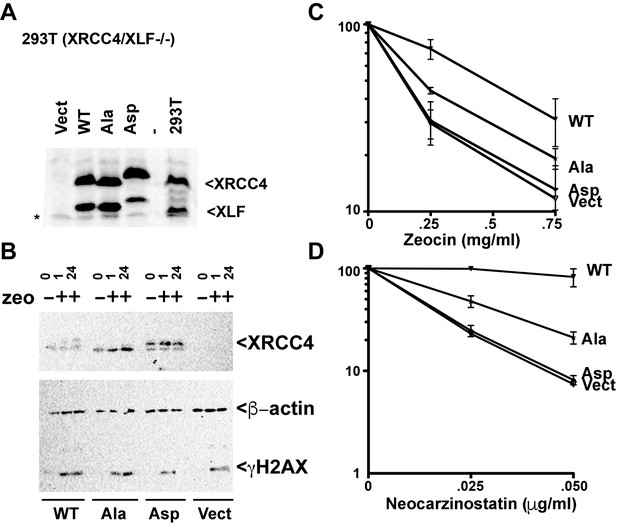
XRCC4 and XLF phosphorylation site mutants do not fully reverse sensitivity to radio-mimetic drugs.
(A) Immunoblots of stable expression of WT, Ala, or Asp mutants of XRCC4 and XLF in 293 T cells that lack both XRCC4 and XLF. * non-specific species. (B) 293 T cells expressing WT, Ala, Asp, or no XRCC4 or XLF were exposed to Zeocin (500 μg/ml for 1 hr) or not and analyzed after 1 hr or 24 hr by immunoblotting for XRCC4, β-actin, or γ-H2AX as indicated. Zeocin (C) and Neocarzinostatin (D) sensitivity of 293T XRCC4/XLF double deficient cell strains complemented by stable expression of equivalent levels of WT, Ala, or Asp mutants of XRCC4 and XLF. Error bars indicate SEM from at least three independent experiments.
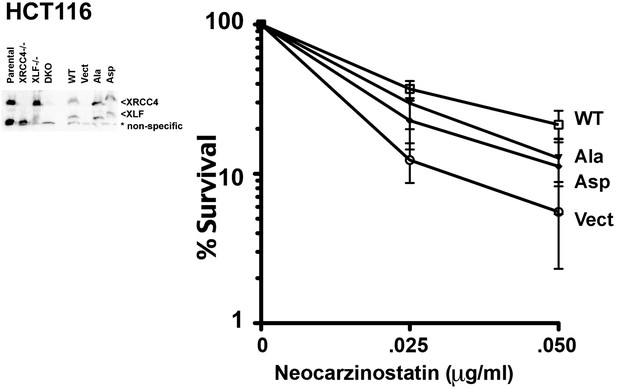
XRCC4 and XLF phosphorylation site mutants do not fully reverse sensitivity to radio-mimetic drugs.
(Left) Immunoblot showing comparable to endogenous, stable expression of WT, Ala, or Asp mutants of XRCC4 and XLF in previously described XRCC4 deficient HCT116 cells that were deleted for XLF. Parental cell strains, as well as XLF deficient cells that were generated by AAV targeting are also depicted. (Right) Neocarzinostatin sensitivity of the indicated cell strains stably expressing WT, Ala, or Asp mutants of XRCC4 and XLF. Error bars indicate SEM from at least three independent experiments.
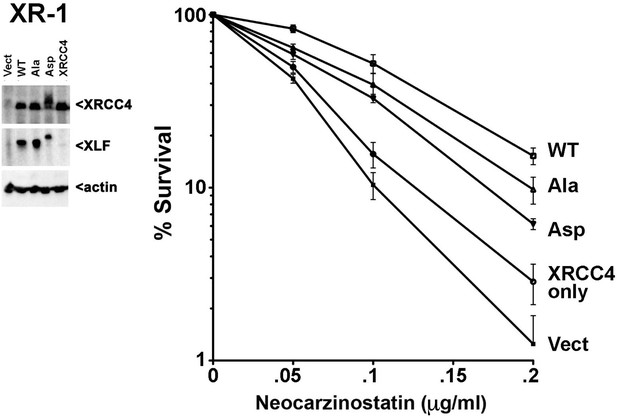
XRCC4 and XLF phosphorylation site mutants do not fully reverse sensitivity to radio-mimetic drugs.
(Left) Immunoblot showing comparable to endogenous, stable expression of WT, Ala, or Asp mutants of XRCC4 and XLF in XRCC4-deficient hamster XR-1 cells deleted for XLF. (Right) Neocarzinostatin sensitivity of the indicated cell strains stably expressing WT, Ala, or Asp mutants of XRCC4 and XLF. Error bars indicate SEM from at least three independent experiments.
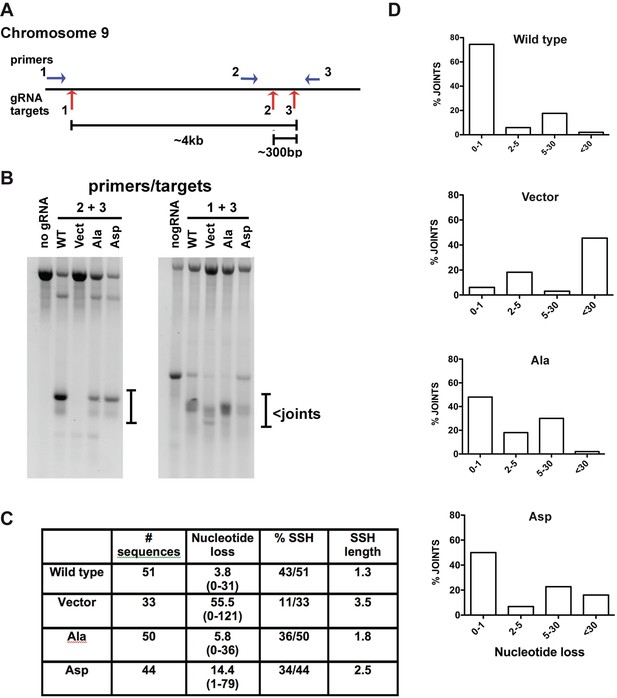
Phospho-mimicking XRCC4 and XLF alters repair of chromosomal DNA DSBs.
(A) Diagram of region on chromosome nine targeted by three different gRNAs and position of primers utilized to detect chromosomal deletions induced by Cas9 and indicated gRNAs. (B) Ethidium bromide staining of PCR amplifications of DNA isolated from cells expressing WT, Ala, Asp, or no XRCC4 and XLF and transfected with the different combinations of gRNAs as indicated. A no gRNA control DNA of parental 293 T cells is also included to help define products induced by Cas9 induced deletions. (C) Summary of sequenced joints utilizing gRNAs 1 and 3 that result in a 4 kb chromosomal deletion. (D) Graphs represent the percentage of joints deleting increasing numbers of nucleotides from predicted double strand break site.
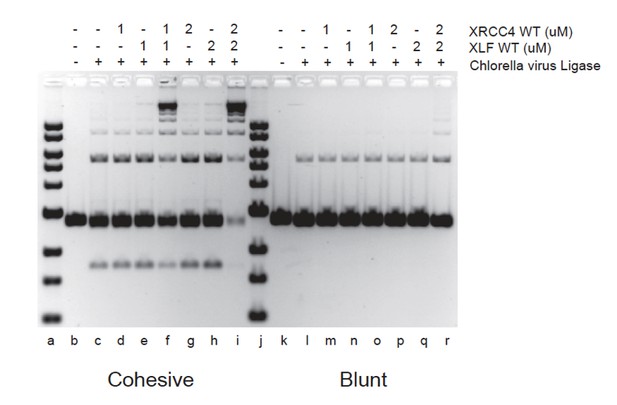
Tables
Impact of phospho-mimicking or phospho-blocking mutations on the thermodynamics parameters of XRCC4-XLF protein-protein interaction by micro-calorimetry.
T (°C) | 2-Me (mM) | [XRCC4] (μM) | [XLF] (μM) | Ratio | N | Ka (M−1) | ΔH0 (kcal/mol) | Kd (μM) | −ΔG0 (kcal/mol) | −TΔS0 (kcal/mol) | Thermogram shown in | Source data | <Kd> (μM) | <ΔH0> (kcal/mol) | <-ΔG0> (kcal/mol) | <-TΔS0> (kcal/mol) | |
---|---|---|---|---|---|---|---|---|---|---|---|---|---|---|---|---|---|
ITC cell | ITC syringe | ||||||||||||||||
XRCC4-WT XLF-WT | 10 | 10 | 17 | 168 | 10 | n.d. | n.d. | n.d. | n.d. | n.d. | n.d. | Figure 5—figure supplement 1A | A | ||||
25 | 10 | 17 | 168 | 10 | 0.97 | 536000 | −1.7 | 1.8 | −7.8 | −6.1 | -- | B | 3.2 ± 0.8 | −2.4 ± 0.4 | −7.5 ± 0.2 | −5.2 ± 0.5 | |
25 | 10 | 33 | 330 | 10 | 0.91 | 306000 | −2.9 | 3.2 | −7.5 | −4.6 | Figure 5—figure supplement 1B | C | |||||
25 | 10 | 25 | 330 | 13 | 0.84 | 222000 | −2.6 | 4.5 | −7.3 | −4.7 | -- | D | |||||
37 | 0 | 23 | 300 | 13 | 0.85 | 273000 | −6.0 | 3.7 | −7.7 | −1.7 | -- | E | 2.4 ± 0.7 | −7.1 ± 1 | −8.0 ± 0.2 | −1.0 ± 0.8 | |
37 | 0 | 23 | 300 | 13 | 0.89 | 278000 | −4.9 | 3.6 | −7.7 | −2.9 | -- | F | |||||
37 | 0 | 12 | 154 | 13 | 0.92 | 848000 | −8.6 | 1.2 | −8.4 | 0.2 | Figure 5A | G | |||||
37 | 0 | 12 | 154 | 13 | 0.84 | 760000 | −8.8 | 1.3 | −8.3 | 0.4 | -- | H | |||||
XRCC4-Ala XLF-Ala | 25 | 10 | 25 | 330 | 13 | 1.21 | 300000 | −1.7 | 3.3 | −7.5 | −5.8 | Figure 5—figure supplement 1C | I | ||||
37 | 0 | 25 | 325 | 13 | 1.07 | 637000 | −3.1 | 1.6 | −8.2 | −5.1 | -- | J | 2.3 ± 0.4 | −5.8 ± 1.4 | −8.0 ± 0.1 | −2.2 ± 1.4 | |
37 | 0 | 25 | 325 | 13 | 1 | 343000 | −3.7 | 2.9 | −7.8 | −4.1 | -- | K | |||||
37 | 0 | 19 | 250 | 13 | 0.92 | 345000 | −8.4 | 2.9 | −7.8 | 0.6 | -- | L | |||||
37 | 0 | 19 | 250 | 13 | 0.92 | 582000 | −8.1 | 1.7 | −8.2 | −0.1 | Figure 5B | M | |||||
XRCC4-Asp XLF-Asp | 25 | 10 | 35 | 350 | 10 | 1.27 | 672000 | −0.9 | 1.5 | −7.9 | −7.0 | -- | N | ||||
25 | 10 | 25 | 330 | 13 | 0.82 | 263000 | −1.2 | 3.8 | −7.4 | −6.2 | Figure 5—figure supplement 1D | O | |||||
37 | 0 | 32 | 416 | 13 | 1.06 | 289000 | −2.4 | 3.4 | −7.8 | −5.4 | -- | P | 2.4 ± 0.4 | −3.7 ± 0.8 | −8.0 ± 0.1 | −4.3 ± 0.7 | |
37 | 0 | 32 | 416 | 13 | 0.95 | 425000 | −2.3 | 2.4 | −8.0 | −5.7 | -- | Q | |||||
37 | 0 | 19 | 250 | 13 | 0.88 | 566000 | −4.7 | 1.8 | −8.2 | −3.5 | Figure 5C | R | |||||
37 | 0 | 17 | 220 | 13 | 0.85 | 538000 | −5.5 | 1.8 | −8.1 | −2.6 | -- | S |
-
2-Me stands for 2-Mercaptoethanol
-
Ratio indicates the titrant over analyte concentration ([XLF]/[XRCC4])
-
Source data specifies the column corresponding to each thermogram in Table 1—source data 1.
-
Last four columns report mean values ± Standard Error of the Mean (SEM) from four experimental runs at 37°C (grey cells) and 3 runs at 25°C (white cells)
-
n.d. denotes no detectable heat transfer (at 10°C)
-
Table 1—source data 1
Thermograms of XRCC4-XLF WT-WT, Ala-Ala, and Asp-Asp experiments.
- https://doi.org/10.7554/eLife.22900.013