High-resolution mapping of heteroduplex DNA formed during UV-induced and spontaneous mitotic recombination events in yeast
Figures
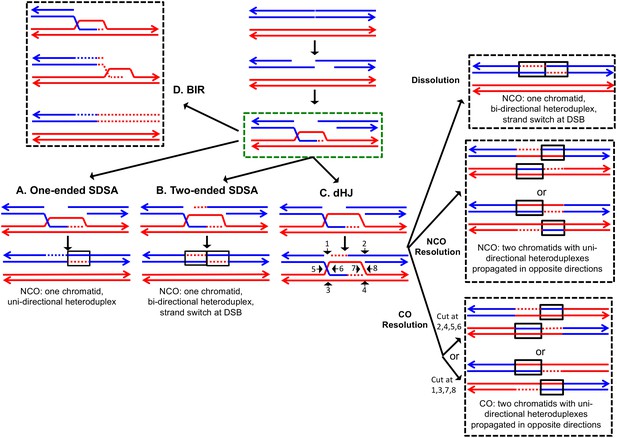
The DSBR repair pathways.
The chromatids are shown as double-stranded molecules with arrows at the 3’ ends. The broken chromatid is colored blue and the intact template chromatid is red. Dotted lines indicate newly-synthesized DNA. Regions of heteroduplex DNA (hetDNA) are outlined by black boxes. All pathways are initiated by invasion of one processed broken end into the unbroken chromatid, forming a D-loop. (A) One-ended Synthesis-dependent strand annealing (SDSA). Following DNA synthesis primed by the invading 3’ end, the invading end dissociates from the intact molecule, and re-anneals with the second broken end. The net result is an NCO event with hetDNA extending uni-directionally from the break. (B) Two-ended SDSA. In this mechanism, following DNA synthesis from the invading end, the other broken end pairs with the D-loop and initiates DNA synthesis. Before ligation to generate a dHJ intermediate, the invading end disengages from the template and re-pairs with the other broken end. The net result is a single chromatid with a bi-directional heteroduplex that has a strand switch at the site of the initiating DSB. (C) Double Holliday junction (dHJ) intermediate. Following DNA synthesis from one invading end, the second broken end pairs with the D-loop (second-end capture). This intermediate can be dissolved by migrating the two junctions inwards, followed by decatenation; this pathway results in a NCO with a bi-directional heteroduplex, identical to the product of the two-ended SDSA mechanism. Alternatively, it can also be resolved by cutting the junctions symmetrically to generate NCOs (cuts at arrows marked 1, 2, 3, and 4 or 5, 6, 7, and 8), or asymmetrically (cuts at arrows marked 2, 4, 5, and 6 or 1, 3, 7 and 8) to generate COs. For both types of resolution, the heteroduplexes are located on different chromatids, flanking the DSB site, and pointing in opposite directions. Note that cuts at positions 1, 3, 7, and eight reflect nick-directed resolution of the junctions. (D) Break-induced replication (BIR). In this pathway, one broken end invades the intact template and copies DNA sequences by conservative replication to the end of the template. The other broken end is lost. Except for the initial strand invasion, heteroduplex intermediates are not relevant to this pathway.
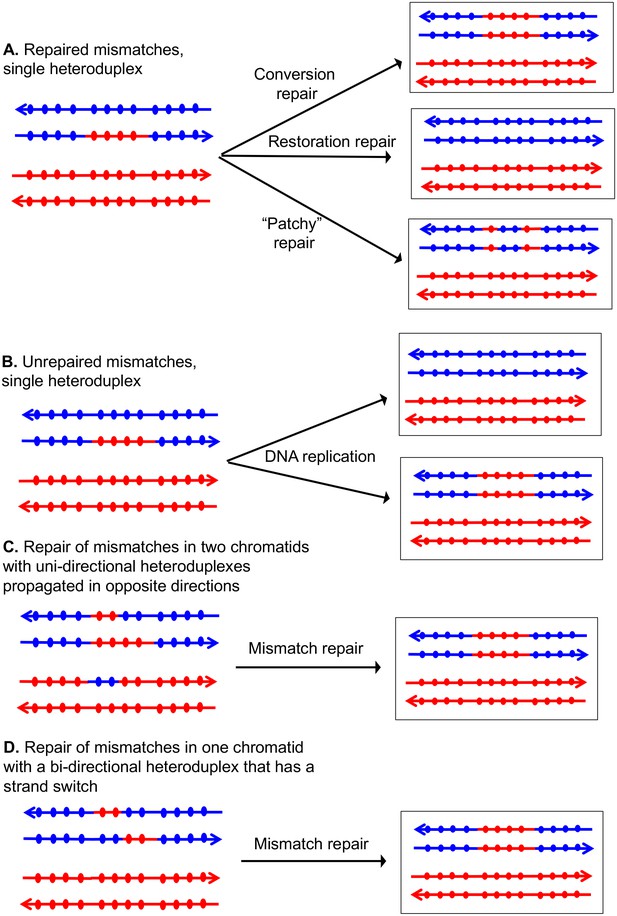
Patterns of mismatch repair in a MMR-proficient strain.
Chromatids are shown as double-stranded DNA structures with circles indicating SNPs. For all parts of the figure, the blue chromatid is broken and, consequently, is the recipient of sequence information from the red chromatid. For these examples, all intermediates were resolved as NCO events. In Figures 2A, C and D, the products shown on the right side of each panel are a consequence of mismatch repair in the mother cell. In Figure 2B, the products are shown in two daughter cells following replication of the chromosomes of the mother cell. (A) Repair of mismatches within a single heteroduplex. In the top panel, the mismatches in the heteroduplex are repaired using the bottom strand as a template, resulting in a conversion event. In the middle panel, the upper strand is used as a template for MMR, resulting in a blue chromatid that is identical to an unbroken chromatid. In the bottom panel, some mismatches undergo conversion-type repair and others restoration-type repair. (B) Loss of mismatches as a consequence of DNA replication. Replication of a chromatid with a heteroduplex results in one product that appears to have undergone conversion-type repair, and a second that is the same as a chromatid without a recombination event. (C) Repair of mismatches intwo chromatids with uni-directional heteroduplexes propagated in opposite directions. If mismatches within the two heteroduplexes are repaired using the red strand as a template, a conversion event that is identical to that shown in the upper panel of Figure 2A would be generated. (D) Repair of mismatches in a one chromatid with a bi-directional heteroduplex that has a strand switch. If all mismatches are repaired using the red strand as a template, the resulting conversion product is identical to that shown in Figure 2A and C.
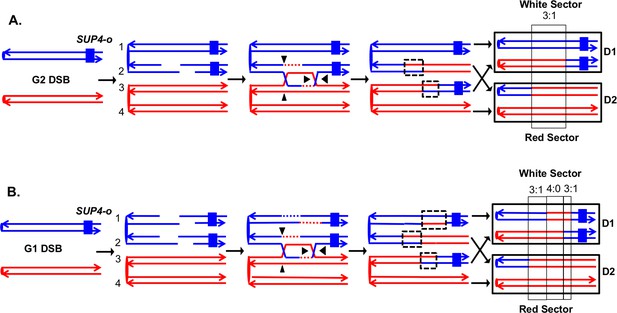
Detection and analysis of crossovers induced by DSBs in single chromatids or in unreplicated chromosomes in an MMR-proficient diploid.
The lines show the two strands of each chromosome/chromatid, and ovals indicate centromeres. The strain is homozygous for ade2-1, an ochre allele that, in the absence of the SUP4-o ochre suppressor, forms a red colony. In the strains used in our study, one copy of SUP4-o is inserted near the telomere of one homolog. Strains with zero, one or two copies of the suppressors form red, pink and white colonies, respectively. Black triangles indicate the positions of cleavages of the dHJ. Heteroduplex intermediates are enclosed by dotted black lines, and conversion tracts are enclosed in thin black lines. Chromosomes in daughter cells are outlined by thick black rectangles, and D1 and D2 denote the two daughter cells resulting from the crossover. (A) Crossover initiated by a single chromatid break (SCB). Following the crossover, segregation of one recombined and one parental chromatid into each cells will generate one cell homozygous for SUP-o and one cell lacking SUP4-o; subsequent divisions will lead to a red/white sectored colony. If mismatches in both heteroduplexes undergo conversion-type repair, a 3:1 conversion event would be observed; within the boxed region, three chromatids have information derived from the red chromatid and one has information derived from the blue chromatid. (B) Crossover initiated by a DSB in an unreplicated blue chromosome, resulting in double sister-chromatid breaks (DSCB). DNA replication of the broken chromosome would result in,two broken sister chromatids. We show the middle pair of chromatids repaired as a crossover, and the top chromatid repair by an SDSA event. In this example, we show the heteroduplex associated with the SDSA event as longer than those of the CO-associated hetDNAs. If all of mismatches are repaired as conversion events, we would see a hybrid 3:1/4:0/3:1 conversion tract. The 4:0 pattern is diagnostic of a DSCB event.
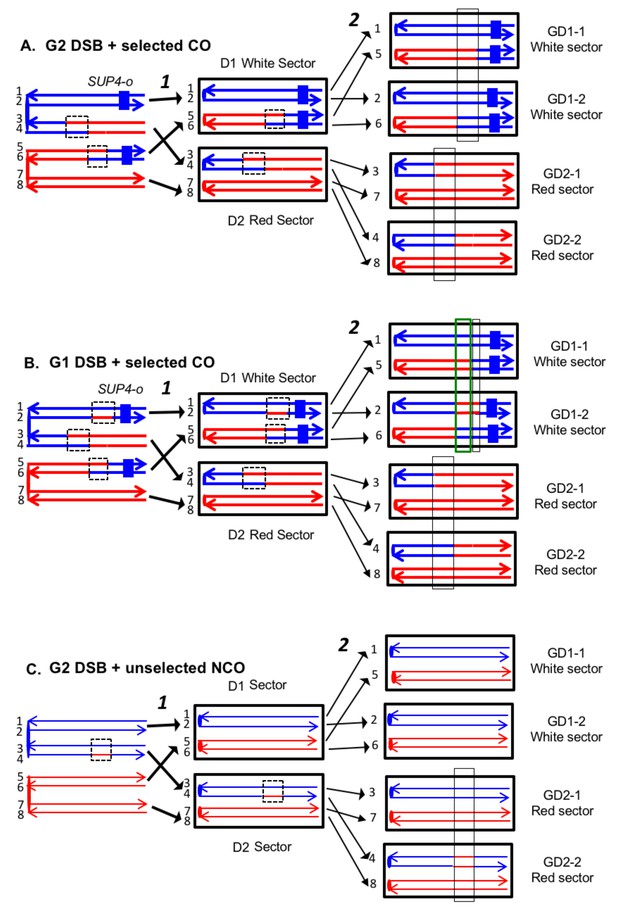
Expected recombination-associated segregation patterns of SNPs into daughter and granddaughter cells in MMR-deficient strains following a G2- or G1-induced DSB.
We show heteroduplexes with unrepaired mismatches (outlined in dashed lines) in the daughter cells D1 and D2. Replication of unrepaired mismatches in heteroduplexes results in granddaughter cells with two different genotypes; these differences are outlined in the right side of the figure by thin black lines. The events shown in Figure 4A and B were selected because the crossovers between the heterozygous SUP4-o marker generated daughter cells that had two copies (resulting in a white sector) or no copies (resulting in a red sector) of SUP4-o. The NCO event shown in Figure 4C was on a homolog that did not contain the SUP4-o marker (shown in thin red and blue lines) and was unselected. (A) CO-associated SCB (selected crossover). If the crossover occurred by the DSBR pathway, we would expect that both sectors would have granddaughter cells with different SNP patterns. (B) CO-associated DSCB (selected crossover). As in Figure 4A, both sectors would have granddaughter cells with different SNPs. One distinguishing feature of the DSCB is that both granddaughter cells of one sector (GD1-1 and GD1-2) have different coupling relationships for SNPs in the same region as outlined in the green rectangle. (C) NCO-associated SCB (unselected). Strains treated with UV have many unselected events. Since these events are induced by UV at the same time as the selected event, we can use the information identifying GD1-1, GD1-2. GD2-1, and GD2-2 from the selected event to determine the patterns of heteroduplex formation for the unselected event by using whole-genome SNP arrays. In the depicted event, only one of the sectors had granddaughters with different genotypes.
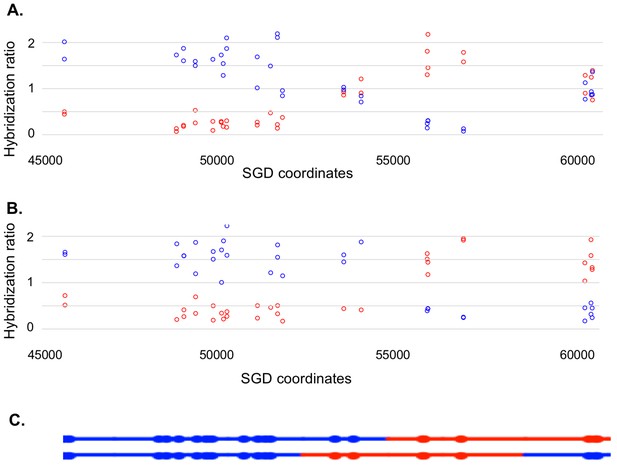
Microarray analysis of one granddaughter cell in a sectored colony resulting from a recombination event on chromosome V.
By methods described in the text, we identified two granddaughter genotypes associated with the red and white sectors of the YYy310-9-5WR sectored colony. SNP-specific microarrays were done on genomic DNA isolated from all four granddaughter strains, and from spore derivatives of each of these granddaughters. (A) Microarray analysis of the W1 granddaughter strain. Genomic DNA was isolated, and hybridized to SNP-specific microarrays in competition with differentially-labeled genomic DNA from a heterozygous control strain (details in Materials and methods). The Y axis shows the hybridization ratio of the experimental samples to allele-specific SNPs normalized to the hybridization levels of the control heterozygous sample; blue and red circles indicate hybridization ratios to YJM789- and W303-1A-specific SNPs, respectively. Heterozygous samples have a ratio of about 1, samples in which the strain-specific allele is present in two copies have a ratio of about 1.7, and those in which the strain-specific allele is missing have a ratio of about 0.3. The X axis has SGD coordinates for chromosome V in the region of the recombination event. Between the left telomere (coordinate 1) and coordinate 51915, the W1 granddaughter is homozygous for the YJM789-derived SNPs, and heterozygous for SNPs between coordinates 60701 and the right telomere. In summary, chromosome V in this strain has a large terminal region that is homozygous for YJM789 SNPs, a short region of heterozygous SNPs, a short region that is homozygous for W303-1A SNPs, and a large heterozygous region that makes up the remainder of the chromosome. (B) Microarray analysis of a spore derived from the W1 granddaughter strain. The W1 diploid was sporulated, and dissected. We examined the genomic DNA of one spore by SNP microarrays. From the pattern of SNPs in the spore, we conclude that one homolog in W1 has blue SNPs for the heterozygous region located near coordinates 55000 and red SNPs for the heterozygous region located between coordinates 60000, and the right telomere. The other homolog has the reciprocal pattern. (C) Inferred arrangement of red and blue SNPs on the two homologs of W1 based on the microarray results shown in Figure 5A and B. This pattern is reproduced in the top part of Supplementary file 2 - S8.
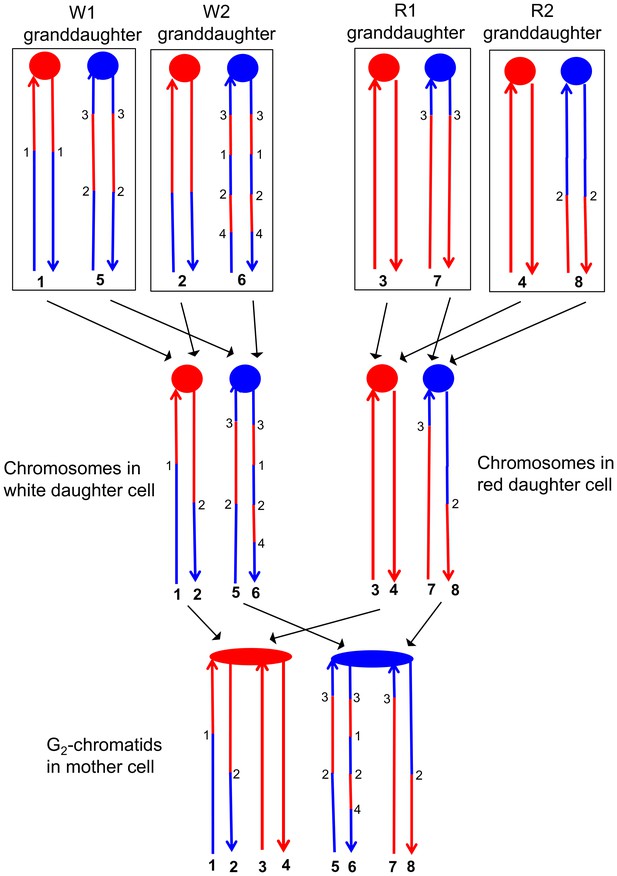
Correlation of SNP patterns in granddaughter cells with heteroduplex patterns in the mother cell.
The example shown is the same crossover on chromosome V described in Figure 5 (YYy310-9-5WR sectored colony). Each chromatid/chromosome is shown as a double-stranded DNA molecule with ovals and circles showing the centromeres. As in Figure 5, red and blue lines signify sequences derived from W303-1A and YJM789, respectively. By the methods described in the text, we identified two genotypes within each sector. The microarray analysis illustrated in Figure 5 allowed us to determine the patterns of LOH in each granddaughter cell (top of the figure). Each chromosome in the granddaughter cell was derived by replication by chromosomes in the daughter cells (middle of the figure). Chromosomes with the same color of centromere in the granddaughter cells must derive from a daughter chromosome with a centromere of the same color. Numbers in bold represent chromatids (top part of figure) or individual DNA strands (bottom part of the figure). For example, chromatids 1 and 2 in the W1 and W2 granddaughters must represent the strands labeled 1 and 2 in the white daughter cell. A similar procedure can be used to assign the strands to the chromosomes in all of the daughter cells. The chromosomes in the daughter cells represent segregants of the G2 chromatids in the mother cell. The daughter chromosomes with red centromeres must have been derived from the paired sister chromatids with the red oval centromere, and a similar conclusion can be drawn about the blue centromeres of the daughter chromosomes and the oval blue centromere in the mother cell. By the pattern of SNPs, we can also conclude that the chromatids in the mother cell with strands 1 and 2, and 7 and 8 were involved in a CO, whereas the other two chromatids were involved in the NCO mode of repair. Numbers that are not in bold represent transitions between W303-1A-derived SNPs and YJM789-derived SNPs; transitions with the same number have identical breakpoints with the resolution of our microarray analysis.
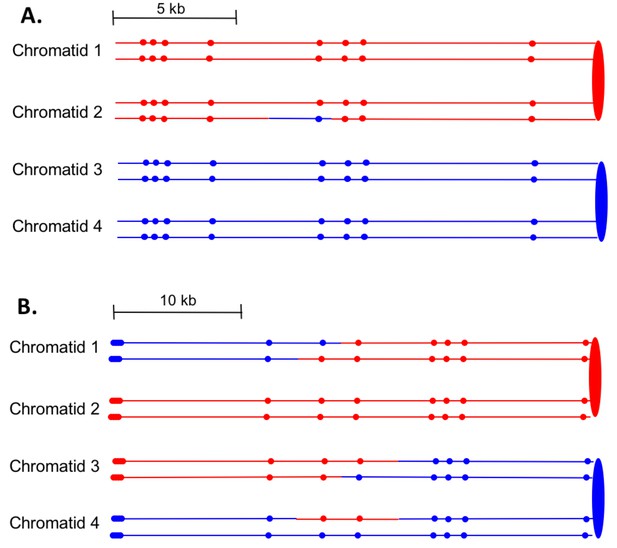
Examples of heteroduplex patterns associated with SCB- and DSCB-initiated events.
Both the SCB event shown in 7A and the DSCB event shown in 7B were unselected events from the same sectored colony (YYy310-905WR; Supplementary file 1 - Table S3). The 7A event occurred on chromosome IV (labeled IV-2 in Supplementary file 1 - Table S3), and the 7B event was on chromosome XIV. The distances between SNPs (shown as red or blue circles) are to the scale shown above each set of chromatids. (A) Inferred pattern of heteroduplexes in an SCB event. The patterns of markers in the granddaughter cells that define the patterns inferred in the G2 mother cell are in Supplementary file 2 - S6. (A) Inferred pattern of heteroduplexes in an DSCB event. The patterns of markers in the granddaughter cells that define the patterns inferred in the G2 mother cell are in Supplementary file 2 - S16.
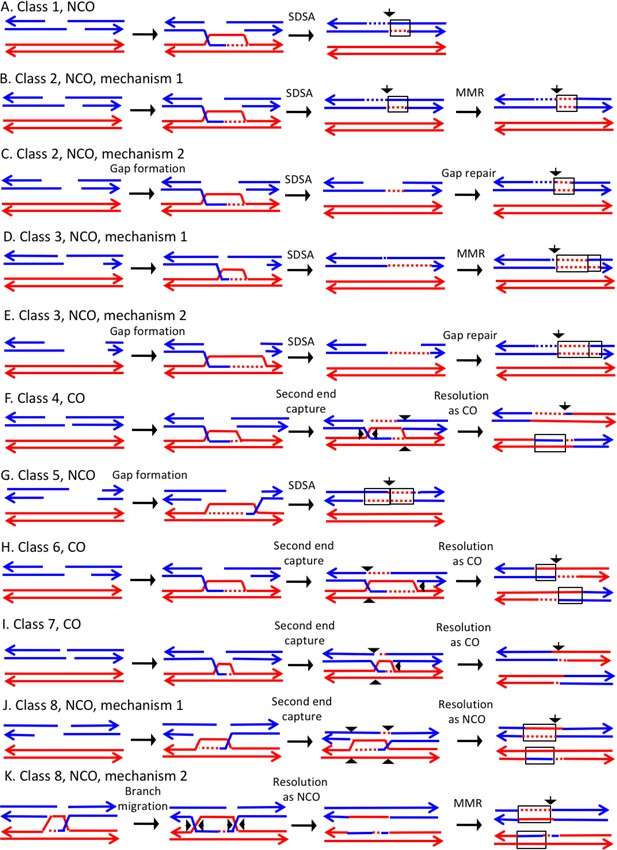
Mechanisms that generate Classes 1–8 recombination events.
For all events, we show the initiating DSB on the blue chromatid. Dotted lines show sequences generated by replication or during mismatch repair. Black arrows indicate the position of the initiating DSB. The mechanisms are discussed in detail in the main text. Red and blue lines represent DNA strands of the W303-1A-derived chromatid and YJM789-derived chromatid, respectively. Arrows on these strands indicate the 3’ ends. (A) Class 1. Class one events are NCOs formed by one-ended SDSA. (B) Class 2. These NCO events could be generated by one-ended SDSA, followed by Mlh1p-independent MMR. (C) Class 2. An alterative possibility is that Class two events reflect repair of a double-stranded DNA gap. (D) Class 3. In this NCO class, the heteroduplex region is adjacent to a conversion tract. Such events could reflect a heteroduplex tract in which mismatches are repaired in one part of the tract and left unrepaired in the other. (E) Class 3. An alternative model for this class is that the conversion tract is the result of repair of a double-stranded DNA gap with a heteroduplex region at one end. (F) Class 4. For this CO class, a heteroduplex is observed on one chromatid but not the other. This class could be explained by the DSBR pathway in which heteroduplex region is short relative to the other; if the short heteroduplex does not contain a mismatch, it would be undetectable. (G) Class 5. In this NCO class, the heteroduplex region is located on the opposite of the DSB site from the conversion region. This pattern is consistent with the repair of a double-stranded DNA gap that was restricted to one of the broken ends. (H) Class 6. This CO class is identical to the pattern expected for the DSBR model. (I) Class 7. In this CO class, no heteroduplexes or conversion tracts are observed adjacent to the crossover, consistent with the formation of a dHJ with short heteroduplex tracts that do not include mismatches. (J) Class 8. In this NCO class, one chromatid has a conversion tract, and the other chromatid has a heteroduplex involving SNPs at the same position. This event could reflect resolution of a dHJ intermediate in the NCO model in which one region of heteroduplex is undetectable. (K) Class 8. An alternative mechanism involves branch migration of a HJ, followed by resolution of the intermediate in a NCO mode. Mismatches in one of the two chromatids are repaired to generate a conversion tract.
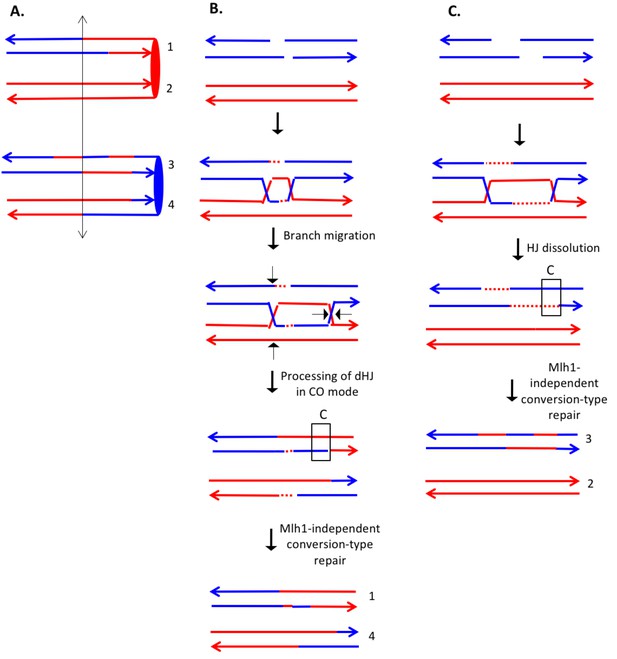
Complex DSCB event derived from analysis shown in Supplementary file 2 - S8.
We infer that the recombination-initiating DSB was on a blue chromosome that was replicated to produce two broken blue chromatids. (A) Depiction of heteroduplexes and conversion tracts present in the mother cell before chromosome segregation. The double-headed arrow indicates the position of the initiating DSB. (B) Mechanism to produce the CO chromatids 1 and 4. Following invasion of the left end of the broken chromatid, one HJ underwent branch migration resulting in a region of symmetric heteroduplexes. The dHJ intermediate was processed to yield a CO as shown by the short arrows. Mismatches in a region of the heteroduplex on the upper chromatid were repaired to yield a conversion event. We suggest that the small homoduplex region near the DSB site did not include a SNP and was, therefore, undetectable. (C) Mechanism to produce the NCO chromatids 2 and 3. Following dHJ formation, the intermediate was dissolved, resulting in bidirectional heteroduplexes on chromatid 2. In addition, as in Figure 8B, we hypothesize that there was a region of mismatch repair in one of the heteroduplexes.
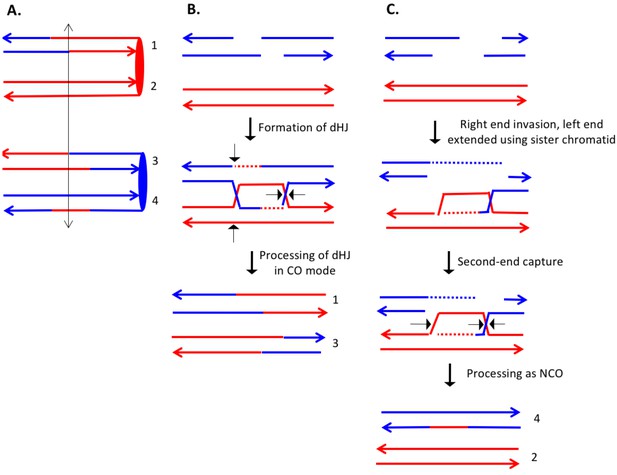
Complex DSCB event derived from the analysis shown in Supplementary file 2 - S16.
As in Figure 8, the initiating DSB was on the blue chromosome. (A) Depiction of recombinant chromatids in mother cell before segregation of chromosomes into the daughters. (B) CO between chromatids 1 and 3. The pattern of heteroduplexes on these chromatids is that expected from the DSBR model. (C) NCO between chromatids 2 and 4. In this event, the heteroduplex spans the putative DSB site on chromatid 4. The right broken end underwent resection of both strands before strand invasion. The left end was extended by invasion of the sister chromatid. Following annealing of the extended end to the D-loop, the intermediate was processed as a NCO. We suggest that the small region of heteroduplex on the red chromatid did not contain a SNP and was not detectable.
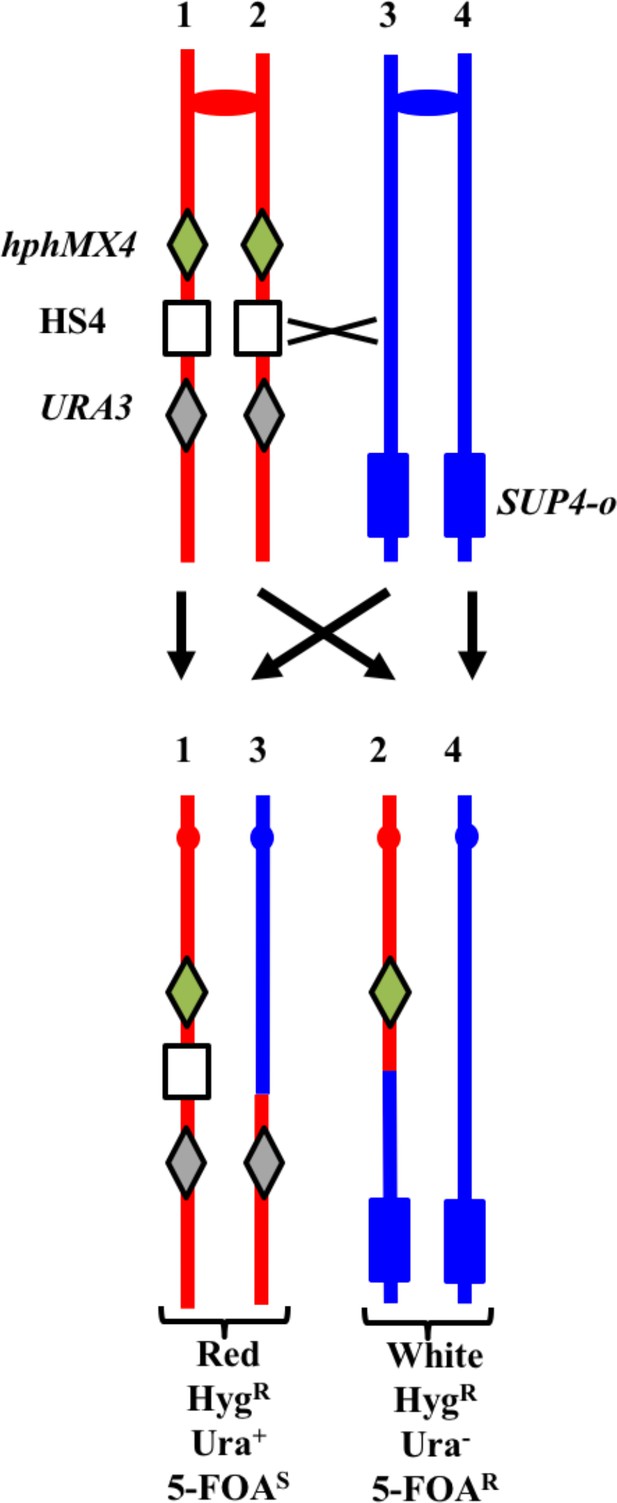
System for detecting crossovers at the HS4 hotspot on chromosome IV (strain YYy311).
The W303-1A- and YJM789-derived chromatids are shown in red and blue, respectively. On the W303-1A-derived homolog, the HS4 hotspot is flanked by the hphMX4 and URA3 markers; SUP4-o is located near the telomere of the YJM789-derived homolog. Since the recombinogenic DSB is located at HS4, HS4-initiated events usually result in loss of HS4 as shown.
Tables
Description of classes of 'simple' recombination events and number of observed events in each class.1
Class | Description of class | Example | Number observed |
---|---|---|---|
1 | NCO, unidirectional heteroduplex, no strand switch; one-ended SDSA, Figure 1A | Chromatid 2 of Figure 7A | 26 |
2 | NCO, single continuous conversion tract on one side of DSB site | Chromatid 3 of Supplementary file 2 - S4 | 18 |
3 | NCO, hybrid heteroduplex/conversion tract located on one side of DSB site | Chromatid 4 of Supplementary file 2 - S5 | 12 |
4 | CO, heteroduplex on only one chromatid on one side of DSB site | Chromatids 1 and 4 of Supplementary file 2 - S21 | 5 |
5 | NCO involving only one chromatid with heteroduplex on one side of DSB and conversion tract on the other | Chromatid 3 of Supplementary file 2 - S14 | 4 |
6 | CO, uni-directional heteroduplexes on both chromatids propagated in opposite directions | Chromatids 1 and 3 of Supplementary file 2 - S16 | 2 |
7 | CO, no detected heteroduplex or conversion tract on either chromatid | Chromatids 1 and 3 of Supplementary file 2 - S24 | 2 |
8 | NCO, one chromatid with uni-directional conversion tract and one chromatid with uni-directional heteroduplex on same side of DSB | Chromatids 2 and 3 of Supplementary file 2 - S67 | 2 |
-
1These classes are further described in the text and are depicted in Figure 8.
Additional files
-
Supplementary file 1
Supplementary Tables S1-S9.
- https://doi.org/10.7554/eLife.28069.014
-
Supplementary file 2
- S1 Mechanisms that generate Classes 1–5 recombination events.
For all events, we show the initiating DSB on the blue chromatid. Dotted lines show sequences generated by replication or during mismatch repair. Black arrows indicate the position of the initiating DSB. The mechanisms are discussed in detail in the main text. Red and blue lines represent DNA strands of the W303-1A-derived chromatid and YJM789-derived chromatid, respectively. Arrows on these strands indicate the 3’ ends. A. Class 1. Class one events are NCOs formed by one-ended SDSA. B. Class 2. These NCO events could be generated by one-ended SDSA, followed by Mlh1p-independent MMR. C. Class 2. An alterative possibility is that Class two events reflect repair of a double-stranded DNA gap. D. Class 3. In this NCO class, the heteroduplex region is adjacent to a conversion tract. Such events could reflect a heteroduplex tract in which mismatches are repaired in one part of the tract and left unrepaired in the other. E. Class 3. An alternative model for this class is that the conversion tract is the result of repair of a double-stranded DNA gap with a heteroduplex region at one end. F. Class 4. For this CO class, a heteroduplex is observed on one chromatid but not the other. This class could be explained by the DSBR pathway in which heteroduplex region is short relative to the other; if the short heteroduplex does not contain a mismatch, it would be undetectable. Supplementary file 2 - S2. Mechanisms that generate Classes 6–8 recombination events. Events are depicted as in Supplementary file 2 - S1. A. Class 5. In this NCO class, the heteroduplex region is located on the opposite of the DSB site from the conversion region. This pattern is consistent with the repair of a double-stranded DNA gap that was restricted to one of the broken ends. B. Class 6. This CO class is identical to the pattern expected for the DSBR model. C. Class 7. In this CO class, no heteroduplexes or conversion tracts are observed adjacent to the crossover, consistent with the formation of a dHJ with short heteroduplex tracts that do not include mismatches. D. Class 8, mechanism 1. In this NCO class, one chromatid has a conversion tract, and the other chromatid has a heteroduplex involving SNPs at the same position. This event could reflect resolution of a dHJ intermediate in the NCO model in which one region of heteroduplex is undetectable. E. Class 8, mechanism 2. An alternative mechanism involves branch migration of a HJ, followed by resolution of the intermediate in a NCO mode. Mismatches in one of the two chromatids are repaired to generate a conversion tract. Supplementary file 2 - S3-S91. In the upper part of the figure (labeled A), we show the patterns of SNPs derived from the W303-1A homolog (red) and YJM789 homolog (blue). Each line represents a chromosome. Each pair of lines is derived from one of the granddaughter cells in the white (W1 or W2) or red (R1 or R2) sectors. The locations of the SNPs, represented by colored circles, are drawn proportionally with a scale showing SGD coordinates at the bottom of A. In the B part of the figures, we show the arrangement of SNPs in double-stranded chromatids in the mother cell before chromosome segregation; in this part of the figure, each line represents a single-strand of DNA of the chromatids. The inferred positions of the initiating DSBs are indicated with arrows. Chromatids 1 and 2 are sisters, and chromatids 3 and 4 are sisters. In part C, for recombination events that are classified as 'Complex', we show possible mechanistic pathways for their formation. Supplementary file 2 - S3-S80 show selected and unselected UV-induced events in strain YYy310. Supplementary file 2 - S81-S85 show selected spontaneous events on chromosome V in strain YYy310, and Supplementary file 2 - S86-S91 represent spontaneous HS4-related crossovers on chromosome V in strain YYy311. The coordinates and classifications of the events represented by these supplemental figures are in Supplementary file 1 - Tables S2 and S3, respectively. Lastly, we note that the event depicted in Supplementary file 2 - S71 has the pattern expected for a BIR event in one of the daughter cells. Because of this complication, we did not attempt to infer the locations of the initiating DSBs.
- https://doi.org/10.7554/eLife.28069.015