Mobilization of LINE-1 retrotransposons is restricted by Tex19.1 in mouse embryonic stem cells
Peer review process
This article was accepted for publication as part of eLife's original publishing model.
History
- Version of Record published
- Accepted
- Received
Decision letter
-
Marianne BronnerReviewing Editor; California Institute of Technology, United States
In the interests of transparency, eLife includes the editorial decision letter and accompanying author responses. A lightly edited version of the letter sent to the authors after peer review is shown, indicating the most substantive concerns; minor comments are not usually included.
Thank you for submitting your article "Mobilization of LINE-1 Retrotransposons is Restricted by Tex19.1 in Mouse Embryonic Stem Cells" for consideration by eLife. Your article has been reviewed by three peer reviewers, and the evaluation has been overseen by Marianne Bronner as Reviewing and Senior Editor. Reviewer 2 is Miguel Branco and reviewer 3 is Todd Macfarlan. The reviewers have discussed the reviews with one another and the Reviewing Editor has drafted this decision to help you prepare a revised submission. At the request of reviewer 1, who chose not to reveal their identity, we have included a separate review, though the consolidated review merges the salient points of all three reviewers.
Summary:
In this paper MacLennan et al. introduce new insights into the role of Tex19.1 protein, a previously identified restriction factor for retrotransposon activity in mammals. The authors show post-transcriptional suppression of L1 retrotransposons in multiple cell lines, and reveal that this involves ubiquitination of the L1 ORF1p protein. A role for a cognate UBR2 E3 ligase is identified. It is demonstrated that Tex19.1 mediates levels of ORF1p and retrotransposition, including in mouse ES cells. This is the first report of modifications of ORF1p in the germline and of its control by ubiquitination. As such, this is a significant paper and worthy of publication in eLife. It is a well-dissected mechanism, and a convincing demonstration of the role of TEX19.1 in restricting L1 retrotransposition.
Major revisions:
1) Subsection “TEX19.1 Orthologs Directly Interact With L1-ORF1p”; Discussion, second paragraph. The authors claim direct binding of TEX19, UBR2, and ORF1p. Of the more than 100 proteins identified by various studies as associated with human L1 ORF1p, almost all associations disappear upon RNase treatment. Interactions between bacterially-expressed proteins in pull-down assays may also be mediated by RNA-tethering. While the ability to interact with an RNA-binding deficient mutant of ORF1p is telling, I do not regard it as conclusive, especially when protein interactions are weak as noted. The most obvious control has not been reported. Therefore, IP assays should be performed in the presence of RNases before the statement is made that Tex19.1 is the first example of a mammalian host protein directly binding an L1 protein.
2) In the experiments of the subsection “UBR2 Interacts With L1-ORF1p And Regulates L1 Independently Of Tex19.1 Orthologs”, the effect of Ubr2 is uncoupled from those of Tex19.1. However, the authors propose a model in which "TEX19.1 stimulates a basal activity of UBR2 to bind to and polyubiquitylate L1-ORF1p (Figure 7)". Therefore, it should be directly tested if UBR2 mediated binding and polyubiquitination of ORF1p is in fact increased in the presence of overexpressed TEX19. Similarly, it would be simple to test whether Tex19.1 KO cells show reduced ubiquitylation and turnover of ORF1p. This would be strongly suggestive evidence that the proposed mechanism works endogenously in ESCs.
3) Subsection “TEX19.1 Interacts With Multiple Components Of The Ubiquitin-Proteasome System”, last paragraph; subsection “Isolation Of TEX19.1-YFP Complexes”. To identify proteins interacting with Tex19.1 in mouse ES cells, IP gel lanes were excised in sections and subjected to MS sequencing. Even if not relevant to the conclusions of this paper, I believe the complete results of these MS analyses should be summarized in a supplemental Table for the information of future Tex19.1 investigations (and especially in light of the recent Tarabay et al. paper). At this time, only those proteins chosen for Western blot confirmation are noted in Supplementary file 1.
4) One basic premise of the paper is that TEX19.1 acts on L1s post-translationally and does not affect L1 transcript abundance, but the relevant data are on the weak side. Firstly, it looks like only one litter of a Tex19.1 het x het cross was analyzed, which is not ideal. Then there's no information on what the qPCR primers are targeting – ORF1, ORF2 or the 5' UTR? If the latter, which class? If the former two, then it remains possible that TEX19.1 only affects full-length elements. The best experiment for this would be a Northern blot, but a qPCR to the 5' UTR of active L1 classes would probably be sufficient.
5) Does endogenous UBR2 associate with TEX19 in ESCs (CoIP)?
6) Are there particular domains of Tex19.1/Orf1p that are critical for their interaction? Are these domains conserved amongst human and mouse orthologs? (showing alignments of human and mouse Tex19.1 and orf1p would be helpful).
7) Is Ubr2 required for Tex19 effects on Orf1p? This could be achieved by overexpressing Tex19 in primary cell lines from Ubr2 mutants (like NSCs or PMEFs).
Reviewer 1:
In this paper MacLennan et al. introduce new insights into the role of Tex19.1 protein, a previously identified restriction factor for retrotransposon activity in mammals. The authors show post-transcriptional suppression of L1 retrotransposons in multiple cell lines, and reveal that this involves ubiquitination of the L1 ORF1p protein. A role for a cognate UBR2 E3 ligase is identified. It is demonstrated that Tex19.1 mediates levels of ORF1p and retrotransposition, including in mouse ES cells. This is the first report of modifications of ORF1p in the germline and of its control by ubiquitination. As such, this is a significant paper and worthy of publication in eLife.
A few modest additions would improve the clarity and impact of the paper:
Subsection “TEX19.1 Orthologs Directly Interact With L1-ORF1p”; Discussion, second paragraph. The authors claim direct binding of TEX19, UBR2, and ORF1p. Of the more than 100 proteins identified by various studies as associated with human L1 ORF1p, almost all associations disappear upon RNase treatment. Interactions between bacterially-expressed proteins in pull-down assays may also be mediated by RNA-tethering. While the ability to interact with an RNA-binding deficient mutant of ORF1p is telling, I do not regard it as conclusive, especially when protein interactions are weak as noted. The most obvious control has not been reported. Therefore, IP assays should be performed in the presence of RNases before the statement is made that Tex19.1 is the first example of a mammalian host protein directly binding an L1 protein.
In the experiments of the subsection “UBR2 Interacts With L1-ORF1p And Regulates L1 Independently Of Tex19.1 Orthologs”, the effect of Ubr2 is uncoupled from those of Tex19.1. However, the authors propose a model in which "TEX19.1 stimulates a basal activity of UBR2 to bind to and polyubiquitylate L1-ORF1p (Figure 7)". Therefore, it should be directly tested if UBR2 mediated binding and polyubiquitination of ORF1p is in fact increased in the presence of overexpressed TEX19.
Subsection “TEX19.1 Interacts With Multiple Components Of The Ubiquitin-Proteasome System”, last paragraph; subsection “Isolation Of TEX19.1-YFP Complexes”. To identify proteins interacting with Tex19.1 in mouse ES cells, IP gel lanes were excised in sections and subjected to MS sequencing. Even if not relevant to the conclusions of this paper, I believe the complete results of these MS analyses should be summarized in a supplemental Table for the information of future Tex19.1 investigations (and especially in light of the recent Tarabay et al. paper). At this time, only those proteins chosen for Western blot confirmation are noted in Supplementary file 1.
Subsection “Tex19.1 Orthologs Stimulate Polyubiquitination and Degradation of L1-ORF1p”. Were any attempts made to map the ubiquitinated residues in ORF1p, perhaps by MS analyses? While I do not regard such data as critical to the submission, any attempts made could be reported. Belancio et al. (NAR 2014 v. 42) previously mentioned 32 predicted ubiquitinated sites in the C-terminus of ORF1p. Reporting this fact would be of interest (and suggest how difficult mapping of ubiquitinated sites might become for ORF1p).
https://doi.org/10.7554/eLife.26152.030Author response
Major revisions:
1) Subsection “TEX19.1 Orthologs Directly Interact With L1-ORF1p”; Discussion, second paragraph. The authors claim direct binding of TEX19, UBR2, and ORF1p. Of the more than 100 proteins identified by various studies as associated with human L1 ORF1p, almost all associations disappear upon RNase treatment. Interactions between bacterially-expressed proteins in pull-down assays may also be mediated by RNA-tethering. While the ability to interact with an RNA-binding deficient mutant of ORF1p is telling, I do not regard it as conclusive, especially when protein interactions are weak as noted. The most obvious control has not been reported. Therefore, IP assays should be performed in the presence of RNases before the statement is made that Tex19.1 is the first example of a mammalian host protein directly binding an L1 protein.
We agree with the reviewers that additional data supporting this important point would improve the study as most reported L1-ORF1p interactions are mediated by RNA. However, these interaction partners usually failed to bind L1-ORF1p when coexpressed in bacteria. To be sure about the direct interaction between TEX19 and L1-ORF1p, we have repeated the pulldown experiments with the bacterially expressed human proteins in the presence of micrococcal nuclease, which digests both RNA and DNA, and we observe no difference in the efficiency of the pulldown (Figure 3B). As DNaseI was present in the bacterial lysis buffer as well, we conclude that the observed interaction is independent of nucleic acids.
We have moved panel G from Figure 2 (interactions between bacterially-expressed proteins) and included this as part of an additional figure (new Figure 3A) along with the micrococcal nuclease data (Figure 3B) and new data to address major point 6 (see below). We have also updated the Results and Methods text to reflect this.
2) In the experiments of the subsection “UBR2 Interacts With L1-ORF1p And Regulates L1 Independently Of Tex19.1 Orthologs”, the effect of Ubr2 is uncoupled from those of Tex19.1. However, the authors propose a model in which "TEX19.1 stimulates a basal activity of UBR2 to bind to and polyubiquitylate L1-ORF1p (Figure 7)". Therefore, it should be directly tested if UBR2 mediated binding and polyubiquitination of ORF1p is in fact increased in the presence of overexpressed TEX19. Similarly, it would be simple to test whether Tex19.1 KO cells show reduced ubiquitylation and turnover of ORF1p. This would be strongly suggestive evidence that the proposed mechanism works endogenously in ESCs.
We appreciate the reviewer’s point that the text is a bit loose here and we cannot distinguish increased binding, increased polyubiquitination, or other potential mechanisms that increase the activity of UBR2. We have tightened this up to read “TEX19.1 stimulates a basal activity of UBR2 that promotes polyubiquitination of L1-ORF1p (Figure 7)”. We have also tried to make this clear in the legend for the model shown in Figure 7 by adding the text – “We cannot exclude the possibility that additional factors and/or mechanisms contribute to the effects of UBR2 and TEX19.1 on the stability of mL1-ORF1p.”
We have not tested whether UBR2 binding to L1-ORF1p increases in response to overexpressed TEX19, but this experiment probably requires purification of pure populations of UBR2:L1-ORF1p and UBR2:TEX19:L1-ORF1p complexes to accurately determine binding affinities in vitro. As expression of TEX19 stimulates polyubiquitination and turnover of L1-ORF1p in cells this will confound any analysis of UBR2-L1-ORF1p interactions by quantitative co-IP. We did however show increased polyubiquitination of L1-ORF1p in response to overexpressed TEX19 in Figure 3C of the original submission (now Figure 4C).
Similarly, quantitatively precipitating transient polyubiquitylated species of the heterogenous copies of L1-ORF1p endogenously expressed in ES cells is a difficult experiment. Our preliminary data from control ES cells (original Figure 6A, now Figure 7A) indicated some variability in the extent of the heterogenous polyubiquitin smear which may reflect endogenous deubiquitylase activity in the ES cell lysates. We note that the cell-based ubiquitylation assay used in Figure 3 in the original submission (now Figure 4) is performed under denaturing conditions to inactivate deubiquitylase enzymes.
With respect to turnover of ORF1p in Tex19.1-/- mouse ES cells, we showed in the original manuscript that Tex19.1 KO ES cells have increased abundance of L1-ORF1p protein, with no detectable change in L1 RNA abundance, or activity of reporters for either L1 promoters or L1 translation sequences (original submission Figure 6 and Figure 6—figure supplement 3, now Figure 7 and Figure 7—figure supplement 3). Taken together these data suggest that a change in L1-ORF1p turnover is contributing to the increased L1-ORF1p abundance in Tex19.1 KO ES cells. However, we cannot exclude the possibility that the change in L1-ORF1p abundance reflects the behaviour of variant L1 sequences that were not captured in the qRT-PCR or reporter assays. We have therefore included an additional paragraph in the Discussion discussing stating this possibility.
3) Subsection “TEX19.1 Interacts With Multiple Components Of The Ubiquitin-Proteasome System”, last paragraph; subsection “Isolation Of TEX19.1-YFP Complexes”. To identify proteins interacting with Tex19.1 in mouse ES cells, IP gel lanes were excised in sections and subjected to MS sequencing. Even if not relevant to the conclusions of this paper, I believe the complete results of these MS analyses should be summarized in a supplemental Table for the information of future Tex19.1 investigations (and especially in light of the recent Tarabay et al. paper). At this time, only those proteins chosen for Western blot confirmation are noted in Supplementary file 1.
We have included a file showing the TEX19.1-YFP specific MS hits for the reviewers.
4) One basic premise of the paper is that TEX19.1 acts on L1s post-translationally and does not affect L1 transcript abundance, but the relevant data are on the weak side. Firstly, it looks like only one litter of a Tex19.1 het x het cross was analyzed, which is not ideal. Then there's no information on what the qPCR primers are targeting – ORF1, ORF2 or the 5' UTR? If the latter, which class? If the former two, then it remains possible that TEX19.1 only affects full-length elements. The best experiment for this would be a Northern blot, but a qPCR to the 5' UTR of active L1 classes would probably be sufficient.
Data on L1 transcript abundance in Tex19.1-/- testes have been reported in multiple previous publications using different assays and primer sets: L1 transcript abundance does not change in Tex19.1-/- testes (Öllinger et al., 2008, Reichmann et al., 2012, Tarabay et al., 2013). There has clearly been more than a single litter of animals analysed in terms of L1 RNA abundance across all these studies, which encompasses multiple genetic backgrounds. We have also assessed abundance of H3K4me3, a chromatin modification associated with transcription, at L1 sequences in Tex19.1-/- testes and this does not change either (Crichton et al., 2017, bioRxiv, 099119).
For L1-ORF1p protein abundance, our apologies, we could have made this clearer. L1-ORF1p abundance has not only been examined in one litter. These data are representative of seven Tex19.1-/- animals across four litters. We have included this information in the legend to Figure 1. The confirmatory immunofluorescence in Figure 2C represents an additional three Tex19.1-/- animals from two litters that were not analysed by Western blot. The use of littermate controls for these experiments is particularly important when analysing RNAs or proteins that exhibit developmentally dynamic expression patterns: there is less variation in developmental stage within litters than between litters, which means fewer animals are required to detect a statistically significant effect (n=3 in Figure 2C).
We agree with the reviewers that it is important to determine whether full-length L1 RNAs are affected by Tex19.1 The primers used for L1 qRT-PCR in the original submission were directed against ORF2 as this set of primers is normally used to detect most L1 RNAs present in cells. This was stated in the primer list in Supplementary file 2 of the original submission. But we agree this could have been made clear in the figure legends also. In response to the reviewer’s comments, we have included this information in the legends to Figures 1 and 7. In addition, we have also included new qRT-PCR data on testes for the RNA abundance A, Tf and Gf L1 subtypes using primers designed against the 5’UTR of active subtypes of L1. These data indicate that these active L1 subtypes are expressed at similar levels in Tex19.1-/- and control testes.
Finally, please note that it is possible that the heterogeneous nature of L1 DNA, RNA and protein populations means that changes in L1-ORF1p abundance could be caused by changes in the abundance of variant L1 sequences that were not detected in the qRT-PCR assays (or would not be detected by Northern blot). Therefore marked/tagged copies of L1 are needed to demonstrate that TEX19 can affect L1-ORF1p abundance without altering the abundance of its encoding L1 RNA molecule. These data are in Figure 3D (now Figure 4D). We have tried to stress the importance of these data more in the Discussion.
5) Does endogenous UBR2 associate with TEX19 in ESCs (CoIP)?
It is not clear whether the reviewer is referring to mouse or human proteins. If mouse, the UBR2 co-immunoprecipitating with TEX19.1-YFP in ESCs in Figure 2A and Figure 2B is endogenous UBR2. The interaction between endogenous UBR2 and endogenous TEX19.1 has been shown previously in mouse testes (Yang et al., 2010). If human, our reagents are probably not suitable to test this, the commercial anti human TEX19 antibodies appear to strongly cross-react with other proteins (see Author response image 1).
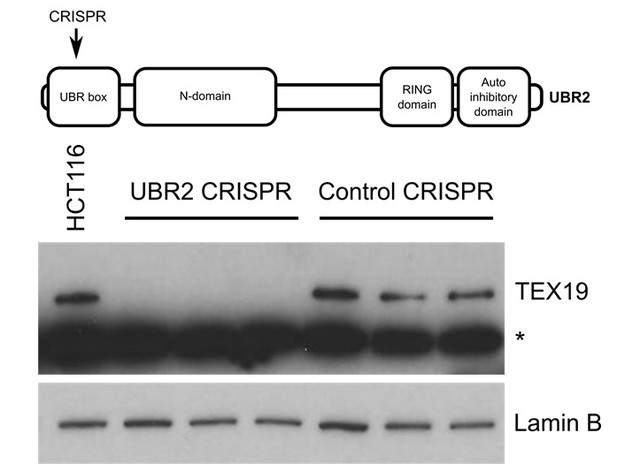
Western blot of endogenous TEX19 in UBR2 mutant and control HCT116 cells.
Parental HCT116 cells and three independent clones isolated from either UBR2 or control CRISPR genome editing experiments are shown. UBR2 CRISPR guides were directed against exon 2 of UBR2, and the three independent UBR2 mutant clones all have deletions in the UBR domain (top panel). Endogenous TEX19 protein is readily detected in parental and control CRISPR lines, but not in UBR2 mutant lines (bottom panel. A cross-reacting band detected with the TEX19 antibodies is marked with an asterisk, and antibodies to lamin B were used as a loading control.
6) Are there particular domains of Tex19.1/Orf1p that are critical for their interaction? Are these domains conserved amongst human and mouse orthologs? (showing alignments of human and mouse Tex19.1 and orf1p would be helpful).
We acknowledge the reviewer’s suggestion and agree that this is useful and informative. Alignments for L1-ORF1p and Tex19 have been published by Boissinot et al. (2016) and Bianchetti et al. (2015) and these are summarized in a new figure (Figure 3, panels C and D). Furthermore, and as suggested by the reviewers, we have performed additional pulldown experiments with sub-fragments of the two proteins to further confine their direct interaction (new figure, Figure 3E and F). These experiments reveal that the highly conserved N-terminal MCP region of Tex19 is necessary and sufficient to interact with L1-ORF1p and suggest the conserved, C-terminal half of the L1ORF1p coiled coil domain as a likely interaction site in all mammals.
7) Is Ubr2 required for Tex19 effects on Orf1p? This could be achieved by overexpressing Tex19 in primary cell lines from Ubr2 mutants (like NSCs or PMEFs).
Published data in the field shows that TEX19.1 protein is unstable and not detectable in the absence of UBR2 in mouse testes (Yang et al., 2010). We performed a similar experiment to the one suggested by the reviewers but took advantage of endogenous TEX19 expression in HCT116 cells rather than using an overexpression experiment. These preliminary experiments showed that TEX19 protein becomes unstable and undetectable in the UBR2 mutant lines (Author response image 1). Therefore, the requirement for UBR2 to maintain TEX19.1 protein stability, and therefore presumably Tex19.1 function (Yang et al., 2010), in mouse germ cells extends to human somatic cells. However, as might be expected, endogenous expression of L1-ORF1p is difficult to detect in somatic cell lines (Philippe et al., 2016, ELife, 13926), and the slow growth and altered cell cycle profiles of the UBR2 mutant lines, which presumably reflects the known functions of this protein in maintaining genome integrity (Ouyang et al., 2006), makes meaningful comparisons with control cells difficult.
https://doi.org/10.7554/eLife.26152.031