Cis-regulatory evolution integrated the Bric-à-brac transcription factors into a novel fruit fly gene regulatory network
Figures
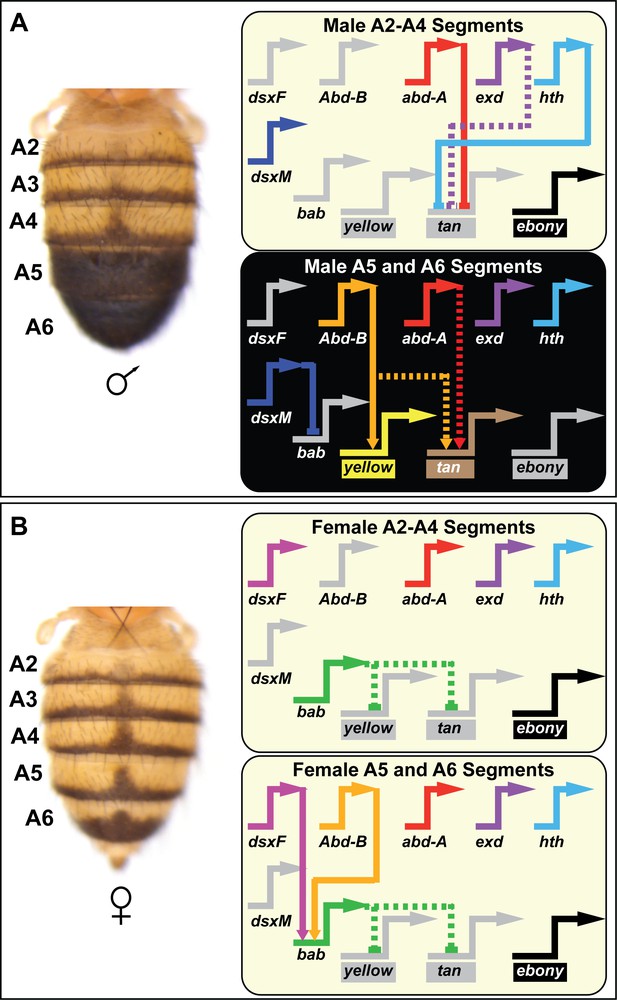
Contemporary model for the D. melanogaster tergite pigmentation Gene Regulatory Network.
Melanic tergite pigmentation requires the specific expression of the pigmentation genes yellow and tan, while blocking the expression of the yellow-pigment promoting ebony gene. (A) Tergite pigmentation pattern for a D. melanogaster male and the regulatory interactions experienced in the non-melanic male A2-A4 segments (top) and the melanic A5 and A6 segments (bottom). Abd-B is not expressed in the anterior A2-A4 segments and resultantly yellow and tan lack the direct and indirect activating input. In these anterior segments, Abd-A acts as a direct repressor of tan in combination with (direct or indirect) repressive effects of exd and hth. Abd-B is expressed in the posterior A5 and A6 segments, where it functions as a direct activator of yellow and an indirect activator of tan. In these segments, Abd-A acts as an indirect activator of tan. (B) Tergite pigmentation pattern of a D. melanogaster female and the key regulatory inputs experienced in the A2-A4 segments (top) and the A5 and A6 segments (bottom). In the female abdomen, Bab acts as a dominant repressor of yellow and tan expression, overriding the presence of Abd-B and Abd-A. In the GRN schematics, inactive genes are indicated in gray coloring, solid lines connecting genes indicate established direct interactions between a transcription factor and a target gene’s CRE, and dashed connections indicate indirect regulatory interactions or interactions not yet shown to be direct. Lines terminating with an arrowhead indicate regulation in which the transcription factor functions as an activator, and lines terminating in a nail-head shape indicate repression.
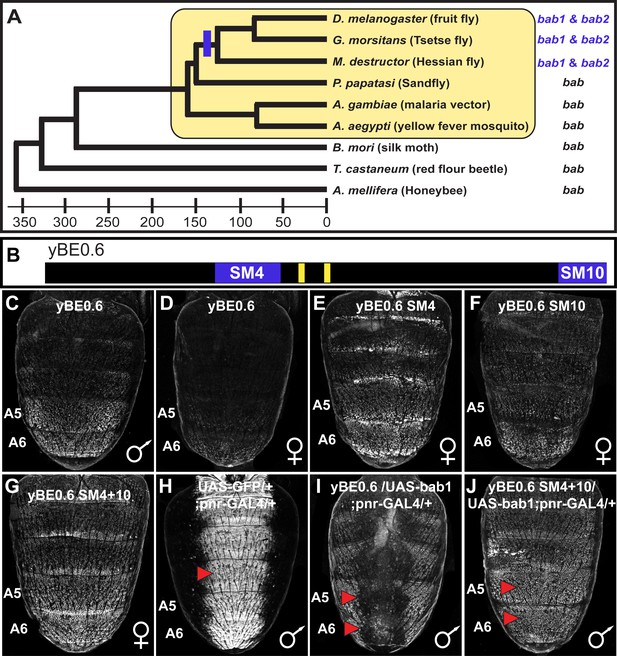
The tandemly duplicated bab genes perform a derived role in repressing a CRE controlling male-specific expression of the gene yellow.
(A) An ancestral bab gene was duplicated into the paralogous bab1 and bab2 genes in a Dipteran lineage that includes Drosophila fruit flies. The time scale indicates approximate divergence times in millions of years ago. (B) Male-specific expression of yellow in the abdominal epidermis is under the control of the yBE0.6 CRE that possesses two binding sites for Abd-B that are shown as yellow rectangles. Blue bars delimit the SM4 and SM10 regions required to suppress CRE activity in females. (C and D) The yBE0.6 EGFP reporter transgene is elevated in the male A5 and A6 abdomen segments (C) but is only barely detected females (D). (E–G) Ectopic reporter expression occurs in the female abdomen when either the SM4, SM10, or both regions are mutated. (H) The pnr-GAL4 driver activates dorsal midline expression of the UAS-EGFP gene, demarcating its domain of misexpression. (I) Dorsal midline expression of the yBE0.6 CRE is lost when bab1 is ectopically expressed by pnr-GAL4. (J) When the SM4 and SM10 regions are mutated, the yBE0.6 CRE can activate reporter expression in midline regions in spite of ectopically expressed bab1.
-
Figure 2—source data 1
Amino acid alignment for Bab homologs.
The amino acid sequences for Drosophila (D.) melanogaster Bab1 and Bab2, D. ananassae Bab1 and Bab2, D. willistoni Bab1, D. mojavensis Bab2, Glossina morsitans Bab1 and Bab2, and Anopheles gambiae Bab were aligned using the Clusta Omega multiple sequence alignment program. The BTB and Conserved Domains for D. melanogaster Bab1 and Bab2 are respectively indicated by the maroon and blue background colors. Within the Conserved Domain the psq domain is indicated by the amino acids with yellow font color and the AT-hooks by amino acids with red font color.
- https://doi.org/10.7554/eLife.32273.005
-
Figure 2—source data 2
Sequence alignment of the yBE0.6 with scanning mutant versions.
Purple background with white letters indicate the AscI (GGCGCGCC) and SbfI (CCTGCAGG) restriction enzymes sites that were appended to primers for cloning CRE versions into matching sites in the S3aG reporter transgene vector. Maroon background and white letters indicate sequences that comprise scanning mutations. The lower case nucleotide letters indicate the non-complementary transversions. The yellow background with black letters indicates the Abd-B sites identified in Jeong et al. (2006) which were not mutated in this study. The blue background with white letters indicate the Bab-bound sequences identified in this study. The gray background with bolded black letters indicate the regions to which the BE2.5 Fwd and BE3.5 Rvs primers (reverse complement of that highlighted) were designed to initially amplify the wild-type CRE sequence.
- https://doi.org/10.7554/eLife.32273.006
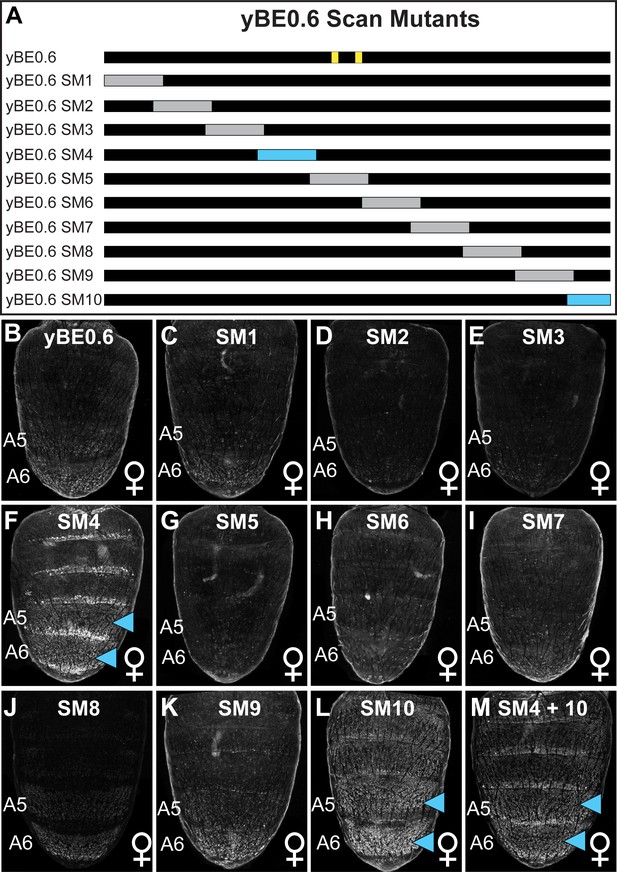
Scanning mutagenesis across the entire yBE0.6 CRE identifies sequences that normally function to repress CRE activity in the female abdomen.
(A) Name and location of yBE0.6 scanning mutations. Scanning mutations for which CRE activity in the female abdomen was not noticeably altered are indicated as gray rectangle and those for which ectopic activity occurred are shown as light blue rectangles. The two vertical yellow lines on the illustration of the wild type CRE indicate the position of previously identified Abd-B binding sites that were not mutated in this study. (B–M) The EGFP reporter gene expression pattern in the female abdomen at ~85 hr after puparium formation driven by the non-muntant (yBE0.6) and scan mutant CRE sequences. (M) Scanning mutations 4 and 10 were combined together. Light blue arrowheads indicate abdomen segments with conspicuous ectopic EGFP expression.
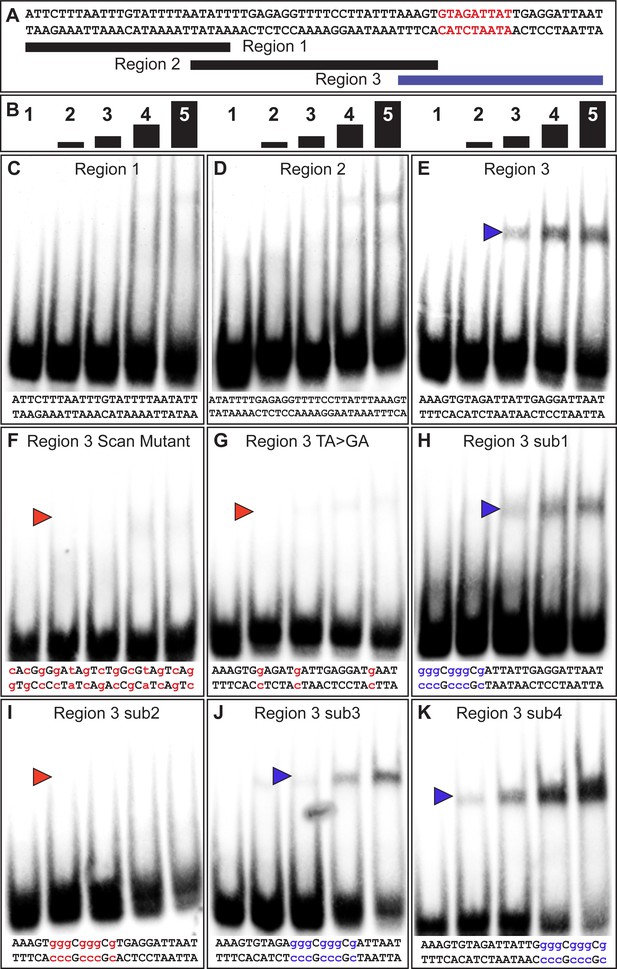
The yBE0.6 possesses a binding site for Bab in the SM4 region.
(A) The wild type DNA sequence of the SM4 region is shown, which was subdivided into three smaller regions annotated below that were used as double stranded probes in gel shift assays with the GST-Bab1 DNA-binding Domain (Bab1-DBD). Red text delimits the inferred Bab-binding site. (B) Each probe was tested in gel shift assay reactions for binding with five different amounts of Bab1-DBD. These were from left to right: 0, 500, 1000, 2000, and 4000 ng. (C–E) Gel shift assays using wild type probe sequences. (F–K) Gel shift assays using mutant probe sequences. Lower case blue letters indicate probe mutations that did not noticeably alter protein binding. Probe base pairs in lower case red letters are changes that altered protein binding. Blue and red arrowheads indicate the location of shifted probe, with red arrowheads indicating cases where the quantity of shifted probe was noticeably reduced.
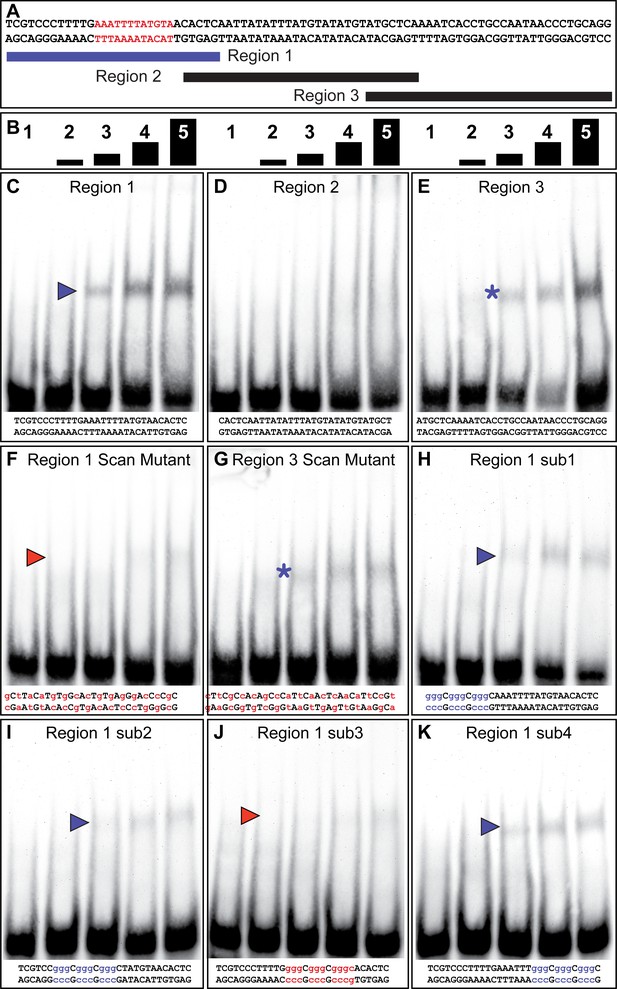
The yBE0.6 possesses a binding site for Bab in the SM10 region.
(A) The wild type DNA sequence of the SM10 region is shown, which was subdivided into three smaller regions annotated below that were used as double stranded probes in gel shift assays with the GST-Bab1 DNA-binding Domain (Bab1-DBD). Red text delimits the inferred Bab binding site. (B) Each probe was tested in gel shift assay reactions for binding with five different amounts of Bab1-DBD. These were from left to right: 0, 500, 1000, 2000, and 4000 ng. (C–E). Gel shift assays using wild type probe sequences. (F–K) Gel shift assays using mutant probe sequences. Lower case purple letters indicate probe mutations that did not noticeably alter protein binding. Probe base pairs in lower case red letters are changes that altered protein binding. Purple and red arrowheads indicate the location of shifted probe, with red arrowheads indicating cases where the quantity of shifted probe was noticeably reduced. Asterisks indicate a situation where binding was non-specific as both the wild type and mutant probes were bound by the Bab1-DBD.
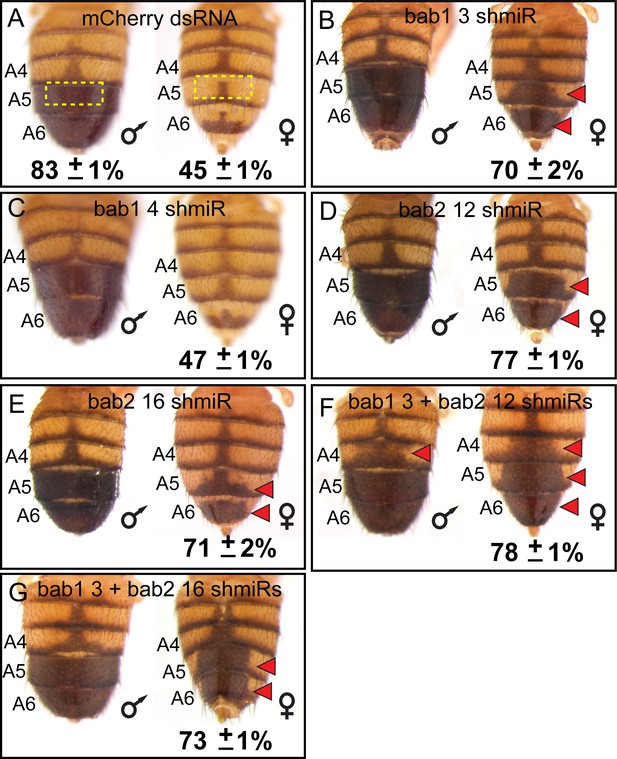
RNA-interference reveals a necessity for both bab1 and bab2 in suppressing female tergite pigmentation.
(A–G) Double-stranded (ds) RNA transgenes with UAS binding sites were expressed in the dorsal midline abdomen region driven by GAL4 that was expressed in the midline pattern of the pnr gene. (A) Expression of a negative control dsRNA that targets a gene (mCherry) that does not naturally exist in the D. melanogaster genome resulted in no apparent pigmentation phenotype from RNA-interference (RNAi). (B and C) Two different dsRNAs specific to bab1 and to (D and E) bab2 were tested for pigmentation phenotypes from RNAi. (F and G) Simultaneous RNAi for bab1 and bab2 was accomplished by expressing ‘chained’ transgenes. Red arrowheads indicate tergite regions where RNAi caused the development of ectopic pigmentation. The anterior midline tergite regions (illustrated in panel A by dashed yellow rectangles) were quantified for their darkness percentage for replicate specimens (n = 4). These percentages and their standard error of the mean (±SEM) are provided below a representative image.
-
Figure 5—source data 1
Analysis of RNA-interference pigmentation phenotypes.
- https://doi.org/10.7554/eLife.32273.010
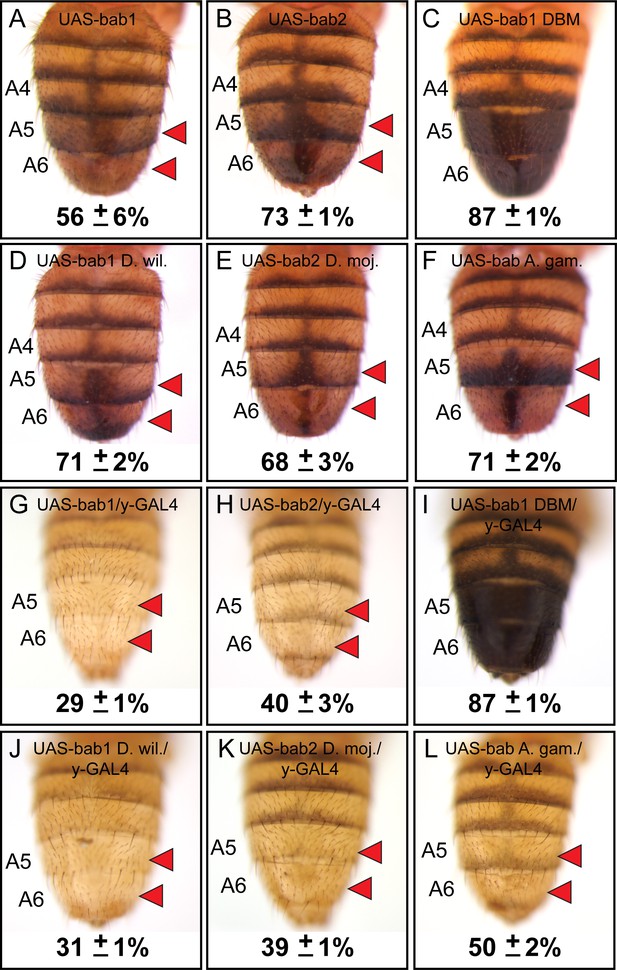
Bab1 and Bab2 are sufficient to suppress tergite pigmentation as DNA-binding transcription factors.
(A–L) Ectopic expression assays for various bab protein coding sequences. (A and G) D. melanogaster bab1, (B and H) D. melanogaster bab2, (C and I) a DNA-binding compromised version of D. melanogaster bab1 (bab1 DBM), (D and J) D. willistoni bab1, (E and K) D. mojavensis bab2, and (F and L) A. gambiae bab. (A–F) Leaky expression of transgenes from the attP40 transgene insertion site. (G–L) Ectopic expression of protein coding sequences in the male abdomen under the control of the y-GAL4 transgene. Red arrowheads indicate tergite regions with conspicuously reduced tergite pigmentation. The A5 and A6 tergite regions were quantified for their darkness percentage for replicate specimens (n = 4). These percentages and their standard error of the mean (±SEM) are provided below a representative image.
-
Figure 6—source data 1
The DNA and translated protein sequences for the bab open-reading frames.
- https://doi.org/10.7554/eLife.32273.018
-
Figure 6—source data 2
Analysis of pigmentation phenotypes from bab open-reading frame ectopic expression.
- https://doi.org/10.7554/eLife.32273.019
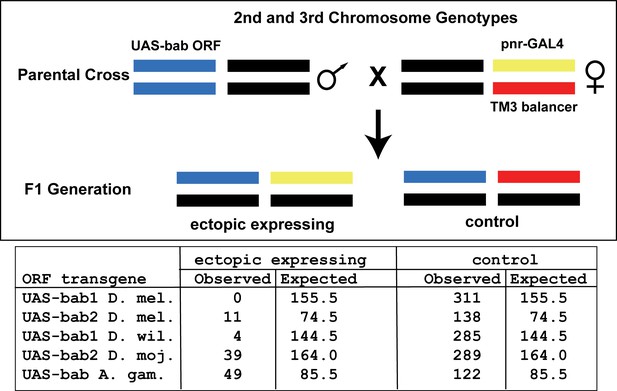
Lethality from ectopic expression of orthologous bab open reading frame transgenes in the pnr pattern.
UAS-bab open-reading frame transgenes are located in the attP40 site on the D. melanogaster second chromosome. Male flies were crossed to females heterozygous for the third chromosome where the GAL4 gene is inserted into the pnr locus and the TM3 balancer. Fewer offspring were obtained that possessed an ectopic bab expressing genotype than expected by chance, indicating lethality due to bab expression in the spatial and temporal pattern of the pnr gene.
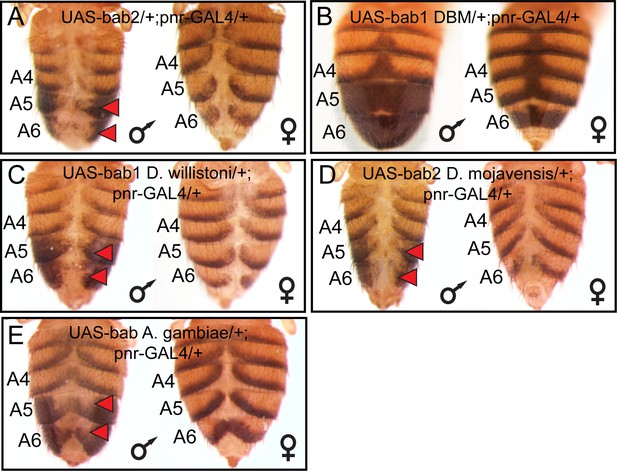
Bab proteins are sufficient to suppress tergite pigmentation when ectopically expressed in the dorsal midline of D. melanogaster.
(A–E) Ectopic expression of bab protein coding sequences in the dorsal midline of male and female abdomens under control of the pnr-GAL4 driver. These coding sequences were (A) D. melanogaster bab2, (B) a DNA-binding compromised version of D. melanogaster bab1 (bab1-DBM), (C) D. willistoni bab1, (D) D. mojavensis bab2, and (E) A. gambiae bab. Red arrowheads indicate tergite regions with conspicuously reduced tergite pigmentation. (A, C, D, and E) Ectopic expression resulted in reduced tergite pigmentation and a non-specific split tergite phenotype. (B) The DNA-binding mutant Bab1 could neither suppress tergite pigmentation nor cause the non-specific split tergite phenotype.
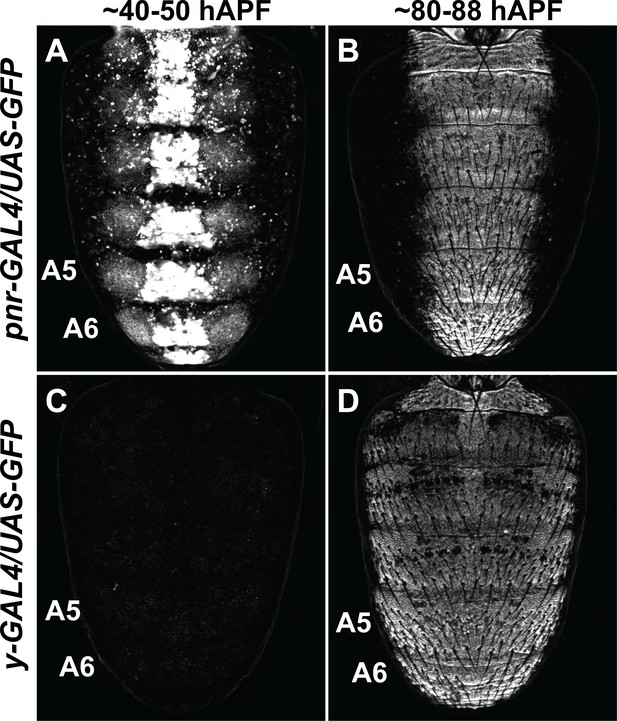
The temporal and spatial domains of activity for GAL4 drivers in D. melanogaster pupa.
(A) Dorsal midline expression of the UAS-GFP transgene under the control of the pnr-GAL4 driver at ~40–50 hr after puparium formation (hAPF). (B) Dorsal midline expression of the UAS-GFP transgene under the control of the pnr-GAL4 driver at ~80–88 hAPF. (C) The UAS-GFP transgene is not expressed at ~40–50 hAPF when under the regulatory control of the y-GAL4 driver. (D) The pan-abdomen expression of the UAS-GFP transgene under the control of the y-GAL4 driver at ~80–88 hAPF. The highest level expression occurs in the A5 and A6 segments due to the activity of the body element CRE which is included in the y-GAL4 transgene. All specimens shown are males.
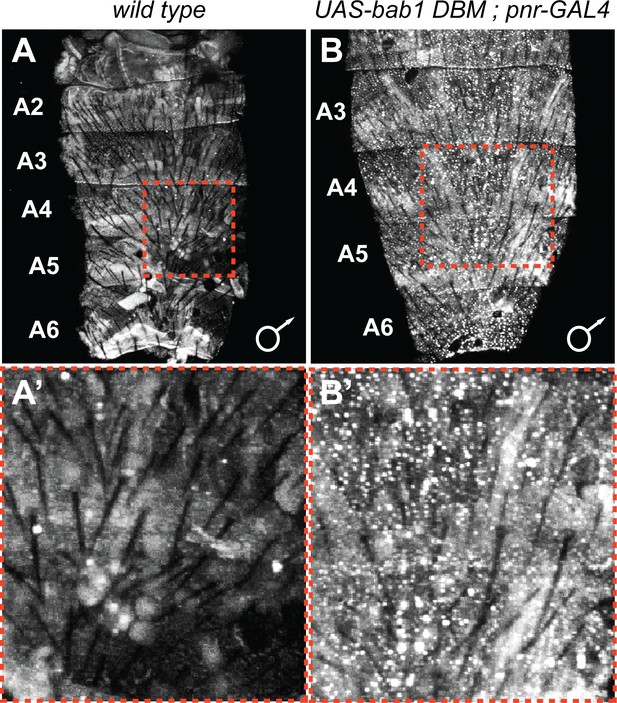
Ectopic expression of the Bab1-DNA-binding mutant protein.
(A) Little-to-no endogenous Bab1 protein can be detected in the dorsal abdominal epidermis of D. melanogaster male pupa. (B) In contrast, nuclear-localized expression of the Bab1 DNA-binding mutant protein can be observed when the UAS-transgene was ectopically expressed under the control of the pnr-GAL4 driver. (A’ and B’) Zoomed in view of the expression within the regions outlined by dashed red boxes in panels A and B. Samples shown are at a pupal developmental stage of ~88 hr after puparium formation.
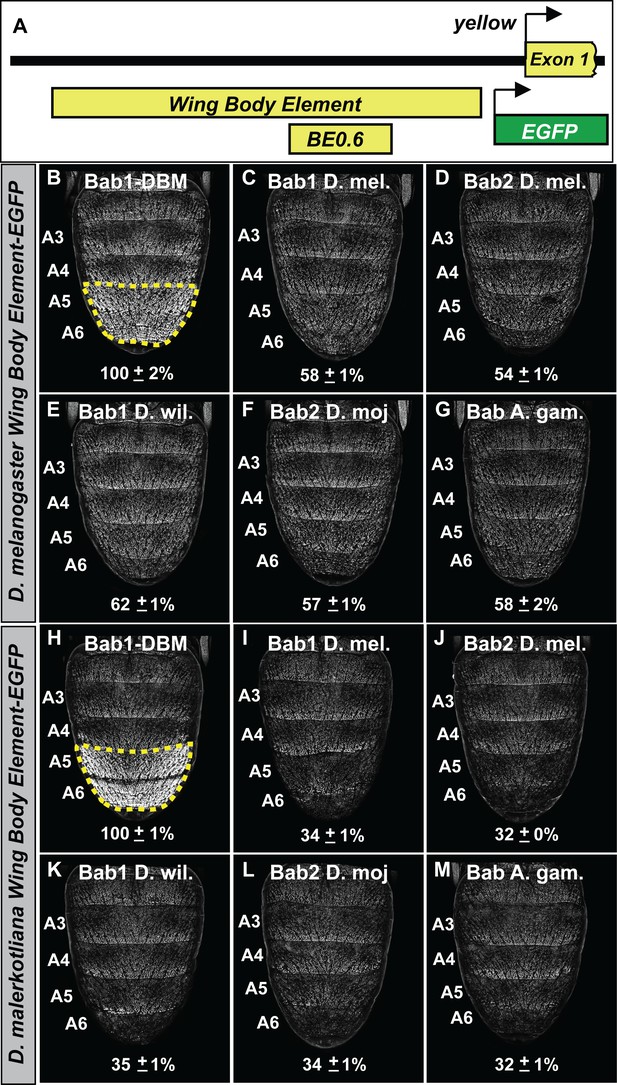
The Bab paralogs can suppress the male-specific activity of the regulatory region containing the wing element and body element CREs.
(A) 5’ of yellow exon one resides the wing element and body element CREs, and the position of the D. melanogaster yBE0.6 is shown below the to-scale representation of the partial locus. (B–G) Comparison of the levels of EGFP-reporter expression in the male A5 and A6 segments driven by the Wing Body Element of D. melanogaster. (H–M) Comparison of the levels of EGFP-reporter expression in the male A5 and A6 segments driven by the Wing Body Element of D. malerkotliana. The levels of EGFP expression are represented as the % of the mean ±SEM for samples in which the Bab1-DBM was expressed. (B and H) Robust EGFP reporter expression in samples ectopically expressing the Bab1-DBM protein in the y-GAL4 pattern. (C and I) Ectopic expression of Bab1 in the y-GAL4 pattern reduced A5 and A6 expression compared to the control. (D and J) Ectopic expression of Bab2 in the y-GAL4 pattern reduced A5 and A6 expression compared to the control. (E and K) Ectopic expression of D. willistoni Bab1 in the y-GAL4 pattern reduced A5 and A6 expression compared to the control. (F and L) Ectopic expression of D. mojavensis Bab2 in the y-GAL4 pattern reduced A5 and A6 expression compared to the control. (G and M) Ectopic expression of A. gambiae Bab in the y-GAL4 pattern reduced A5 and A6 expression compared to the control.
-
Figure 7—source data 1
Analysis of ectopic expression effects of bab open-reading frames on the dimorphic activities of yellow gene CREs.
- https://doi.org/10.7554/eLife.32273.021
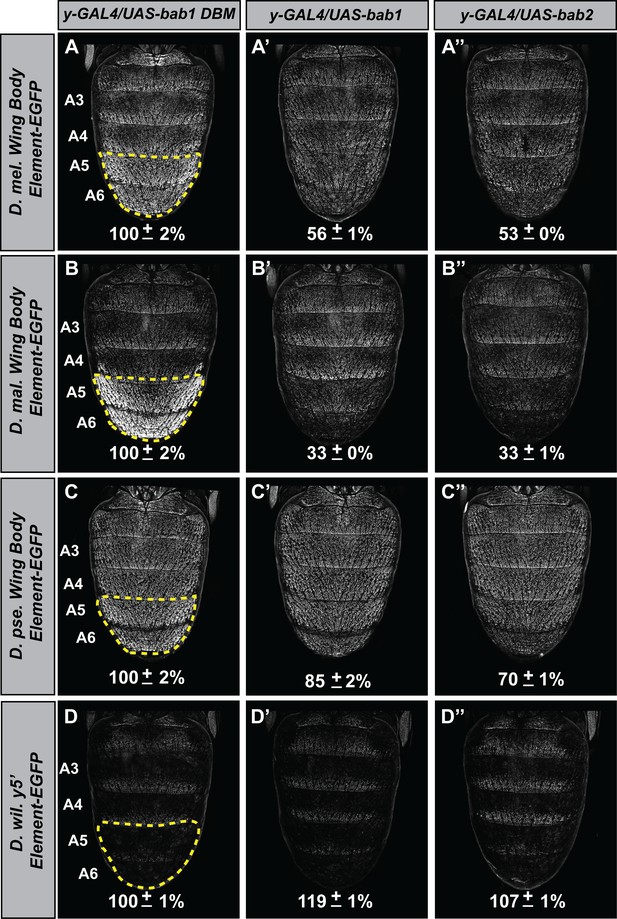
The evolved repression by Bab for cis-regulatory regions 5’ of yellow.
(A–A’’) Comparison of the levels of EGFP-reporter expression in the male A5 and A6 segments driven by the Wing Body Element of D. melanogaster. (B–B’’) Comparison of the levels of EGFP-reporter expression in the male A5 and A6 segments driven by the Wing Body Element of D. malerkotliana. (C–C’’) Comparison of the levels of EGFP-reporter expression in the male A5 and A6 segments driven by the Wing Body Element of D. pseudoobscura. (D–D’’) Comparison of the levels of EGFP-reporter expression in the male A5 and A6 segments driven by the 5’ non-coding region of D. willistoni yellow. For each comparison, the level of EGFP expression are expressed as the percentage of the mean ±SEM for samples in which the Bab1-DBM was expressed. (A–D) Ectopic expression of the Bab1-DBM in the y-GAL4 pattern. (A’–D’) Ectopic expression of Bab1 in the y-GAL4 pattern. (A’’–D’’) Ectopic expression of Bab2 in the y-GAL4 pattern.
-
Figure 8—source data 1
Analysis of the ectopic expression effects of bab open reading frames on yellow gene CREs from species with dimorphic and monomorphic tergite pigmentation.
- https://doi.org/10.7554/eLife.32273.024
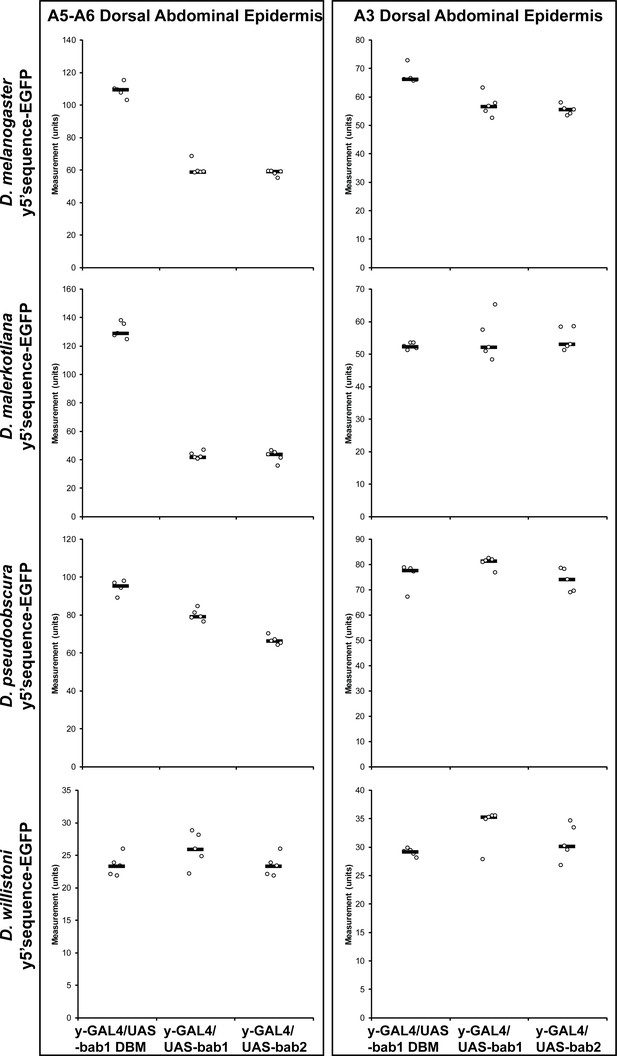
Orthologous regulatory regions 5’ of the yellow gene differ in their responsiveness to bab.
Scatter plots of the pixel intensity statistics obtained for the EGFP reporter expression occurring in the dorsal abdominal epidermis of the A5 and A6 segments and the A3 segment. For each condition (a reporter transgene with expression driven by a yellow CRE and an ectopically expressed bab open reading frame), expression was measured for five replicate male specimens, except for the D. pseudoobscura y5’ sequence in the presence of ectopic bab1 DBM expression (n = 4), for which the mean is shown as a horizontal black bar. All specimens used were at the developmental stage of ~85 hr after puparium formation for growth at 25°C.
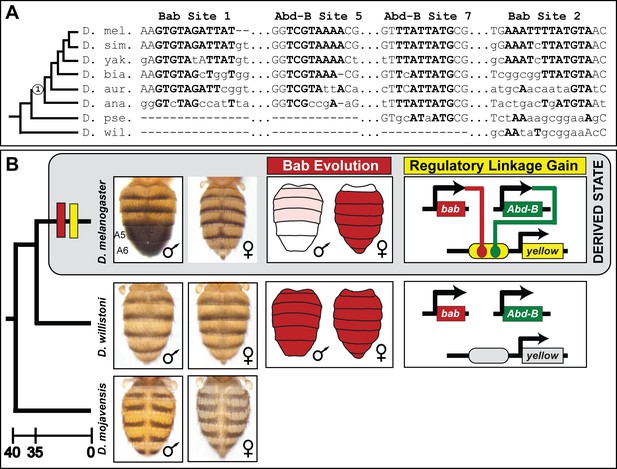
The evolution of male-specific pigmentation required the gain of a regulatory linkage between Bab and the newly evolved body element CRE controlling yellow expression.
(A) An alignment of the Bab-bound sequences in the SM4 (site 1) and SM10 (site 2) regions and for the two previously identified binding sites for Abd-B in the yBE0.6 CRE (Jeong et al., 2006). ‘Node 1’ on the phylogeny indicates the most recent common ancestor suspected to have possessed the derived male-specific pattern of pigmentation. Time scale shown is in millions of years ago. Bold capital letters indicate the bases bound by the transcription factor in the D. melanogaster CRE, and those which are conserved in the orthologous regions for related species. (B) Model for the derivation of a dimorphic pigmentation trait where dimorphic pigmentation required the evolution of a dimorphic Bab expression and the gain of a regulatory linkage between Bab and yellow through gains of binding sites in the body element CRE.
Tables
Design of small interfering RNA output for the bab1 ORF.
https://doi.org/10.7554/eLife.32273.011siRNA id | Position | SS sequence (Passenger) | AS sequence (Guide) | Corrected score |
---|---|---|---|---|
1 | 560 | GGAUAGCUGAGAUGUUGAAAG | UUCAACAUCUCAGCUAUCCUG | 99.7 |
2 | 1442 | CCGAUGACUUGGAGAUCAAGC | UUGAUCUCCAAGUCAUCGGCG | 85.7 |
3 | 278 | GGAACAACUAUCAGACGAACC | UUCGUCUGAUAGUUGUUCCAG | 97.6 |
4 | 162 | GAGUCAAGGUCAUGCUGUAGC | UACAGCAUGACCUUGACUCUC | 95.2 |
5 | 1483 | CGAGAGGAAGAAAGGGUAAGU | UUACCCUUUCUUCCUCUCGGA | 81.8 |
6 | 150 | CGAGGACAAGGAGAGUCAAGG | UUGACUCUCCUUGUCCUCGUC | 94.6 |
7 | 1473 | CGAGAUGAUCCGAGAGGAAGA | UUCCUCUCGGAUCAUCUCGGC | 78.9 |
8 | 219 | GGGCAGGAGUUCUUCGGUAGC | UACCGAAGAACUCCUGCCCUG | 89.5 |
9 | 359 | GCGAUGGUCGGUCCAUGAAGG | UUCAUGGACCGACCAUCGCAU | 88.2 |
10 | 664 | CCCAAGGAGAGCACUUCAACU | UUGAAGUGCUCUCCUUGGGCG | 84.7 |
11 | 368 | GGUCCAUGAAGGCCCACAAGA | UUGUGGGCCUUCAUGGACCGA | 87.5 |
Design of small interfering RNA output for the bab2 ORF.
https://doi.org/10.7554/eLife.32273.012siRNA _id | Position | SS sequence (Passenger) | AS sequence (Guide) | Corrected score |
---|---|---|---|---|
6 | 16 | GAUUGUGGACUUUGAAAUAAA | UAUUUCAAAGUCCACAAUCUG | 98.1 |
12 | 279 | CGGAGCUGGUGAAGUCCAAGG | UUGGACUUCACCAGCUCCGUU | 94.5 |
20 | 51 | GCGAAAUCGAUCAGUUCGAGG | UCGAACUGAUCGAUUUCGCCG | 94.4 |
18 | 155 | AGAAAGUACUCACCCGAAAGG | UUUCGGGUGAGUACUUUCUGU | 93.6 |
19 | 202 | AAGUGAGGUGGUUGAUCAAAU | UUGAUCAACCACCUCACUUGG | 92.5 |
23 | 241 | CGUUGGAGAAGUCAAGUCACC | UGACUUGACUUCUCCAACGCU | 92.3 |
42 | 1 | GGACAUGACCAAACAGAUUGU | AAUCUGUUUGGUCAUGUCCAU | 91.7 |
43 | 14 | CAGAUUGUGGACUUUGAAAUA | UUUCAAAGUCCACAAUCUGUU | 91.6 |
45 | 63 | AGUUCGAGGCGAGUGACUACA | UAGUCACUCGCCUCGAACUGA | 91.4 |
40 | 154 | CAGAAAGUACUCACCCGAAAG | UUCGGGUGAGUACUUUCUGUU | 90.7 |
49 | 13 | ACAGAUUGUGGACUUUGAAAU | UUCAAAGUCCACAAUCUGUUU | 90.7 |
28 | 306 | CGAUGAACGACCAAGCUUUGA | AAAGCUUGGUCGUUCAUCGGA | 90.6 |
46 | 140 | CUAGAGGACCAGAACAGAAAG | UUCUGUUCUGGUCCUCUAGAU | 90.4 |
13 | 625 | GACCAAUGUCUUUGACGAACU | UUCGUCAAAGACAUUGGUCAG | 90.3 |
55 | 12 | AACAGAUUGUGGACUUUGAAA | UCAAAGUCCACAAUCUGUUUG | 90.3 |
38 | 297 | AGGCGAGUCCGAUGAACGACC | UCGUUCAUCGGACUCGCCUUG | 89.8 |
27 | 443 | CAGCCUCAACCAAAUCUUAAG | UAAGAUUUGGUUGAGGCUGUG | 89.6 |
10 | 822 | UGGUGGAGUUCAUGUACAAGG | UUGUACAUGAACUCCACCAGG | 88.9 |
4 | 1099 | GGACUUGAAUCAGCGACAAAG | UUGUCGCUGAUUCAAGUCCAA | 88.8 |
Reagent type (species) or resource | Designation | Source or reference | Identifiers |
---|---|---|---|
Gene (Drosophila melanogaster) | bab1 | FLYB:FBgn0004870 | |
Gene (D. melanogaster) | bab2 | FLYB:FBgn0025525 | |
Gene (D. melanogaster) | yellow | FLYB:FBgn0004034 | |
Genetic reagent (D. melanogaster) | pnr-Gal4 | Bloomington Drosophila Stock Center | BDSC:3039 |
Genetic reagent (D. melanogaster) | y-Gal4 | Bloomington Drosophila Stock Center | BDSC:44267; FLYB:FBst0044267; RRID:BDSC_44267 |
genetic reagent (D. melanogaster) | UAS-GFP-nls | Bloomington Drosophila Stock Center | BDSC:4776 |
Genetic reagent (D. melanogaster) | UAS-mCherry dsRNA | Bloomington Drosophila Stock Center | BDSC:35785 |
Genetic reagent (D. melanogaster) | UAS-bab1 ORF of D. melanogaster | this paper | |
Genetic reagent (D. melanogaster) | UAS-bab2 ORF of D. melanogaster | this paper | |
Genetic reagent (D. melanogaster) | UAS-bab1 DBM ORF of D. melanogaster | this paper | |
Genetic reagent (D. melanogaster) | UAS-bab1 ORF of D. willistoni | this paper | |
Genetic reagent (D. melanogaster) | UAS-bab2 ORF of D. mojavensis | this paper | |
Genetic reagent (D. melanogaster) | UAS-bab Anopheles gambiae | this paper | |
Genetic reagent (D. melanogaster) | UAS-bab1 siRNA id #3 | this paper | |
Genetic reagent (D. melanogaster) | UAS-bab1 siRNA id #4 | this paper | |
Genetic reagent (D. melanogaster) | UAS-bab2 siRNA id #12 | this paper | |
Genetic reagent (D. melanogaster) | UAS-bab2 siRNA id #16 | this paper | |
Genetic reagent (D. melanogaster) | UAS-bab1 siRNA id #3 + bab2 siRNA id#12 | this paper | |
Genetic reagent (D. melanogaster) | UAS-bab1 siRNA id #3 + bab2 siRNA id#16 | this paper | |
Genetic reagent (D. melanogaster) | yBE0.6-EGFP reporter | PMID:25835988 | |
Genetic reagent (D. melanogaster) | yBE0.6 SM4-EGFP reporter | PMID:25835988 | |
Genetic reagent (D. melanogaster) | yBE0.6 SM10-EGFP reporter | PMID:25835988 | |
Genetic reagent (D. melanogaster) | yBE0.6 SM4 + SM10 EGFP reporter | PMID:25835988 | |
Genetic reagent (D. melanogaster) | yWing + Body Element (D. melanogaster)-EGFP reporter | PMID:25835988 | |
Genetic reagent (D. melanogaster) | yWing + Body Element (D. malerkotliana)-EGFP reporter | PMID:25835988 | |
Genetic reagent (D. melanogaster) | yWing + Body Element (D. pseudoobscura)-EGFP reporter | PMID:25835988 | |
Genetic reagent (D. melanogaster) | y5'1 (D. willistoni)-EGFP reporter | PMID:25835988 | |
Antibody | anti-Bab1 (rabbit polyclonal) | PMID:18724934 | |
Antibody | Alexa 647-secondary | Invitrogen | A-21244 |
Recombinant DNA reagent | GST-Bab1 DNA Binding Domain (DBD) | this paper |
Oligonucleotides used to make Scan Mutant four region gel shift probes.
https://doi.org/10.7554/eLife.32273.026Probe | Sequence (5’ to 3’) | Oligo name |
---|---|---|
SM4 Region 1 | ATTCTTTAATTTGTATTTTAATATT | yBE 4i1 Top |
AATATTAAAATACAAATTAAAGAAT | yBE 4i1 Bottom | |
SM4 Region 2 | ATATTTTGAGAGGTTTTCCTTATTTAAAGT | yBE 4i2 Top |
ACTTTAAATAAGGAAAACCTCTCAAAATAT | yBE 4i2 Bottom | |
SM4 Region 3 | AAAGTGTAGATTATTGAGGATTAAT | yBE 4i3 Top |
ATTAATCCTCAATAATCTACACTTT | yBE 4i3 Bottom | |
SM4 Region 3 Scan Mutant | cAcGgGgAtAgTcTgGcGtAgTcAg | y4i3 T Scrm |
cTgAcTaCgCcAgAcTaTcCcCgTg | y4i3 B Scrm | |
Region 3 TA > GA | AAAGTGgAGATgATTGAGGATgAAT | yBE 4i3 TA > GA Top |
ATTcATCCTCAATcATCTcCACTTT | yBE 4i3 TA > GA Bottom | |
SM4 Region 3 sub1 | gggCgggCgATTATTGAGGATTAAT | y4i3 sub1 T |
ATTAATCCTCAATAATcGgggGccc | y4i3 sub1 B | |
SM4 Region 3 sub2 | AAAGTgggCgggCgTGAGGATTAAT | y4i3 sub2 T |
ATTAATCCTCAcGcccGcccACTTT | y4i3 sub2 B | |
SM4 Region 3 sub3 | AAAGTGTAGAgggCgggCgATTAAT | y4i3 sub3 T |
ATTAATcGcccGcccTCTACACTTT | y4i3 sub3 B | |
SM4 Region 3 sub4 | AAAGTGTAGATTATTGgggCgggCg | y4i3 sub4 T |
cGcccGcccCAATAATCTACACTTT | y4i3 sub4 B |
Oligonucleotides used to make Scan Mutant 10 region gel shift probes.
https://doi.org/10.7554/eLife.32273.027Probe | Sequence (5’ to 3’) | Oligo name |
---|---|---|
SM10 Region 1 | TCGTCCCTTTTGAAATTTTATGTAACACTC | yBE 10i1 Top |
GAGTGTTACATAAAATTTCAAAAGGGACGA | yBE 10i1 Bottom | |
SM10 Region 2 | CACTCAATTATATTTATGTATATGTATGCT | yBE 10i2 Top |
AGCATACATATACATAAATATAATTGAGTG | yBE 10i2 Bottom | |
SM10 Region 3 | ATGCTCAAAATCACCTGCCAATAACCCTGCAGG | yBE 10i3 Top |
CCTGCAGGGTTATTGGCAGGTGATTTTGAGCAT | yBE 10i3 Bottom | |
SM10 Region 1 Scan Mutant | gCtTaCaTgTgGcAcTgTgAgGgAcCcCgC | y10i1 T Scrm |
GcGgGgTcCcTcAcAgTgCcAcAtGtAaGc | y10i1 B Scrm | |
SM10 Region 3 Scan Mutant | cTtCgCcAcAgCcCaTtCaAcTcAaCaTtCcGt | y10i3 T Scrm |
aCgGaAtGtTgAgTtGaAtGgGcTgTgGcGaAg | y10i3 B Scrm | |
SM10 Region 1 sub1 | gggCgggCgggCAAATTTTATGTAACACTC | y10i1 sub1 T |
GAGTGTTACATAAAATTTGcccGcccGccc | y10i1 sub1 B | |
SM10 Region 1 sub2 | TCGTCCgggCgggCgggCTATGTAACACTC | y10i1 sub2 T |
GAGTGTTACATAGcccGcccGcccGGACGA | y10i1 sub2 B | |
SM10 Region 1 sub3 | TCGTCCCTTTTGgggCgggCgggCACACTC | y10i1 sub3 T |
GAGTGTGcccGcccGcccCAAAAGGGACGA | y10i1 sub3 B | |
SM10 Region 1 sub4 | TCGTCCCTTTTGAAATTTgggCgggCgggC | y10i1 sub4 T |
GcccGcccGcccAAATTTCAAAAGGGACGA | y10i1 sub4 B |
Oligonucleotides for cloning bab1 and bab2 shRNAs into NheI and EcoRI sites of pattB-NE3 vector.
https://doi.org/10.7554/eLife.32273.028siRNA name and sequence | Oligo name | Oligo sequence (5’ – 3’) |
---|---|---|
bab1 siRNA 3 TTCGTCTGATAGTTGTTCCAG | b1_3 Top b1_3 Bottom | ctagcagtCTGGAACAACAATCAGACGTAtagttatattcaagcataTTCGTCTGATAGTTGTTCCAGgcg aattcgcCTGGAACAACTATCAGACGAAtatgcttgaatataactaTACGTCTGATTGTTGTTCCAGactg |
bab1 siRNA 4 TACAGCATGACCTTGACTCTC | b1_4 Top b1_4 bottom | ctagcagtGAGAGTCAAGCTCATGCTGAAtagttatattcaagcataTACAGCATGACCTTGACTCTCgcg aattcgcGAGAGTCAAGGTCATGCTGTAtatgcttgaatataactaTTCAGCATGAGCTTGACTCTCactg |
bab2 siRNA 16 TATTTCAAAGTCCACAATCTG | b2_16 Top b2_16 Bottom | ctagcagtCAGATTGTGGTCTTTGAAAAAtagttatattcaagcataTATTTCAAAGTCCACAATCTGgcg aattcgcCAGATTGTGGACTTTGAAATAtatgcttgaatataactaTTTTTCAAAGACCACAATCTGactg |
bab2 siRNA 12 TTGGACTTCACCAGCTCCGTT | b2_12 Top b2_12 Bottom | ctagcagtAACGGAGCTGCTGAAGTCCTAtagttatattcaagcataTTGGACTTCACCAGCTCCGTTgcg aattcgcAACGGAGCTGGTGAAGTCCAAtatgcttgaatataactaTAGGACTTCAGCAGCTCCGTTactg |
bab2 siRNA 20 TCGAACTGATCGATTTCGCCG | b2_20 Top b2_20 Bottom | ctagcagtCGGCGAAATCCATCAGTTCCAtagttatattcaagcataTCGAACTGATCGATTTCGCCGgcg aattcgcCGGCGAAATCGATCAGTTCGAtatgcttgaatataactaTGGAACTGATGGATTTCGCCGactg |
Additional files
-
Transparent reporting form
- https://doi.org/10.7554/eLife.32273.029