Pollination: Solar flower power
Most species of flowering plant cannot produce seeds without help from animals, like insects and birds, who transfer pollen between the flowers of different plants – often in return for a reward, such as a drink of sugar-rich nectar. In order to forage efficiently, pollinators seek out flowers with traits that they associate with a higher chance of getting a reward.
Some floral traits like color, shape and scent are obvious to our human senses, and as early as the 18th century scientists had worked out that these signals attract insects (Sprengel, 1793). However, the majority of floral traits have been discovered just recently, using modern technology. Indeed, we now know that pollinators use many different traits to find and evaluate flowers including: CO2 emission (Goyret et al., 2008); ultraviolet-absorbing pigmentation (Sheehan et al., 2016); humidity surrounding the flower (von Arx et al., 2012); fluorescence (Thorp et al., 1975); nectar color (Johnson et al., 2006); and even floral temperature (Dyer et al., 2006; Whitney et al., 2008).
Bees, for example, can use heat detectors on their legs and antennae to tell the difference between two flowers that differ in temperature by just two degrees (Heran, 1952). Now, in eLife, Sean Rands and colleagues from the Universities of Bristol and Exeter – including Michael Harrap as first author – report that bumblebees can also detect temperature differences within a single flower (Harrap et al., 2017).
Thermal images of more than 100 species of flowering plant taken in sunlight revealed a wide range of temperature patterns, reminiscent of the diversity of multi-colored petals we see with our own eyes (Figure 1A). More than half of the tested species had flowers in which some parts of the petals were at least 2°C warmer than the rest. Based on these findings, the researchers hypothesized that pollinators could use these temperature patterns to decide which flowers to visit.
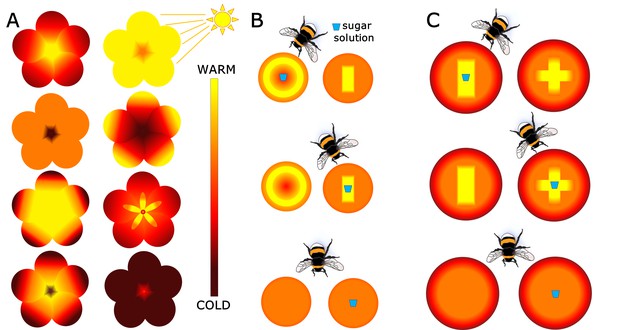
Floral temperature patterns and their role in bumblebee foraging.
(A) Schematic examples of the diversity of floral temperature patterns in the sunlight. By using thermal imaging, Harrap et al. show that more than half of the species they tested have temperature differences within flowers that are big enough to be detected by bumblebees (color indicates temperature as shown in gradient). Experiments with (B) small and (C) large artificial flowers tested if bumblebees could associate a certain temperature pattern with a reward, in this case a sugar solution (blue cup symbol). Bumblebees were presented with two variants of temperature patterns, one containing the sugar reward and the other just water (top and middle rows). As a control, flowers without temperature patterns were used as both rewarding and non-rewarding flowers (bottom row). The position of the bumblebee in B and C shows which floral temperature pattern was visited the most after 60 visits, demonstrating that bumblebees were able to use these patterns to increase their foraging success.
Harrap et al. tested if bumblebees (Bombus terrestris audax) could learn to associate a reward, in this case a drop of a sugary solution, with a certain temperature pattern. They presented two types of artificial flowers containing heating elements to naïve bumblebees. The artificial flowers in the first experiment had either a warm center or a warm periphery (Figure 1B), while those in the second had warmer centers in two different shapes (Figure 1C). In all experiments, one variant contained a drop of sugar, while the other just offered water. Flowers with disconnected heating elements were used as controls.
The bumblebees did recognize different temperature patterns and, in fewer than 20 visits, had learned to forage from those flowers that would give them the reward. Importantly, when there were no temperature patterns, as is in the controls, the bumblebees could not discriminate rewarding from non-rewarding flowers. Also, once the bumblebees had learned to associate a certain temperature pattern with a sugary reward, they continued to prefer this type of flower even when the reward was removed. These results indicate that the bumblebees were using the flower temperature patterns (and not other cues) to make an informed decision when foraging.
Why did plants evolve such incredibly complex and diverse floral traits? Plants often have to compete for pollinators, either with other plant species or with other members of their own species. Any trait that enables a flower to attract more pollinators than its competitors will give it an evolutionary advantage (in other words, more seeds or higher quality offspring). Of course, plants do not invent new traits with the intention of manipulating pollinators. Instead, small mutations occur in each generation and those that change floral traits offer a chance to bring the interaction between pollinator and flower closer to perfection. Hence, it is not surprising that so many floral traits are important and act together at the same time in a single plant species.
It is exciting that sunlight is needed to turn 'on' these temperature patterns and guide pollinators to flowers. Many other floral traits are hidden from our eyes and we have just started to unveil the ways in which pollinators are able to perceive and use floral signals. Combining 'old-fashioned' natural observations with new tools, such as thermal imaging cameras or 3D printers (Campos et al., 2015), allows us to unearth the wealth of strategies that pollinators and plants use to successfully interact with each other. Floral temperature patterns triggered by sunlight to signal to bumblebees are yet another example of an impressive feat of evolution.
References
-
Untersuchungen über den Temperatursinn der Honigbiene (Apis mellifica) unter besonderer Berücksichtigung der Wahrnehmung strahlender WärmeZeitschrift Für Vergleichende Physiologie 34:179–206.
-
BookDas entdeckte Geheimniss der Natur im Bau und in der Befruchtung der BlumenBerlin: Vieweg.
Article and author information
Author details
Publication history
- Version of Record published: December 19, 2017 (version 1)
Copyright
© 2017, Bing et al.
This article is distributed under the terms of the Creative Commons Attribution License, which permits unrestricted use and redistribution provided that the original author and source are credited.
Metrics
-
- 1,940
- views
-
- 184
- downloads
-
- 0
- citations
Views, downloads and citations are aggregated across all versions of this paper published by eLife.
Download links
Downloads (link to download the article as PDF)
Open citations (links to open the citations from this article in various online reference manager services)
Cite this article (links to download the citations from this article in formats compatible with various reference manager tools)
Further reading
-
- Ecology
Predator-prey arms races have led to the evolution of finely tuned disguise strategies. While the theoretical benefits of predator camouflage are well established, no study has yet been able to quantify its consequences for hunting success in natural conditions. We used high-resolution movement data to quantify how barn owls (Tyto alba) conceal their approach when using a sit-and-wait strategy. We hypothesized that hunting barn owls would modulate their landing force, potentially reducing noise levels in the vicinity of prey. Analysing 87,957 landings by 163 individuals equipped with GPS tags and accelerometers, we show that barn owls reduce their landing force as they approach their prey, and that landing force predicts the success of the following hunting attempt. Landing force also varied with the substrate, being lowest on man-made poles in field boundaries. The physical environment, therefore, affects the capacity for sound camouflage, providing an unexpected link between predator-prey interactions and land use. Finally, hunting strike forces in barn owls were the highest recorded in any bird, relative to body mass, highlighting the range of selective pressures that act on landings and the capacity of these predators to modulate their landing force. Overall, our results provide the first measurements of landing force in a wild setting, revealing a new form of motion-induced sound camouflage and its link to hunting success.
-
- Ecology
Diaphorina citri serves as the primary vector for ‘Candidatus Liberibacter asiaticus (CLas),’ the bacterium associated with the severe Asian form of huanglongbing. CLas-positive D. citri are more fecund than their CLas-negative counterparts and require extra energy expenditure. Therefore, understanding the molecular mechanisms linking metabolism and reproduction is of particular importance. In this study, we found adipokinetic hormone (DcAKH) and its receptor (DcAKHR) were essential for increasing lipid metabolism and fecundity in response to CLas infection in D. citri. Knockdown of DcAKH and DcAKHR not only resulted in the accumulation of triacylglycerol and a decline of glycogen, but also significantly decreased fecundity and CLas titer in ovaries. Combined in vivo and in vitro experiments showed that miR-34 suppresses DcAKHR expression by binding to its 3’ untranslated region, whilst overexpression of miR-34 resulted in a decline of DcAKHR expression and CLas titer in ovaries and caused defects that mimicked DcAKHR knockdown phenotypes. Additionally, knockdown of DcAKH and DcAKHR significantly reduced juvenile hormone (JH) titer and JH signaling pathway genes in fat bodies and ovaries, including the JH receptor, methoprene-tolerant (DcMet), and the transcription factor, Krüppel homolog 1 (DcKr-h1), that acts downstream of it, as well as the egg development related genes vitellogenin 1-like (DcVg-1-like), vitellogenin A1-like (DcVg-A1-like) and the vitellogenin receptor (DcVgR). As a result, CLas hijacks AKH/AKHR-miR-34-JH signaling to improve D. citri lipid metabolism and fecundity, while simultaneously increasing the replication of CLas, suggesting a mutualistic interaction between CLas and D. citri ovaries.