Theta oscillations locked to intended actions rhythmically modulate perception
Figures
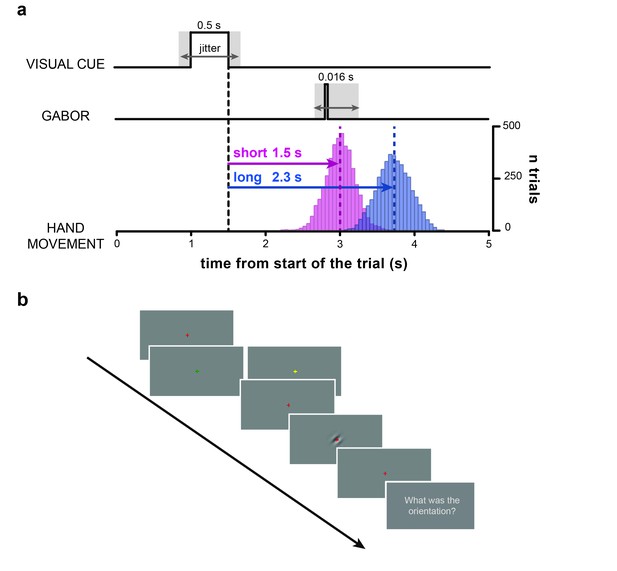
Task.
(a) Timeline of the trial. After a variable delay between 0.8 and 1.2 s from the start of the trial (i.e., display of the red fixation cross) the visual cue is presented (i.e., a change in color of the fixation cross to either yellow or green). The color of the visual cue (counter-balanced across subjects) indicates whether the participants have to wait for a short (1.5 s) or a long (2.3 s) time interval before executing the hand movement with the isometric joystick. After 0.5 s, the visual cue is removed (i.e., the fixation cross turns red again). The offset of the visual cue marks the start of the time interval (black vertical dashed line) that participants have to wait before executing the hand movement. Bar histograms show the distribution of movement onset times (pooled across participants) for the short (pink) and long (blue) movement timing condition. The dashed vertical lines indicate the mean onset times (short: 1.5 ± 0.2 s; long: 2.22 ± 0.24 s; MEAN±SD). At random times between –0.35 and +0.25 s relative to the instructed movement time (short time interval in this example) a near-threshold contrast Gabor tilted 45 deg clockwise or counterclockwise is briefly flashed for 0.016 s (two frames). Therefore, cue-Gabor delays are on average 1.45 and 2.25 s for the short and long condition, respectively. (b) Example series of snapshots of the visual display during the trial. The red fixation cross is displayed throughout the trial over a uniform gray background, except when it changes color to either yellow or green (visual cue) to indicate the movement timing condition (short/long). The fourth snapshot shows a clockwise-tilted Gabor as an example (for illustrative purposes visual contrast is higher than what used in the experiment). Participants were instructed to wait for the appearance of the question ‘What was the orientation?’ before verbally reporting the orientation of the visual Gabor (last snapshot).
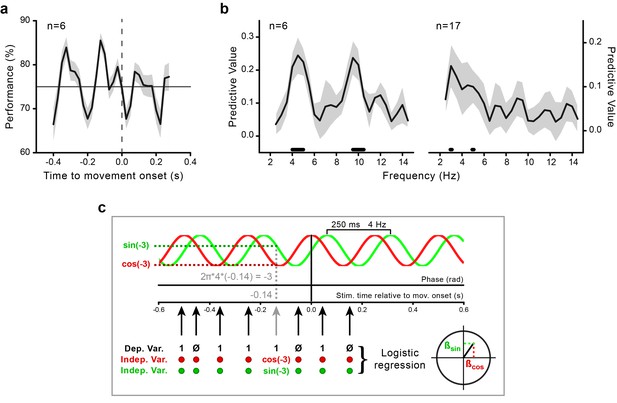
Movement-locked oscillations in visual perception.
(a) Time course of the performance (percentage of correct responses) in the orientation task as a function of stimulus presentation time relative to movement onset (zero time by definition) calculated for the data pooled across subjects (n = 6, behavioral experiment). The gray-shaded area indicates the standard error. The horizontal line indicates threshold performance level (75% correct); the dashed vertical line indicates movement onset time. (b) Predictive value (estimated with Jackknife) for the perceptual outcome of sinusoidal functions with frequencies between 2.5 and 14.5 Hz for the purely behavioral (n = 6; left) and the combined behavioral-EEG dataset (n = 17; right). The gray-shaded area represents the jackknife standard error. The black horizontal bars indicate the frequencies that significantly predict perceptual performance at the group level (p<0.05, not corrected for multiple comparisons across frequencies; see Methods and panel c). (c) Schematic illustration of the analysis for an example sinusoidal function of 4 Hz. For each trial, the phase of the sinusoidal function at stimulus presentation time is computed as 2π*f*t (where f is the frequency of the sinusoid and t is the stimulus presentation time relative to movement onset; in this example 4 Hz and –0.14 s, respectively). The sine and the cosine of the resulting phase value (here, –3 rad) are then used as regressors (independent variables) to predict the perceptual performance (0–1, incorrect-correct) in a logistic regression analysis. Separate regression models are fitted for each subject and frequency in the range from 2.5 to 14.5 Hz. Second-level random-effect analysis is performed by submitting the participant-specific beta coefficients to the Hotelling’s T-square test against zero (see Methods). This test provides significant results only if two conditions are concomitantly fulfilled: (1) the regression coefficients are large (i.e., the phase of the sinusoidal function is consistently associated with perceptual performance), and (2) they have the same sign across subjects (i.e., the phases associated with the highest/lowest performance are aligned across subjects). A very similar pattern of results is also found with a fixed-effects analysis approach based on fast Fourier transform (FFT) on the aggregated data from all participants in combination with permutations at the single trial level (see Figure 2—figure supplement 1 and Materials and methods).
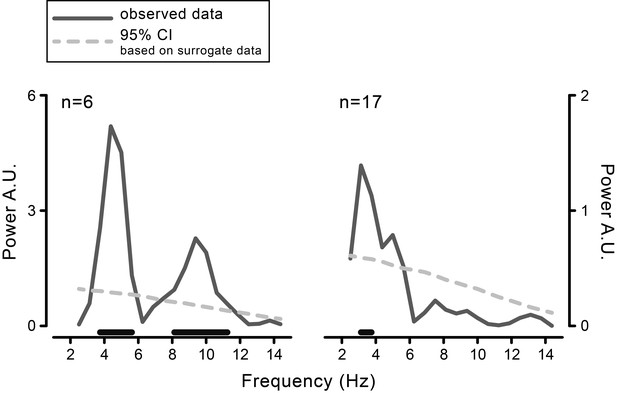
Behavioral results obtained with a fixed-effect analysis based on fast Fourier transform (FFT).
FFT-derived power spectra of the time course of visual performance aligned to movement onset, calculated for the data pooled across participants, separately for the purely behavioral (left) and the combined behavioral-EEG experiment (right). The power value at each frequency in the range from 2.5 to 14.5 Hz (in steps of 0.5 Hz) is compared to a surrogate distribution derived by iteratively (5000 times) shuffling stimulus presentation times. The p-values are yielded by the proportion of times in which the power of the surrogate data exceeds the power of the observed data. Black horizontal bars show the significant frequencies (FDR-corrected).
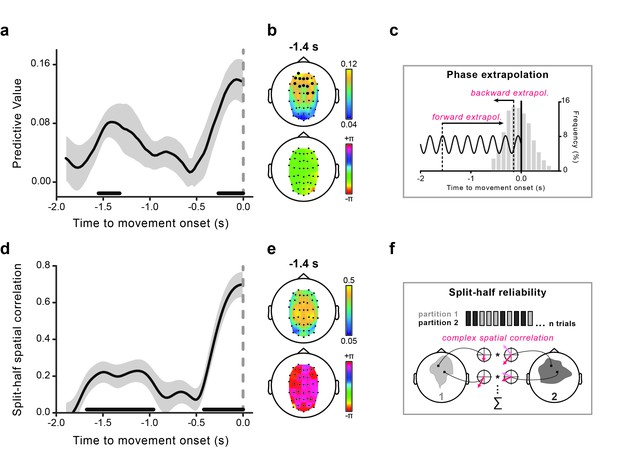
Theta phase predicts visual performance and aligns to movement onset.
(a) Predictive value (estimated with Jackknife) of the 4 Hz theta phase for perception as a function of the time where the phase was estimated relative to movement onset. The gray-shaded area represents jackknife standard error. The black horizontal bars indicate significant time points (after False Discovery Rate – FDR correction across space and time). (b) The topography of the predictive value of theta phase for perception is shown at −1.4 s (earliest peak time in the effect) in the top graph. Significant channels are marked by bigger black circles (FDR-corrected). The topographical distribution of the optimal phase angle (the phase associated with the highest perceptual performance) is shown for the same time point (−1.4 s) in the bottom graph. (c) Schematic illustration of the phase extrapolation procedure. For each trial, the phase at stimulus onset is calculated by extrapolation from the instantaneous EEG phases estimated in the interval from −1.9 to 0 s relative to movement onset. For time points long before the movement (from −1.9 to ~–0.6 s) extrapolation is only performed forward in time, as the visual stimuli always follow the EEG phase estimation point. For points closer to movement onset, extrapolation can either be forward or backward in time, depending on the stimulus presentation time relative to the phase estimation point. The distribution of stimulus presentation times for all analyzed trials (between −0.6 and +0.6 s) is plotted in the gray bar histogram (pooled across subjects). (d) Time course of the split-half spatial correlation (see f) for the theta phase-locking to movement onset time. The gray-shaded area represents the standard error of the mean. The black horizontal bars indicate the time points where the theta oscillation is significantly phase-locked to movement onset (FDR-corrected). (e) Topography of the strength of the inter-individual consistency in the phase of the theta movement-locked oscillation (top) and topographical distribution of the mean phase angle (bottom) are shown for the same time point as in panel b. (f) Schematic illustration of the statistical assessment of the phase-locking to movement onset. For each subject, trials are split into two random partitions of equal size. The mean across trials of the signal’s Hilbert transform time-locked to movement onset is calculated for each partition (and channel) - represented by the red vectors (mean resultant vectors, MRVs). The results are then correlated across space (see Materials and methods for details). This procedure is repeated 500 times, and the obtained spatial correlations are averaged across iterations. This single-subject correlation serves as the input for the group-level statistical test, which consists in submitting the real parts of the individual correlation values to a one-sample t-test (in fact, under the null hypothesis of no phase-locking to movement onset, the expected value of the real part of the complex-valued spatial correlation is equal to 0; see Materials and methods).
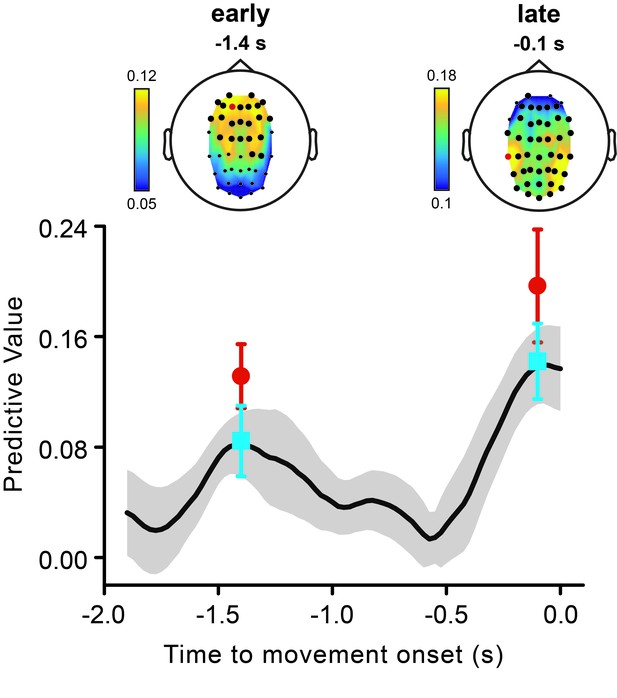
Early and late theta phases have independent predictive power for perception.
The black line represents the time course of the predictive value of theta phase (same data as shown in Figure 3a). To test whether the early and the late peaks in the theta predictive value reflected one or two independent phenomena, we ran logistic regression analyses in which we used both the early (estimated at −1.4 s) and the late theta phase (estimated at −0.1 s) as predictors of the perceptual performance. We performed this analysis in two versions. In a first version, the logistic regression analysis was performed channel-by-channel (as described in the paper for the original analysis), and we used the early and the late theta phases estimated at corresponding EEG channels. Both the early and the late theta phases predict perception, with an almost identical pattern as the original analysis, both with respect to topography (top graphs) and effect size (bottom graph, cyan symbols). This indicates that the two effects are independent. However, because the early and the late effect have different topographies, the channel-by-channel analysis is unlikely to include the relevant phases (the ones for which one has to partial out) for every channel. Therefore, we also ran a second analysis, in which we partialled out for the phases of the other effect measured at its best channel, i.e., the channel with the highest effect size in the original analysis for the early and the late effect (channels are marked in red in the top graphs; early effect: AF3; late effect: CP5). Also in this case, both effects (red symbols) are significant (early effect, AF3: p<0.001; late effect, CP5: p<0.001), confirming their statistical independence.
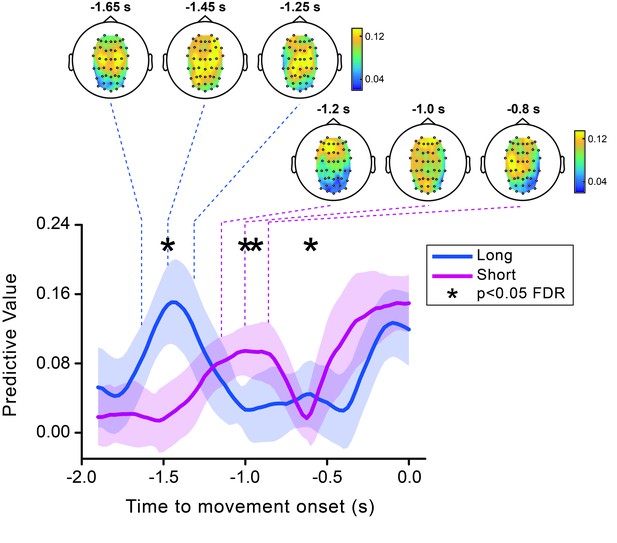
Theta predictive value for perception is modulated by movement timing.
Time course of the predictive value of theta phase calculated separately for the short (pink) and the long (blue) movement timing condition. Shaded areas represent jackknife standard errors. Asterisks show the time points where the short and long timing conditions are significantly different (after FDR correction across time and space). Topographies calculated at three different time points encompassing the early peak in the theta predictive effect for the short (indicated by the dashed pink lines) and the long (indicated by the dashed blue lines) conditions are shown at the top.
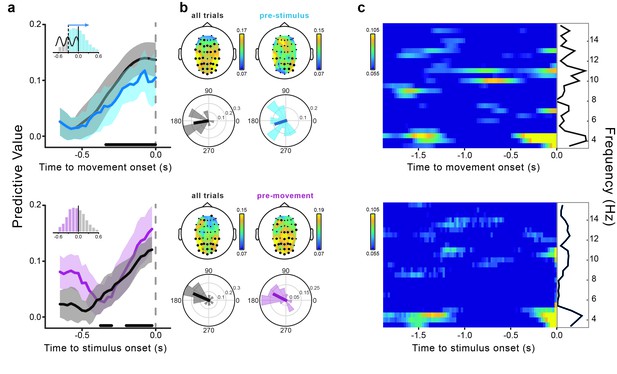
Movement-locked and stimulus-locked effects.
(a) Time course of the predictive value of theta phases from −0.65 to 0 s relative to movement (top) and stimulus onset (bottom). The dark gray lines represent the predictive value calculated for all trials (i.e., stimuli presented from −0.6 to +0.6 s relative to movement onset; movement-locked data are the same as shown in Figure 3a). The light blue line represents the movement-locked effect calculated for trials in which the phase estimation time point preceded the stimulus presentation time (i.e., pre-stimulus or forward extrapolation trials). The pink line represents the stimulus-locked effect calculated only for trials in which the stimuli preceded movement onset. Shaded areas represent the jackknife standard errors. Horizontal bars indicate significant time points for all trials (after FDR correction across space and time points [−0.65–0 s]). The bar histograms in the insets show the distribution of stimulus presentation times relative to movement onset. (b) Topography of the predictive value of theta phase and across-subjects distribution of the optimal phase angle for all trials (dark gray), pre-stimulus trials (light blue; movement-locked effect) and pre-movement trials (pink; stimulus-locked effect). Results are shown at the peak time of the effect (−0.1 and −0.025 s for movement- and stimulus-locked effects, respectively). Significant channels are marked by black bigger circles (FDR-corrected across space and time points [−0.65–0 s] for all trials and across space for the control analyses). (c) Predictive value of oscillatory phase for the perceptual performance as a function of frequency (3.5–15.5 Hz) and time relative to movement (top) and stimulus onset (bottom). The black lines show the predictive value as a function of frequency averaged across the entire time window from −1.9 to 0 s. Time-frequency power plots for both movement- and stimulus-locked data are reported in Figure 4—figure supplement 2.
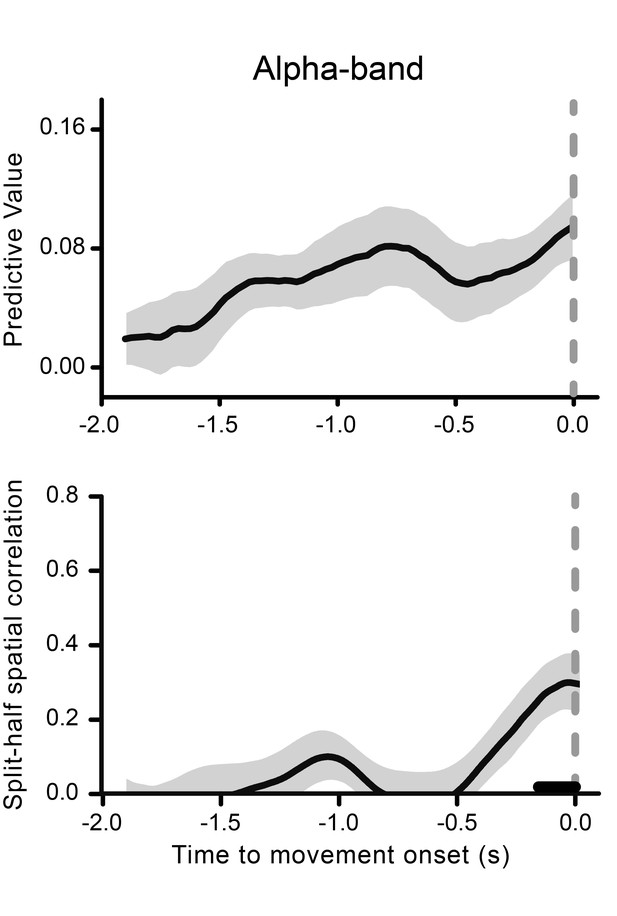
Alpha-band results.
The time course of the predictive value of the alpha (11 Hz) phase for perception is shown as a function of movement onset time in the top graph. The gray-shaded area represents the jackknife standard error. Alpha phase-locking to movement onset as indexed by the split-half spatial correlation is illustrated in the bottom graph. The gray-shaded area represents the standard error of the mean. The black horizontal line shows the time points where alpha-band oscillatory activity is significantly phase-locked to movement onset time (after FDR-correction across time points).
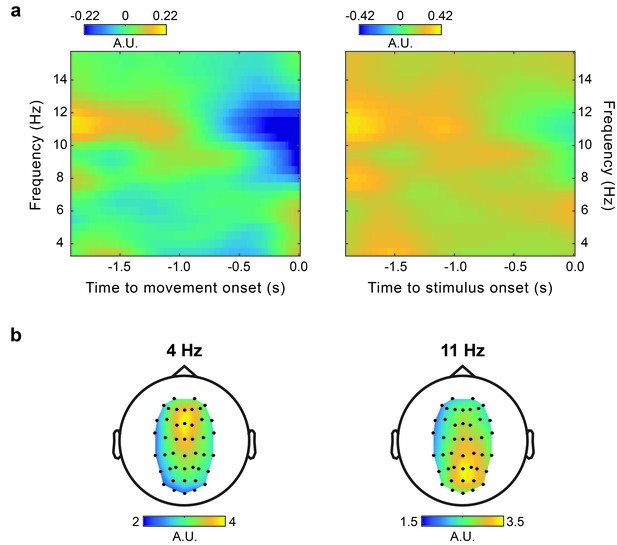
Power.
(a) Time-frequency power plots for movement-locked (left) and stimulus-locked (right) data. Because the pre-cue EEG epoch, which could yield an estimate of baseline power, was often corrupted by artifacts related to the verbal response provided in the preceding trial, and there were no other time windows that could yield adequate baseline measures, power was normalized by the mean over the entire time window considered (i.e., −1.9–0 s). (b) Topography of the non-normalized theta (4 Hz) and alpha (11 Hz) power averaged in the time window from −1.9 to 0 s relative to movement onset.