Structural insights into the light-driven auto-assembly process of the water-oxidizing Mn4CaO5-cluster in photosystem II
Figures
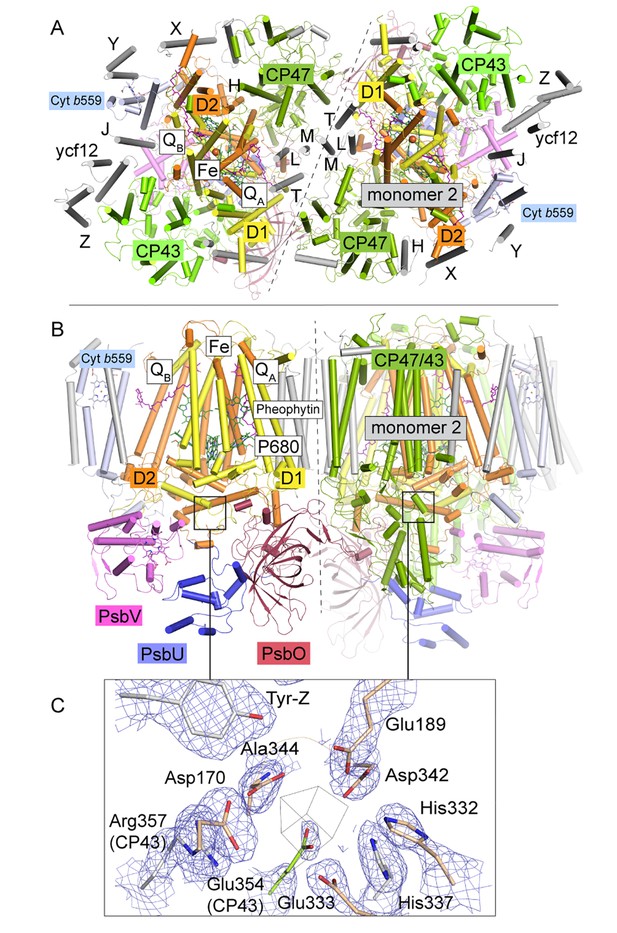
T. elongatus PSIIcc dimers viewed.
(A) from the cytoplasmic side and (B) from the membrane. The reaction centre transmembrane subunits D1/D2, the internal antennae (CP43/CP47, omitted in the left monomer) and the membrane extrinsic subunits PsbU/V/O are highlighted in color. Small transmembrane subunits comprising a single α-helix (two for PsbZ) are indicated by single letter referring to the PsbH-Y proteins and ycf12. QA/B denote the plastoquinone cofactor, Fe the non-heme iron. The site of the water-oxidizing complex (WOC, Mn4CaO5) within each monomer is shown by the black box. (C) Electron density map (1σ 2Fo-Fc) at the depleted WOC site (shown for the locked dimer, discussed below). Free coordinating residues from the D1 subunit (cream) and CP43 (green) are shown alongside Arg357(CP43) and His 337(D1), which are within hydrogen-bond distance to cluster oxygen atoms in Umena et al. (2011), (PDB:3WU2).
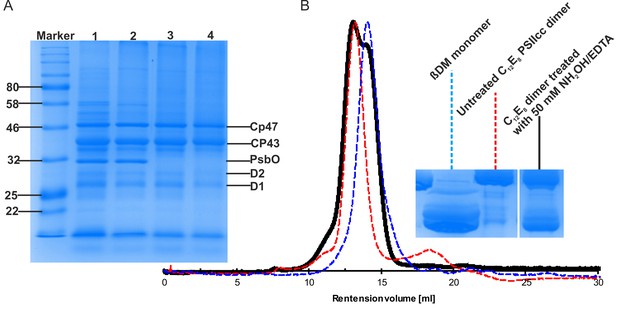
Effect of NH2OH-EDTA treatment performed on dPSIIcc solutions.
(A) SDS-PAGE profiles showing the completeness of large PSII subunits in C12E8 dPSIIcc solutions before (lane 1) and after (lane 2, lane 3 and lane 4) size exclusion chromatography. The dPSIIcc samples were incubated with variable NH2OH/EDTA concentrations in darkness at 4°C for 1–2 hr and then washed three times with MCB buffer without CaCl2. The samples were treated with 0 mM (lane 1, lane 2), 20 mM (lane 3) and 50 mM (lane 4) NH2OH/EDTA. After the treatment as described above PSII samples at 0 mM, 20 mM and 50 mM NH2OH/EDTA were eluted from a size exclusion column shown in lane 2, 3 and 4. B: Gel filtration chromatogram showing the effect of NH2OH/EDTA (50 mM) treatment on monomerization of C12E8 dPSIIcc solution (left panel). Untreated C12E8 dPSIIcc (red, dotted line) and ßDM PSII monomer (blue, dotted line) are used as references. The treated C12E8 dPSIIcc solution using 50 mM NH2OH/EDTA is represented in black. BN-PAGE profiles using the same sample were obtained after gel filtration (right panel).
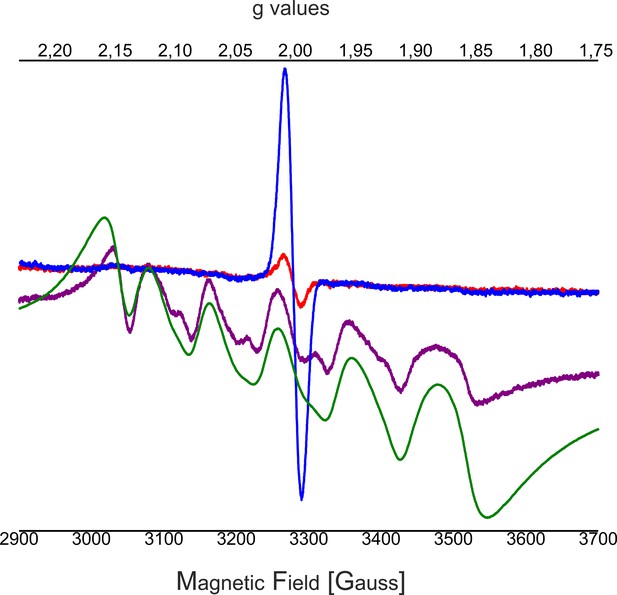
Influence of EDTA added to NH2OH treated C12E8 dPSIIcc solutions.
EPR spectra of untreated dPSIIcc samples (blue trace), 50 mM NH2OH treated dPSIIcc (purple trace), 50 mM NH2OH/EDTA treated dPSIIcc (red trace) and 1 mM dissolved MnCl2 in dPSIIcc (green trace). Dimeric PSIIcc solutions were incubated in the presence of 50 mM NH2OH (purple trace) for 10–15 min at RT in dark. The sample was further washed with 50 mM EDTA (red trace). Samples were measured at 20 K (microwave frequency, 9.22 GHz; field modulation amplitude, 32 G at 100 kHz; microwave power, 1 mW).
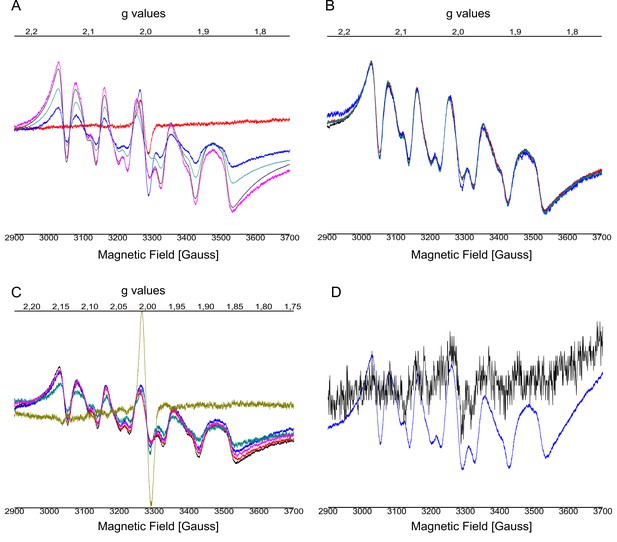
EPR spectra after addition of NH2OH using different concentrations.
(A) 0 mM up to 20 mM and from (B) 20 mM up to 100 mM performed on C12E8 dPSIIcc solutions, from (C) 0 mM to 100 mM performed on C12E8 dPSIIcc microcrystals. EPR spectra (D) of added Mn2+ with apo-WOC-PSIIcc using 10 mM MnCl2 washed with buffers (20 mM MES pH 6.0, 0.5 M betaine and 0.02% C12E8) with (black trace) and without CaCl2 (blue trace). Samples were measured at 20 K (microwave frequency, 9.22 GHz; field modulation amplitude, 32 G at 100 kHz; microwave power, 1 mW). Panel A: PSII solutions were treated with no NH2OH (red trace), 1 mM NH2OH (blue trace), 5 mM NH2OH (blue-green trace), 10 mM NH2OH (black trace) and 20 mM NH2OH (pink trace) and incubated for 10–15 min at RT in dark. Panel B: Samples were treated with 20 mM NH2OH (black trace), 30 mM NH2OH (blue-green trace), 50 mM NH2OH (red trace), 100 mM NH2OH (blue trace) and incubated for 10–15 min at RT in dark. Panel C: Microcrystals were treated with no NH2OH (yellow trace), 20 mM NH2OH (blue-green trace), 30 mM NH2OH (pink trace), 40 mM NH2OH (blue trace), 50 mM NH2OH (red trace), 100 mM NH2OH (black trace) and incubated for 10–15 min at RT in dark. Panel D: PSII solutions containing 10 mM MnCl2 were treated with buffers with (black trace) and without (blue trace) CaCl2. We observe a six-line signal at g ≈ 2 from Mn2+ bound to protein that is still present after repeated washing. The spectra have similar features as intact PSII treated with NH2OH. The sample washed with buffer with CaCl2 shows a weaker signal than the one without CaCl2. After Mn depletion, apo-PSII core complexes bind Mn2+ ions with low affinity at nonspecific sites when the concentration of the MnCl2 used is above the stoichiometric levels and in absence of other divalent ions like Ca2+ or Mg2+. In presence of an excess of another divalent cation, in our case Ca2+ (from CaCl2), the contribution from the Mn2+ ions with low binding affinity can be eliminated (black trace). It has been shown before that Ca2+ plays a role in preventing or reversing the binding of this low-affinity nonfunctional Mn2+ (Chen et al., 1995).
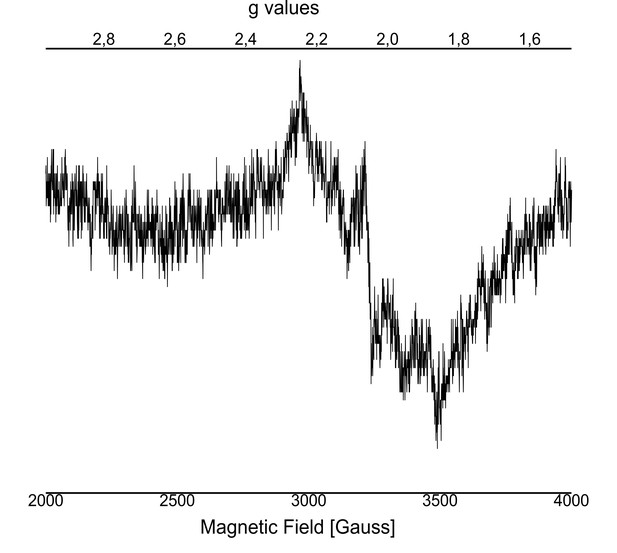
EPR spectra of added Mn2+ with apo-WOC-PSIIcc using 10 mM MnCl2 washed with buffers with CaCl2.
Spectrometer conditions were as follows: microwave frequency, 9.22 GHz; field modulation amplitude, 32 G at 100 kHz; microwave power, 5 milliwatts. Here, we see a broad signal centered near g ≈ 2.04 with peak width of ~ 780 Gauss. In a previous study, this feature was assigned to Mn2+, which binds to apo-PSII at the WOC in the presence of Ca2+. Ananyev and Dismukes (1997) used a double difference spectrum between the reassembled PSII sample washed with CaCl2 and MgCl2 to assign the multiline type spectrum to the 55Mn hyperfine transitions found in spin-coupled dimanganese (II,II) centers. Thus, the broad peak at g ≈ 2.04 may indicate that there are pairs of Mn(II) ions in the WOC in the presence of Ca2+.
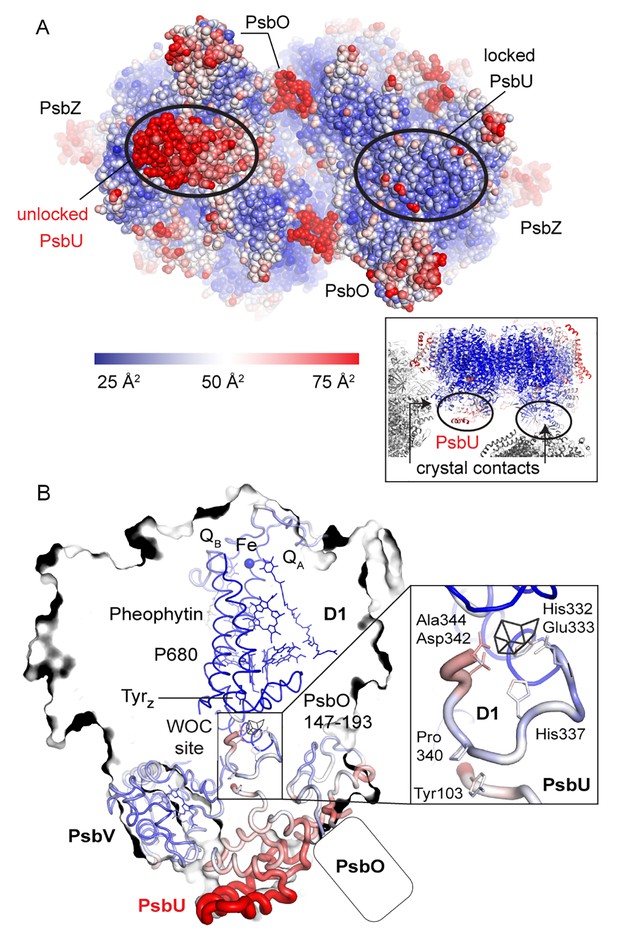
Comparison between the locked and unlocked monomer.
(A) Locked (right) and flexible, unlocked monomer (left). Atomic B-factors are shown using the indicated color coding. The inset shows the crystal contacts acting on PsbU in the right monomer. (B) A slice through the unlocked PSII monomer showing D1 and extrinsic, manganese stabilizing subunits PsbU, PsbV, PsbO (the 147–193 loop and the position of the external β-barrel). The inset shows the WOC-binding site and C-terminal loops of both PsbU and D1 including, residues involved in their interaction (Tyr103 and Pro340) and residues involved in cluster binding in intact PSII.
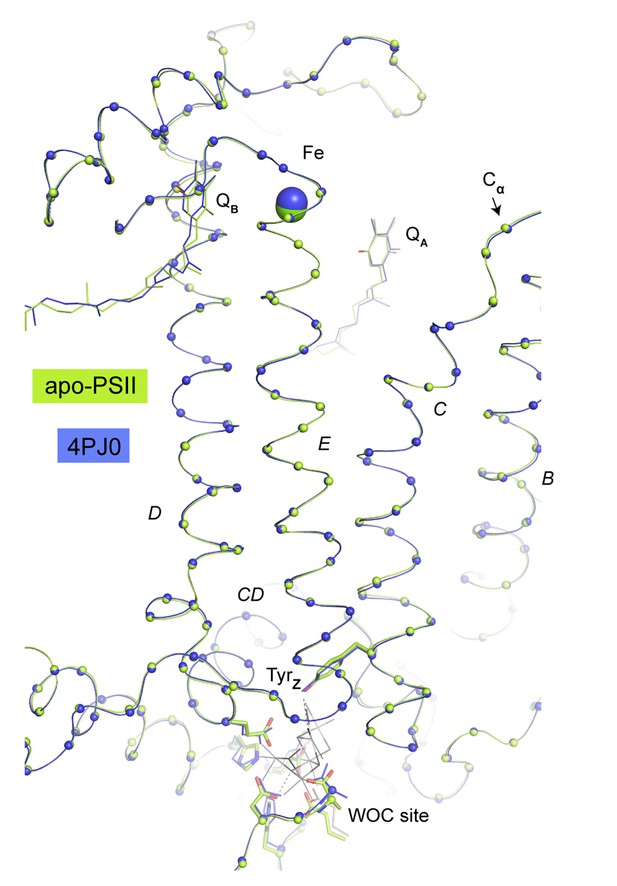
Overlay of Cα atoms (spheres) of D1 residues between the WOC site and the acceptor site (Fe, QA, QB).
The present structure is shown in green, the reference structure from the same crystal system but not treated with NH2OH and EDTA (PDB: 4PJ0) is shown in blue. D1 Helices are labeled according to (Zouni et al., 2001). No consistent changes were observed in helices D and E connecting donor and acceptor site or at the acceptor site itself. We do not detect any major rearrangements that could explain observations linking the redox potential of the primary quinone acceptor, QA, to Ca and/or Mn depletion of the PSII donor side (Krieger and Rutherford, 1997; Allakhverdiev et al., 2011; Brinkert et al., 2016). But this does not preclude more subtle structural changes from asserting such effect. We note that the side chain of Lys264 is insufficiently resolved in order to determine the distance from its Nζ to the carbonate species. The same ambiguity existed in earlier structures and it was unclear if Lys264 was a determinant of the protonation state of bicarbonate at the none-heme iron (discussed in [Brinkert et al., 2016]).
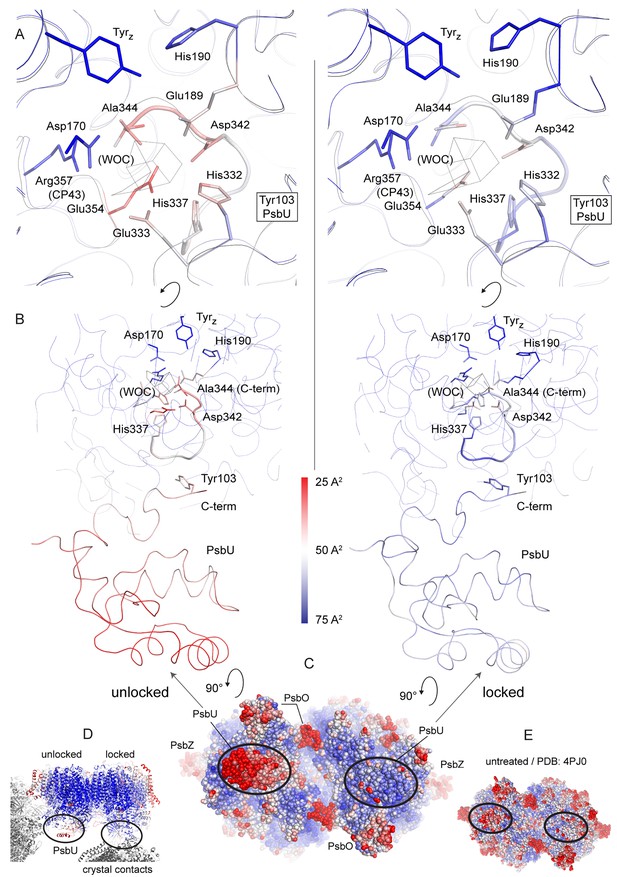
Increase in B-factors (blue, less flexible to red, more flexible) between PsbU and the WOC site in the unlocked (left) monomer, but much less so in the monomer locked by a crystal contact (right).
(A) Side chains binding the WOC in intact PSII, TyrZ and His190. (B) Slice through PSII as oriented in (D). Thick ribbons show the C-terminal loop of D1 and PsbU. (C) PSII viewed from the lumenal side compared to untreated PSII (E). (D) Contacts from symmetry related PSII molecules (grey) in the crystal.
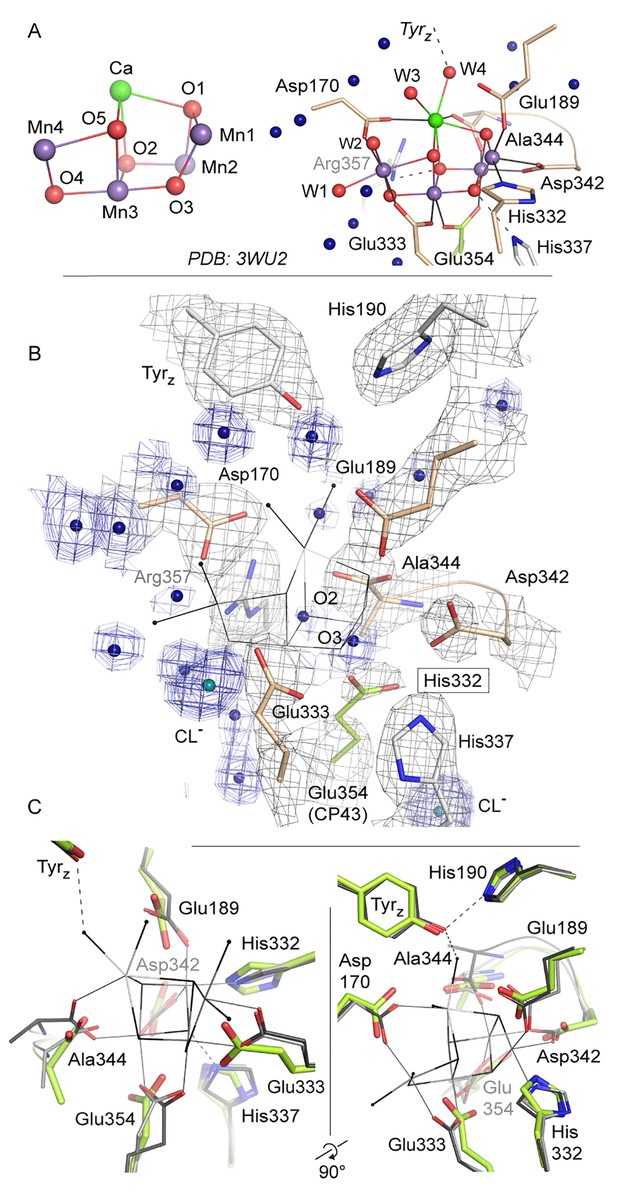
Comparison of intact WOC site and Mn-depleted site.
(A) Coordination of the intact WOC by side chains from the D1 subunit (cream) and CP43 (green) and four water molecules (W1–W4). Arg357(CP43) and His 337(D1) form hydrogen bonds to WOC oxygen atoms (Umena et al., 2011). (B) Model of sidechains and electron density map (1 sigma 2Fo-Fc, grey) of the apo-WOC and electron density (0.8–1.5 sigma 2Fo-Fc, blue) tentatively modeled as waters at the site of the WOC and surrounding water molecules (blue). Multiple contour lines indicate a stronger (more defined) peak in the electron density map (e.g. waters at Asp170), while fewer lines show a weaker signal (e.g. the water at the WOC O2 site). (C) Overlay of apo-WOC model (green) with two holo-PSII models at a similar (PDB: 4PJ0, [Hellmich et al., 2014], light grey) and higher resolution (PDB: 3WU2, [Umena et al., 2011], dark grey).
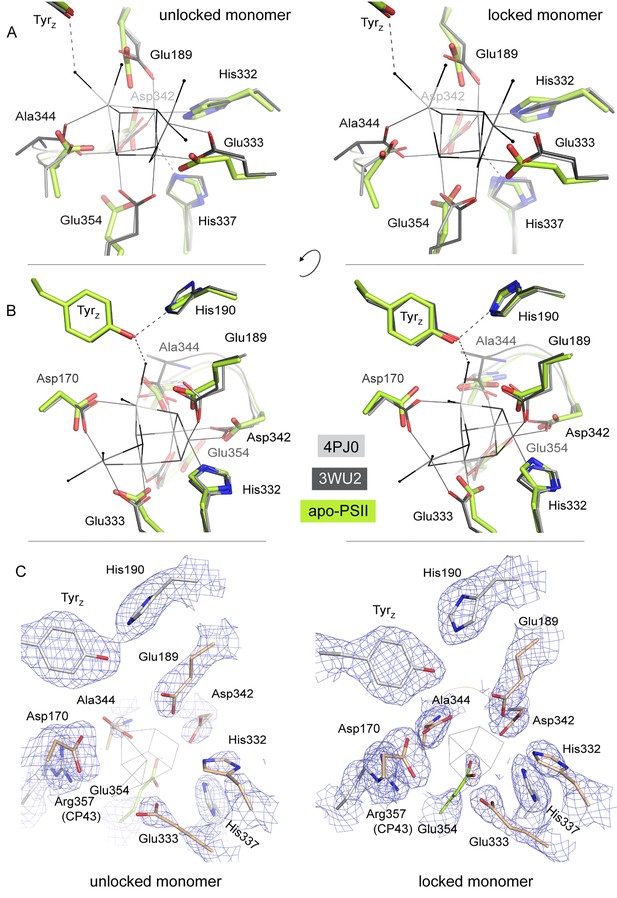
Overlay of the WOC-coordinating side chains at both monomers of dPSIIcc.
(A/B) Overlay of residues coordinating the WOC (thin lines) in two reference structures (4Pj0 Hellmich et al. and 3WU2 Umena et al.) with the same residues in apo-PSII (green). (C) The same residues in apo-PSII. Residues formerly coordinating Mn/Ca are shown in sand (D1) and green (CP43), those within hydrogen-bond distance to μ-oxo bridges in grey. 1 σ 2Fo-Fc map surrounding the side chains is shown as blue mesh. Position of the WOC in 3WU2 is indicated as thin black lines.
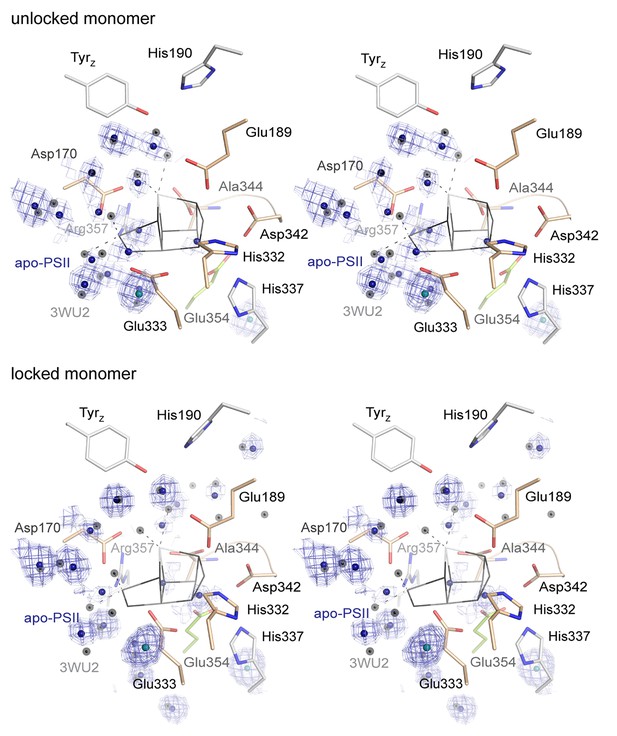
Stereo view of the WOC binding site as shown in Figure 4 but with the 0.8–1.5 σ 2Fo-Fc electron density map (blue) shown at water positions (blue spheres) and chloride positions (emerald spheres) only.
Grey spheres indicate water positions in the high-resolution reference structure 3WU2.
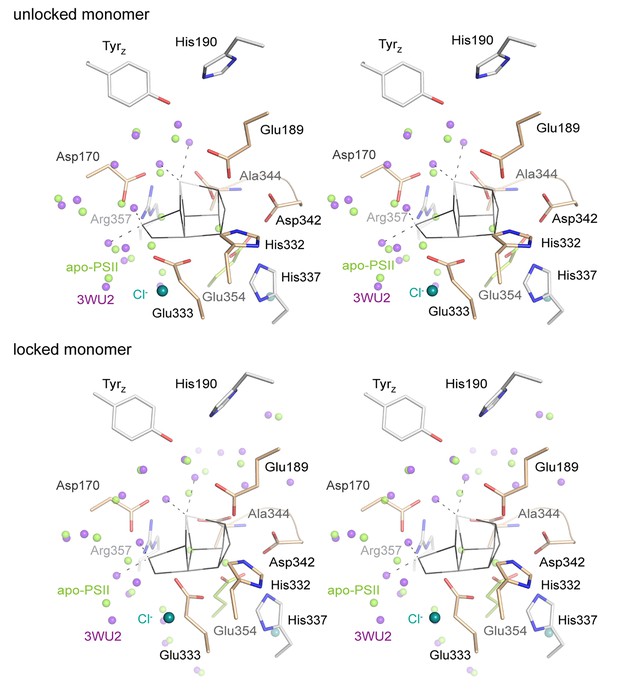
Stereo view comparing water positions at the WOC site in apo-PSII (green) with those in 3WU2 (purple).
Chloride ions are shown in emerald for apo-PSII only.
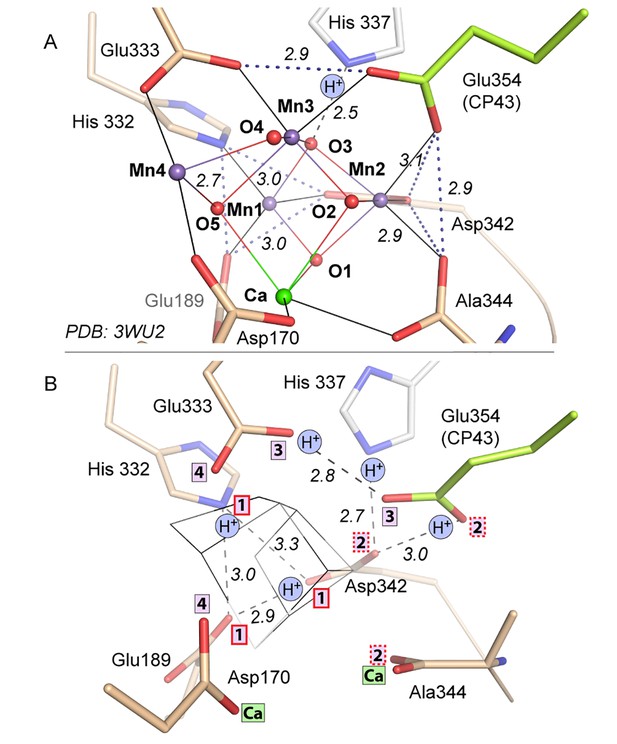
Comparison of intact WOC site and Mn-depleted site.
(A) Intact WOC: Distances (Å) between O/N atoms of residues coordinated to the same metal ion. (B) Apo-WOC: Distances (Å) between O/N atoms which are likely connected by H-bonds. In B, also estimates for likely protonation states are shown. Boxed numbers indicate to which metal ion (Mn1 to Mn4; Ca) the respective O/N binds in the intact WOC. The His337 is H-bonded to a µ3-oxo bridge between Mn1, Mn2, and Mn3. Positions of Glu354 and Ala344 have higher uncertainty or mobility (see also Figures 1C and 4C).
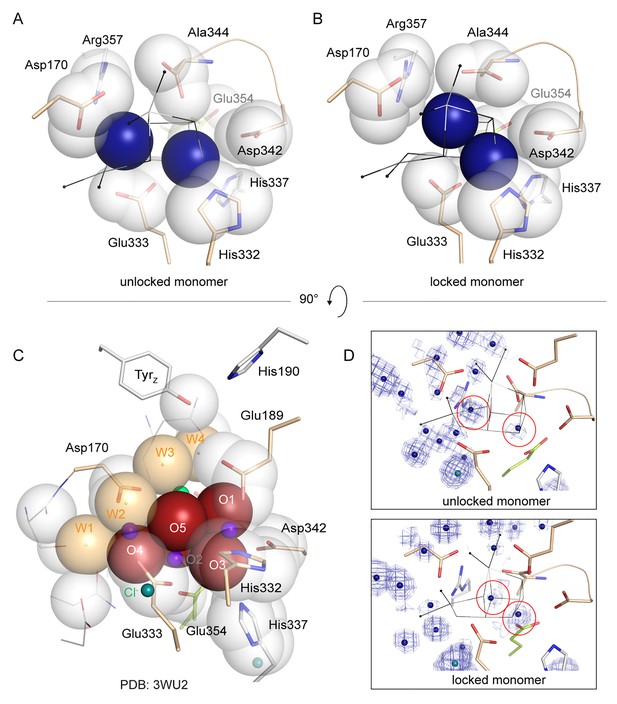
Packing of water molecules in apo-PSII and μ-oxo-bridges in intact PSII.
Proximal side chain atoms are shown as van-der-Waals radii. (A, B) two peaks in the electron density map (circled in D along with 0.8–1.5 σ 2Fo-Fc map) tentatively modeled as waters are shown in blue and plainly present the simplest model. The waters are sufficient to fill the cavity left by the WOC and no further van-der-Waals sphere would fit between them. The right peak is present in both monomers and is located at what appears to be within a short distance (2.3 Å) of His332, while the left peak differs between monomers. Such a short distance may indicate presence of a metal at low occupancy, but the coordinating side chain would share such low occupancy and we would not expect to be able to discern its true position and thus distance in the electron density map. We note that the water structure at the WOC site appears less well defined than surrounding waters (Figure 4—figure supplement 3). This may indicate the presence of multiple states or ions in different PSII molecules within the crystal. (C) Van-der-Waals radii of Mn-complexing O1-O5 and W1-W4 alone represent an ideal packing of spheres in the intact WOC (3WU2).
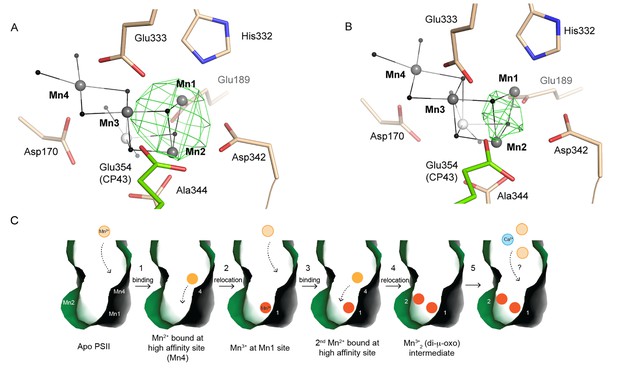
Partially (dis)assembled WOC.
(A) Difference electron density obtained for the partially reconstituted and (B) partially disassembled WOC. Grey spheres indicate the position of manganese atoms in 3WU2 when D1 Cα atoms are superimposed. Green mesh indicates 4.5 σ Fo-Fo(apo) maps at 4.5 Å (A) and 4 Å (B) resolution. (C) Possible Model describing the initial reassembly of the WOC based on the structural data for the partially reconstituted WOC. The scheme represents the binding and the light driven relocation/oxidation of the first two Mn ions into the apo-PSII. The first Mn2+ (light orange) moves to the Mn4 position of the apo-PSII (green) and binds at this high affinity site (1). After light driven oxidation with low quantum efficiency a rearrangement takes place where the oxidized Mn3+ (orange) migrates from the Mn4 position to the Mn1 position (2). The same rearrangement also applies to the second Mn2+ which binds at the Mn4 position and then relocates as the oxidized Mn3+ to the Mn2 position (3, 4). Both Mn3+ ions form a stable Mn1-(µ-O)2-Mn2 assembly intermediate (4), as shown in A). Binding of the two remaining Mn ions and the Ca ion (blue) into the apo-WOC has not been resolved yet (5). Please note that our data do not exclude that Ca2+ could also bind in an earlier step, e.g. in step 3, 4 or 5.
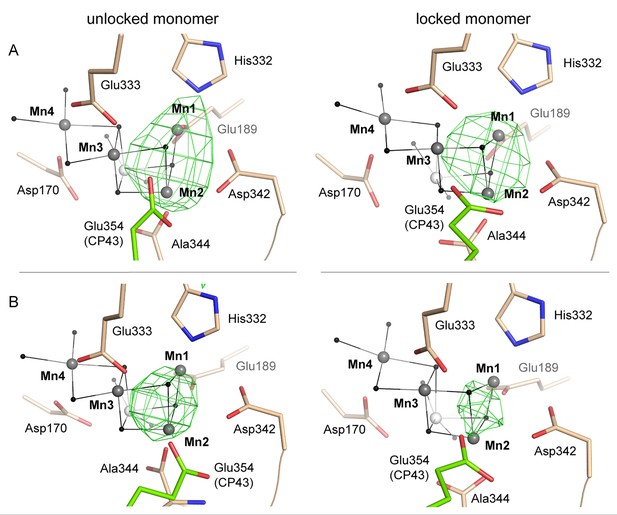
Reconstitution and disassembly of the WOC.
(A) Partially reconstituted and (B) partially disassembled WOC as shown in Figure 6 (right) alongside the unlocked monomer (left). Grey spheres indicate the position of manganese atoms in 3WU2 when D1 Cα atoms are superimposed. Green mesh indicates 4.5 σ Fo-Fo(apo) maps at 4.5 Å (A) and 4 Å (B) resolution. As a result of the limited resolution, it is uncertain if the electron density results from one or more atoms (e.g. Mn1 and Mn2 or a single atom positioned in between in B).
Tables
Data collection and refinement statistics.
2.55 Å data | Extended 2.2 Å data | |
---|---|---|
Data collection | ||
Wavelength | 0.9184 | 0.9184 |
Resolution range | 49.65–2.55 (2.641–2.55) | 49.65–2.197 (2.276–2.197) |
Space group | P 21 21 21 | P 21 21 21 |
Unit cell (Å, °) | 116.33, 219.62, 304.04 90, 90, 90 | 116.33, 219.62, 304.04 90, 90, 90 |
Total reflections | 3348095 (259200) | 4094682 (131257) |
Unique reflections | 252839 (25073) | 364603 (25404) |
Multiplicity | 13.2 (10.3) | 11.2 (5.2) |
Completeness (%) | 1.00 (1.00) | 0.93 (0.65) |
Mean I/sigma(I) | 13.10 (1.85) | 9.34 (0.44) |
Wilson B-factor | 36.00 | 37.11 |
R-merge | 0.208 (1.211) | 0.2500 (3.270) |
R-meas | 0.2163 (1.275) | 0.2621 (3.629) |
CC1/2 | 0.996 (0.752) | 0.993 (0.1320) |
Refinement | ||
R-work | 0.1912 (0.2890) | 0.2115 (0.3998) |
R-free | 0.2414 (0.3250) | 0.2596 (0.4298) |
Number of non-H atoms | 50407 | |
macromolecules | 41206 | |
ligands | 8498 | |
Protein residues | 5428 | |
RMS(bonds) | 0.012 | |
RMS(angles) | 1.17 | |
Ramachandran favored (%) | 96 | |
Ramachandran outliers (%) | 0.33 | |
Rotamer outliers (%) | 1 | |
Clashscore | 7.99 | |
Average B-factor | 36.05 | |
macromolecules | 36.76 | |
ligands | 33.11 | |
solvent | 30.15 |
-
Values in parentheses refer to the highest resolution shell.
-
R-meas: Redundancy-independent merging R-factor (Diederichs and Karplus, 1997).
-
CC1/2: Pearson correlation coefficient of half data sets (Karplus and Diederichs, 2012).
Additional files
-
Supplementary file 1
Effect of NH2OH on inhibition of oxygen evolution rates in intact C12E8-dPSIIcc.
The oxygen evolution was assayed at 25°C using different concentrations of NH2OH from 0 mM to 2 mM. *The rates are the averages of at least three repeated measurements. †The percentage of control rates are given in parentheses.
- https://doi.org/10.7554/eLife.26933.019
-
Supplementary file 2
Completeness of PSII subunits after NH2OH/EDTA treatment.
‡ n-Dodecyl ß-D-maltoside Masses from linear mode MALDI-TOF-MS and assigned to PSIIcc subunits. The experimental determined mass from the C12E8 dPSIIcc treated with 50 mM NH2OH/EDTA is the average mass from spectra recorded from three independent sample preparations. n.d.: not detectable
- https://doi.org/10.7554/eLife.26933.020