RETRACTED: Proteasome storage granules protect proteasomes from autophagic degradation upon carbon starvation
Figures
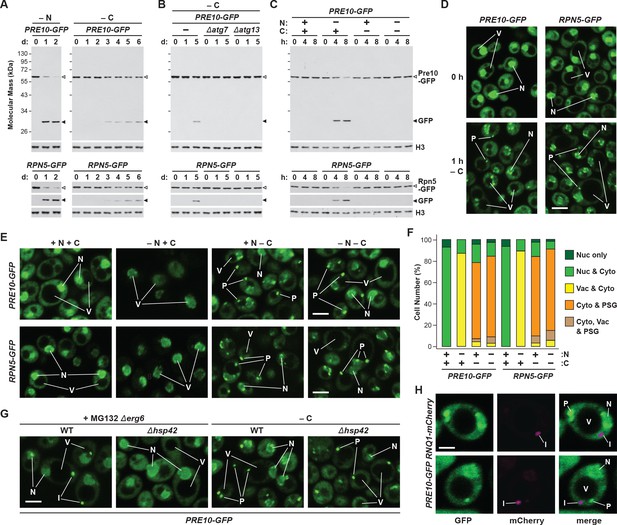
Proteasomes are rapidly degraded upon nitrogen but not carbon starvation.
(A, B and C) Measurement of proteaphagy upon nitrogen and/or carbon starvation by monitoring the release of free GFP from the CP and RP proteasome subunit reporters Pre10-GFP and Rpn5-GFP, respectively. Cells expressing PRE10-GFP or RPN5-GFP, and also containing the Δatg7 or Δatg13 mutations (panel B only), were switched from nutrient-rich medium (+N + C) to medium lacking either nitrogen (–N), carbon (–C), or both (–N –C). Total protein extracts from cells collected at the indicated times were assayed for GFP release by immunoblot analysis with anti-GFP antibodies. Open and closed arrowheads locate the GFP fusions and free GFP, respectively. The full gels are shown for the Pre10-GFP reporter, whereas only the regions of the gels containing the GFP fusion and free GFP are shown for the Rpn5-GFP reporter. Immunodetection of histone H3 was used to confirm near equal protein loading. (D) Proteasomes rapidly coalesce into PSG-type puncta soon after carbon starvation. PRE10-GFP or RPN5-GFP cells were examined by confocal fluorescence microscopy immediately before and 1 hr after switching from +N +C medium to –C medium. Scale bar, 2 µm. (E) Proteasomes are deposited into vacuoles upon nitrogen starvation, but form cytoplasmic PSG-type puncta in response to carbon starvation. PRE10-GFP or RPN5-GFP cells were grown on +N +C medium and then switched to +N +C, –N, –C, or –N –C media for 24 hr before imaging by confocal fluorescence microscopy. Scale bar, 2 µm. (F) Quantification of the cellular distribution of proteasomes when grown in +N +C, –N, –C, or –N –C media. Cells were treated and imaged as in panel (E). Each bar represents analysis of at least 200 cells. (G) Aggregation of proteasomes into IPODs, but not PSGs, requires the Hsp42 chaperone. PRE10-GFP cells with or without the Δerg6 and/or Δhsp42 mutations were switched from +N +C medium to either –C medium or +N +C medium containing 80 µM MG132 (+MG132) for 24 hr before imaging as in panel (E). Scale bar, 2 µm. (H) PSGs formed upon carbon starvation are distinct from IPOD puncta. PRE10-GFP cells also expressing the IPOD marker RNQ1-mCherry were switched from +N +C medium to –C medium for 24 hr before imaging as in panel E. Shown are the GFP, mCherry and merged fluorescence images. Scale bar, 1 µm. In panels D, E, G, and H: N, nucleus; V, vacuole; P, PSG; I, IPOD.
-
Figure 1—source data 1
Source data for Figure 1F.
- https://doi.org/10.7554/eLife.34532.006
-
Figure 1—source data 2
Source data for Figure 1—figure supplement 1A and B.
- https://doi.org/10.7554/eLife.34532.007
-
Figure 1—source data 3
Source data for Figure 1—figure supplement 2B.
- https://doi.org/10.7554/eLife.34532.008
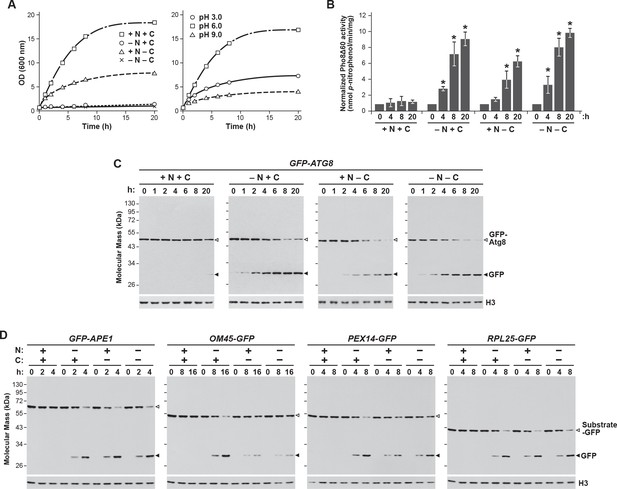
Carbon starvation activates both bulk and selective autophagy.
(A) Both carbon and nitrogen starvation, and growth at high or low pH, strongly attenuates yeast cell growth. Cells were grown in nutrient-rich (+N +C) medium at pH 6.0 and then switched to either medium lacking nitrogen (–N), carbon (–C), or both (–N –C; left panel), or to medium buffered to pH 3.0, 6.0 or 9.0 and containing 100 μM CCCP (right panel). Cell growth at the indicated times was monitored by measuring culture density at OD600. (B) Bulk autophagy is induced upon nitrogen and carbon starvation. Cells expressing Pho8Δ60 were grown for either 0, 4, 8 or 20 hr after a switch from +N +C medium to –N, –C or –N –C media. Cells were assayed for bulk autophagy using the phosphatase activity generated upon vacuolar activation of the Pho8Δ60 reporter. Values were normalized to those obtained at 0 hr. Each bar represents the mean (±SD) of three biological replicates, each comprised of three technical replicates. Asterisks indicate data points that are statistically significantly different to the 0 hr time point (p<0.05). (C) Both carbon and nitrogen starvation induce Atg8-mediated autophagy, as judged by release of free GFP from the GFP-Atg8 reporter. GFP-ATG8 cells were switched from +N +C medium to –N, –C or –N –C media. Total protein extracts from cells collected at the indicated times were assayed for GFP release by immunoblot analysis with anti-GFP antibodies. Open and closed arrowheads locate the GFP-Atg8 fusion and free GFP, respectively. Immunodetection of histone H3 antibodies was used to confirm near equal protein loading. (D) Both carbon and nitrogen starvation activate multiple selective autophagic routes. Cells expressing the GFP-Ape1 (CVT), Om45-GFP (mitophagy), Pex14-GFP (pexophagy), or Rpl25-GFP (ribophagy) reporters were switched from +N +C medium to –N, –C or –N –C media. Total protein extracts from cells collected at the indicated times were assayed for GFP release by immunoblot analysis with anti-GFP antibodies, as in panel (C). Open and closed arrowheads highlight the different GFP fusions and free GFP, respectively.
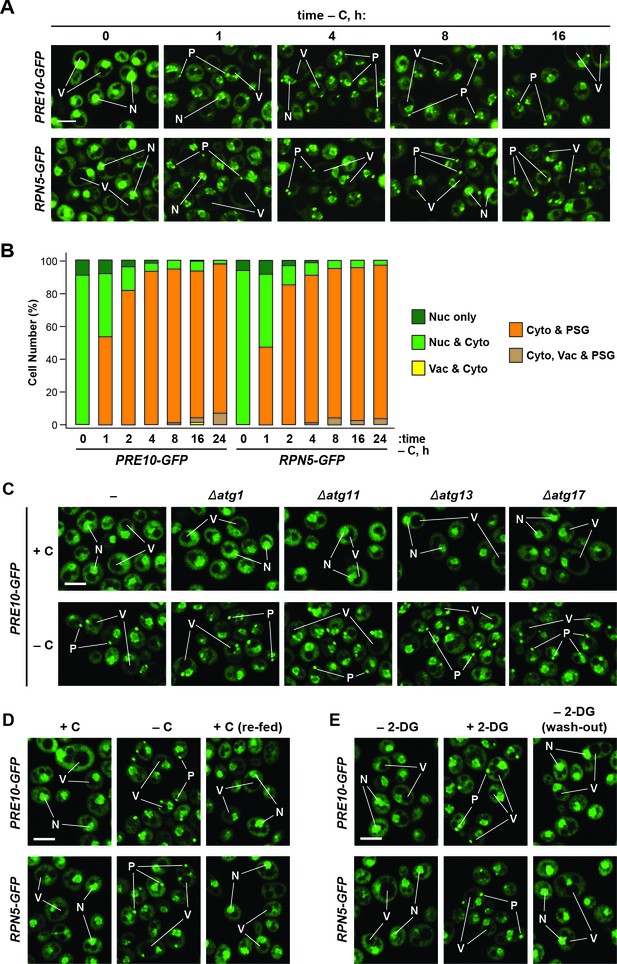
Formation of PSGs occurs rapidly in response to carbon starvation, is independent of the pre-autophagosomal structure (PAS), and is reversible.
(A) Proteasomes rapidly coalesce into PSG-type puncta soon after carbon starvation. PRE10-GFP or RPN5-GFP cells were grown on nutrient-rich (+N +C) medium and then switched to medium lacking carbon (–C) for the indicated periods of time before imaging by confocal fluorescence microscopy. Scale bar, 2 µm. (B) Time course for the changes in the cellular distribution of proteasomes when switched to growth in –C medium. The intracellular distribution of proteasomes was quantified from cells treated and imaged as in panel (A). Each bar represents analysis of at least 200 cells. (C) PSGs form upon carbon starvation even in mutants that cannot scaffold the PAS. PRE10-GFP cells with or without the Δatg1, Δatg11, Δatg13 or Δatg17 mutations were grown on nutrient-rich (+N +C) medium and then switched to –C medium for 6 hr before imaging as in panel (A). Scale bar, 2 µm. (D) PSG formation upon carbon starvation is rapidly reversible. PRE10-GFP cells were grown on +N +C medium, switched to –C medium for 6 hr, and then returned to +C medium for 30 min before imaging as in panel (A). Scale bar, 2 µm. (E) PSG formation upon treatment with 2-deoxyglucose (2-DG) is rapidly reversible. PRE10-GFP cells were grown on +N +C medium, switched to +C medium containing 5 mM 2-DG and 2 mM NaN3 medium for 6 hr, and then returned to +C medium lacking 2-DG and NaN3 for 1 hr before imaging as in panel (A). Scale bar, 2 µm. In panels A, C, D and E: N, nucleus; V, vacuole, P, PSG.
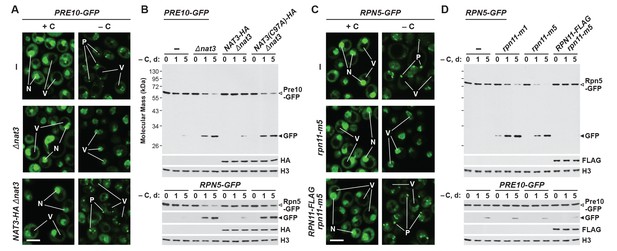
Mutants that block PSG formation accelerate proteaphagy upon carbon starvation.
(A) Elimination of the Nat3 subunit of the NatB N-acetylation complex promotes autophagic transport of proteasomes to the vacuole. PRE10-GFP cells containing the Δnat3 mutation with or without rescue with HA-tagged Nat3 were grown on nutrient-rich (+N +C) medium and then switched to –C medium for 24 hr before imaging by confocal fluorescence microscopy. Quantification is shown in Figure 2—figure supplement 1B. (B) Suppression of PSG assembly by deletion of Nat3 permits proteaphagy of the entire proteasome in response to carbon starvation. PRE10-GFP or RPN5-GFP cells containing the Δnat3 mutation, with or without rescue with HA-tagged Nat3 or the inactive Nat3(C97A) variant, were switched from +N +C medium to –C medium for the indicated times. Total protein extracts were assayed for GFP release by immunoblot analysis with anti-GFP antibodies as shown in Figure 1A. Open and closed arrowheads locate the GFP fusions and free GFP, respectively. Accumulation of the Nat3-HA and Nat3(C97A)-HA proteins was confirmed by immunoblotting with anti-HA antibodies. Immunodetection of histone H3 was used to confirm near equal protein loading. (C) The rpn11-m5 mutation blocks entry of the RP into PSGs and encourages transport of the RP to the vacuole in response to carbon starvation. RPN5-GFP cells containing the rpn11-m5 mutation with or without rescue with FLAG-tagged Rpn11 were switched from +N +C medium to –C medium for 24 hr before imaging by confocal fluorescence microscopy as in panel (A). Quantification in shown in Figure 2—figure supplement 1B. (D) Suppression of RP entry into PSGs by the rpn11-m1 and rpn11-m5 mutations promotes autophagic degradation of the RP but not the CP. PRE10-GFP or RPN5-GFP cells containing the rpn11-m1 or rpn11-m5 mutations with or without rescue with FLAG-tagged Rpn11 were switched from +N +C medium to –C medium for the indicated times and assayed for GFP release by immunoblotting as in panel (B). Accumulation of the Rpn11-FLAG protein was confirmed by immunoblotting with anti-FLAG antibodies. In panels A and C: N, nucleus; V, vacuole; P, PSG. Scale bar, 2 µm.
-
Figure 2—source data 1
Source data for Figure 2—figure supplement 1B.
- https://doi.org/10.7554/eLife.34532.011
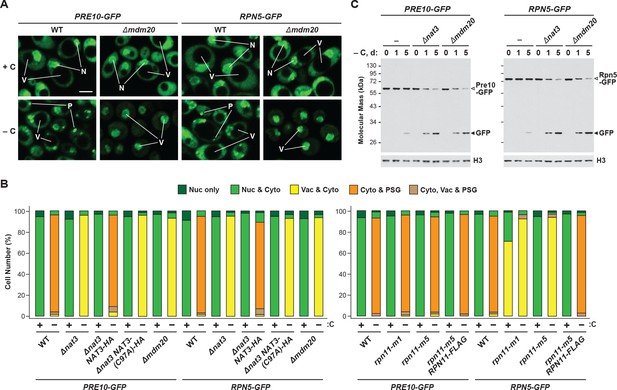
PSG formation requires Nat3, Mdm20, and the C-terminus of Rpn11.
(A) Elimination of the Mdm20 subunit of the NatB N-acetylation complex promotes autophagic transport of proteasomes to the vacuole. PRE10-GFP or RPN5-GFP cells with or without the Δmdm20 mutation were grown on nutrient-rich (+N +C) medium and then switched to –C medium for 24 hr before imaging by confocal fluorescence microscopy. Scale bar, 2 µm. (B) Quantification of the cellular distribution of proteasomes upon carbon starvation in the absence of Nat3, Mdm20, or the C-terminus of Rpn11. Cells were grown, treated and imaged as in panels A and C of Figure 2. Each bar represents analysis of at least 200 cells. (C) Suppression of PSG assembly by deletion of Mdm20 permits proteaphagy of the entire proteasome in response to carbon starvation. PRE10-GFP (left panel) or RPN5-GFP (right panel) cells with or without the Δnat3 or Δmdm20 mutations were switched from +N +C medium to –C medium for the indicated times. Total protein extracts were assayed for GFP release by immunoblot analysis with anti-GFP antibodies, as shown in Figure 1A. Open and closed arrowheads locate the GFP fusions and free GFP, respectively. Immunodetection of histone H3 was used to confirm near equal protein loading.
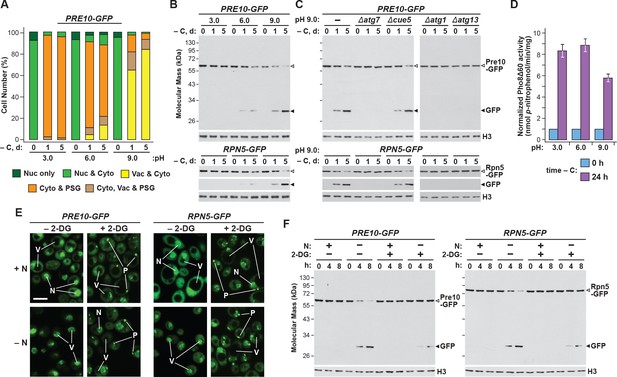
Growth conditions that impact PSG formation inversely affect autophagic clearance of proteasomes.
(A) Growth on high pH medium, which supresses PSG assembly, promotes proteaphagy in response to carbon starvation. PRE10-GFP or RPN5-GFP cells were switched from nutrient-rich (+N +C) medium buffered to pH 6.0 to the same medium buffered to pH 3.0, 6.0 or 9.0 and containing 100 µM CCCP for 1 hr, and then incubated for the indicated times in the same media lacking carbon. Shown is quantification of the cellular distribution of proteasomes following the indicated treatments. Each bar represents analysis of at least 200 cells. (B) Growth of yeast cells at high pH, but not low pH, accelerates proteaphagy. PRE10-GFP or RPN5-GFP cells were treated as in panel (A) and total protein extracts were assayed for GFP release by immunoblot analysis with anti-GFP antibodies as shown in Figure 1A. Open and closed arrowheads locate the GFP fusions and free GFP, respectively. Immunodetection of histone H3 was used to confirm near equal protein loading. (C) Accelerated proteaphagy at high pH is dependent on the core autophagy machinery, but not the autophagic receptor Cue5. PRE10-GFP or RPN5-GFP cells containing the Δatg1, Δatg7, Δatg13 or Δcue5 mutations were grown in pH 9.0 medium lacking carbon as in panel (A). Cell aliquots were collected at the indicated times and assayed for GFP release by immunoblotting as in panel (B). (D) Bulk autophagy is not appreciably impacted by the pH of the culture medium. Cells expressing PHO8Δ60 were switched from +N +C medium buffered to pH 6.0 to the same medium buffered to pH 3.0, 6.0 or 9.0 and containing 100 µM CCCP for 1 hr, and then further incubated for either 0 or 24 hr after a switch to the same media lacking carbon. Cells were assayed for bulk autophagy using the phosphatase activity generated upon vacuolar activation of the Pho8Δ60 reporter. Values were normalized to those obtained at 0 hr. Each bar represents the mean (±SD) of three independent biological replicates, each comprised of three technical replicates. (E) Exposing cells to 2-DG stimulates PSG formation. PRE10-GFP or RPN5-GFP cells grown in +N +C medium were pre-treated for 6 hr with or without 5 mM 2-deoxyglucose (2-DG) and 2 mM NaN3, and then switched to medium lacking nitrogen for 8 hr before imaging by confocal fluorescence microscopy. N, nucleus; V, vacuole; P, PSG. Scale bar, 2 µm. (F) Exposing nitrogen-starved cells to 2-DG protects proteasomes from autophagic degradation. PRE10-GFP or RPN5-GFP cells were pre-treated with 2-DG for 6 hr and then starved of nitrogen for 8 hr as in panel (E). Cell aliquots were collected at the indicated times and total protein extracts were assayed for GFP release by immunoblotting as in panel (B).
-
Figure 3—source data 1
Source data for Figure 3A and D.
- https://doi.org/10.7554/eLife.34532.013
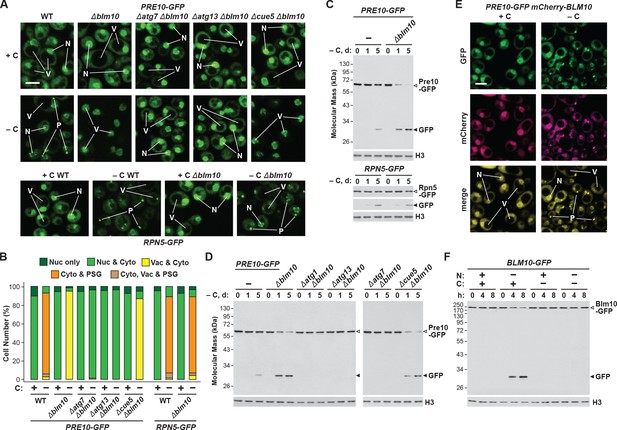
Blm10 encourages formation of CP-containing PSGs and suppresses autophagy of the CP in response to carbon starvation.
(A) Elimination of Blm10 suppresses formation of CP-containing PSGs and permits autophagic transport of the CP to the vacuole. PRE10-GFP or RPN5-GFP cells with or without the Δblm10 mutation, either alone or in combination with the Δatg7, Δatg13 or Δcue5 mutations, were grown on nutrient-rich (+N +C) medium and then switched to –C medium for 24 hr before imaging by confocal fluorescence microscopy. Scale bar, 2 µm. (B) Quantification of the cellular distribution of 26S proteasomes in response to carbon starvation in the absence of Blm10 and components of the autophagy machinery. Cells were grown, treated and imaged as in panel A. Each bar represents analysis of at least 200 cells. (C) Deletion of Blm10 accelerates proteaphagy of the CP, but not the RP, in response to carbon starvation. PRE10-GFP or RPN5-GFP cells with or without the Δblm10 mutation were switched from +N +C medium to –C medium for the indicated times. Total protein extracts were assayed for GFP release by immunoblot analysis with anti-GFP antibodies as shown in Figure 1A. Open and closed arrowheads locate the GFP fusion and free GFP, respectively. Immunodetection of histone H3 was used to confirm near equal protein loading. (D) Autophagic turnover of the CP in response to carbon starvation in the absence of Blm10 requires the core autophagy machinery, but not Cue5. PRE10-GFP cells with or without the Δblm10 mutation, either alone or in combination with the Δatg1, Δatg7, Δatg13 or Δcue5 mutations, were grown on +N +C medium and then switched to –C medium for the indicated times. Total protein extracts were assayed for GFP release by immunoblot analysis with anti-GFP antibodies as shown in panel (C). (E) Blm10 co-localizes with Pre10 into PSGs upon carbon starvation. PRE10-GFP cells also expressing mCherry-BLM10 were switched from +N +C medium to –C medium for 24 hr before imaging by confocal fluorescence microscopy. Shown are the GFP, mCherry, and merged fluorescence images. Scale bar, 2 µm. (F) Blm10 is targeted for autophagic degradation upon nitrogen starvation but not carbon starvation. BLM10-GFP cells were switched from +N +C medium to –N, –C, or –N –C media for the indicated times. Total protein extracts were assayed for GFP release by immunoblot analysis with anti-GFP antibodies as shown in panel (C). In panels A and E: N, nucleus; V, vacuole; P, PSG.
-
Figure 4—source data 1
Source data for Figure 4B.
- https://doi.org/10.7554/eLife.34532.016
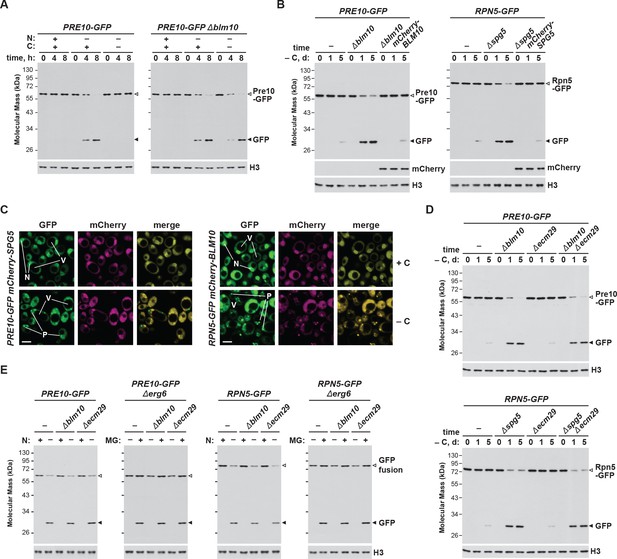
Functional copies of Blm10 and Spg5, but not Ecm29, are required to protect proteasomes from autophagic degradation upon carbon starvation.
(A) Blm10 is required to prevent proteaphagy of the CP upon simultaneous nitrogen and carbon starvation. PRE10-GFP cells with or without the Δblm10 deletion were switched from nutrient-rich (+N +C) medium to media lacking nitrogen (–N) or both nitrogen and carbon (–N –C). Total protein extracts from cells collected at the indicated times were assayed for GFP release by immunoblot analysis with anti-GFP antibodies. Open and closed arrowheads locate the Pre10-GFP fusion and free GFP, respectively. Immunodetection of histone H3 antibodies was used to confirm near equal protein loading. (B) Carbon-starvation-induced proteaphagy of the CP in the Δblm10 mutant, and of the RP in the Δspg5 mutant, can be rescued by expression of mCherry-tagged versions of Blm10 and Spg5, respectively. PRE10-GFP cells containing the Δblm10 deletion with or without expression of mCherry-BLM10 (left panel), or RPN5-GFP cells containing the Δspg5 deletion with or without expression of mCherry-SPG5 (right panel), were switched from +N +C medium to medium lacking carbon (–C). Total protein extracts from cells collected at the indicated times were assayed for GFP release by immunoblot analysis with anti-GFP antibodies, as in panel (A). Accumulation of the mCherry fusion proteins was confirmed by immunoblotting with anti-mCherry antibodies. (C) Blm10 co-localizes with Rpn5 in PSGs upon carbon starvation, but Spg5 does not co-localize with Pre10. Cells expressing PRE10-GFP and mCherry-Spg5, or RPN5-GFP and mCherry-BLM10, were switched from +N +C medium to –C medium for 24 hr before imaging by confocal fluorescence microscopy. Shown are the GFP, mCherry and merged fluorescence images. N, nucleus; V, vacuole; P, PSG. Scale bar, 2 µm. (D) Ecm29 is not required to either stimulate or prevent carbon starvation-induced proteaphagy. PRE10-GFP or RPN5-GFP cells with or without the Δblm10, Δspg5 and/or Δecm29 deletions were switched from +N +C medium to medium lacking carbon (–C), and cell aliquots were taken at the indicated periods of time. Total protein extracts were assayed for GFP release by immunoblot analysis with anti-GFP antibodies, as in panel (A). (E) Ecm29 is not required for nitrogen starvation- or inhibitor-induced proteaphagy. Cells expressing PRE10-GFP or RPN5-GFP with or without the Δerg6 mutation were switched from +N +C medium to medium lacking nitrogen (–N), or +N +C medium containing 80 μM MG132 (+MG132) and incubated for 8 hr. Total protein extracts were assayed for GFP release by immunoblot analysis with anti-GFP antibodies as in panel (A).
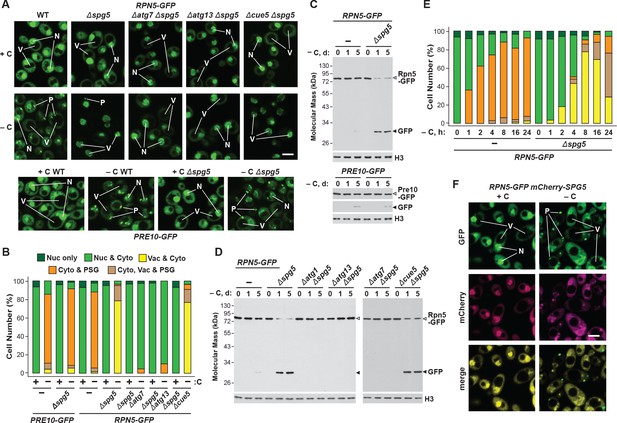
Spg5 encourages formation of RP-containing PSGs and suppresses autophagy of the RP in response to carbon starvation.
(A) Elimination of Spg5 suppresses formation of RP-containing PSGs and permits autophagic transport of the RP to the vacuole. PRE10-GFP or RPN5-GFP cells with or without the Δspg5 mutation, either alone or in combination with the Δatg7, Δatg13 or Δcue5 mutations, were grown on nutrient-rich (+N +C) medium and then switched to –C medium for 24 hr before imaging by confocal fluorescence microscopy. Scale bar, 2 µm. (B) Quantification of the cellular distribution of 26S proteasomes in response to carbon starvation in the absence of Spg5 and components of the autophagy machinery. Cells were grown, treated and imaged as in panel (A). Each bar represents analysis of at least 200 cells. (C) Deletion of Spg5 accelerates proteaphagy of the RP, but not the CP, in response to carbon starvation. PRE10-GFP or RPN5-GFP cells with or without the Δspg5 mutation were switched from +N +C medium to –C medium for the indicated times. Total protein extracts were assayed for GFP release by immunoblot analysis with anti-GFP antibodies, as shown in Figure 1A. Open and closed arrowheads locate the GFP fusion and free GFP, respectively. Immunodetection of histone H3 was used to confirm near equal protein loading. (D) Autophagic turnover of the RP in response to carbon starvation in the absence of Spg5 requires the core autophagy machinery, but not Cue5. RPN5-GFP cells with or without the Δspg5 mutation, either alone or in combination with the Δatg1, Δatg7, Δatg13 or Δcue5 mutations, were grown on +N +C medium and then switched to –C medium for the indicated times. Total protein extracts were assayed for GFP release by immunoblot analysis with anti-GFP antibodies as shown in panel (C). (E) Deletion of Spg5 delays, but does not completely block, formation of RP-containing PSGs in response to carbon starvation. RPN5-GFP cells with or without the Δspg5 mutation were switched from +N + C medium to –C medium for the indicated times before imaging by confocal fluorescence microscopy as in panel (A). The cellular distribution of GFP was quantified as in panel B; the color code for the bars is also included in this panel. Each bar represents analysis of at least 200 cells. (F) Spg5 does not routinely co-localize with Rpn5 into PSGs upon carbon starvation. RPN5-GFP cells also expressing mCherry-SPG5 were switched from +N +C medium to –C medium for 24 hr before imaging by confocal fluorescence microscopy. Shown are the GFP, mCherry, and merged fluorescence images. Scale bar, 2 µm. In panels A and F: N, nucleus; V, vacuole; P, PSG.
-
Figure 5—source data 1
Source data for Figure 5B and E.
- https://doi.org/10.7554/eLife.34532.019
-
Figure 5—source data 2
Source data for Figure 5—figure supplement 1.
- https://doi.org/10.7554/eLife.34532.020
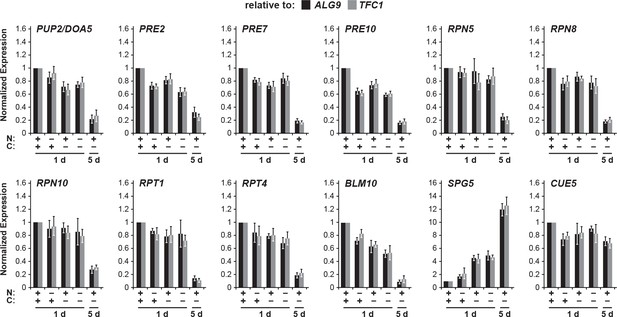
Long-term carbon starvation represses proteasome subunit gene expression but induces expression of SPG5.
Total RNA was extracted from cells following 1 or 5 days of starvation for nitrogen, carbon, or both nitrogen and carbon, and converted into first-strand cDNA. The relative transcript abundance of various proteasome subunit genes, including CP α- and β-subunits, RP base and lid subunits, the CP capping factor BLM10, the selective proteaphagy receptor CUE5, and the starvation-induced gene SPG5, was determined by quantitative real-time PCR, using the ALG9 and TFC1 genes as internal reference standards. All data points were normalized to non-starved cells. The bars represent the mean (±SD) from three biological replicates, each comprised of three technical replicates.
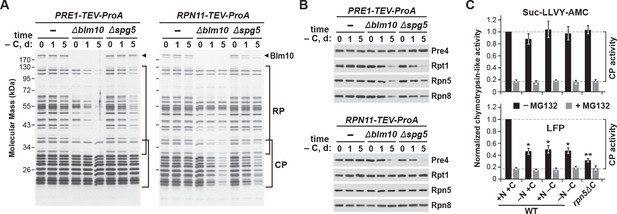
The CP and RP dissociate from each other upon carbon starvation and are separately delivered into PSGs.
(A and B) Yeast proteasomes selectively lose the RP or CP sub-complexes when purified from Δblm10 and Δspg5 cells via the CP or RP, respectively, upon growth on –C medium. PRE1-TEV-ProA or RPN11-TEV-ProA cells with or without the Δblm10 or Δspg5 mutations were switched from nutrient-rich (+N +C) medium to –C medium for the indicated times before affinity purification of proteasomes based on their ProA tags in the presence of ATP. The enriched proteasomes were subjected to SDS-PAGE followed by either staining for total protein with silver (panel A) or by immunoblotting with antibodies specific to subunits of the CP (Pre4) or RP (Rpt1, Rpn5 or Rpn8; panel B). In panel A, the distributions of the core CP and RP subunits are indicated by the brackets, and the position of Blm10 is indicated by the arrowheads. (C) Proteasome CPs remain active under conditions that promote PSG formation, but are less associated with the RP. Cells were grown on +N +C medium and then switched to media lacking either nitrogen (–N), carbon (–C), or both (–N –C) for 1 day. Total protein extracts were then assayed for CP peptidase activity using either Suc-LLVY-amc or Mca-AKVYPYPME-(Dpa)Dnp-amide (LFP) substrates that monitor total CP activity or RP-dependent CP activity, respectively. Black and grey bars represent the mean chymotrypsin-like activity (±SD) in the absence and presence of MG132, respectively, from three independent biological replicates, each comprised of three technical replicates.
-
Figure 6—source data 1
Source data for Figure 6C.
- https://doi.org/10.7554/eLife.34532.022
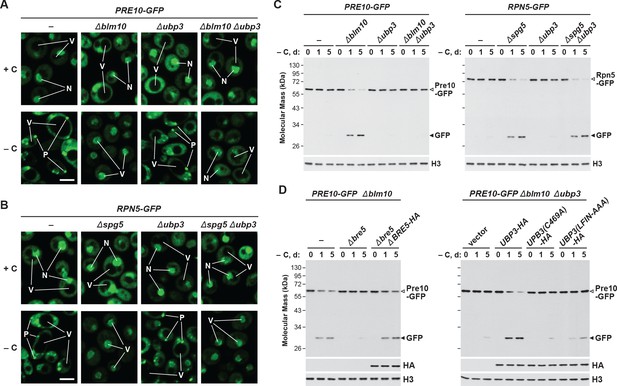
Carbon starvation-induced proteaphagy of the CP in the absence of Blm10 requires the deubiquitylating enzyme Ubp3.
(A and B) Elimination of Ubp3 suppresses transport of the CP (but not the RP) sub-complex to the vacuole in carbon-starved Δblm10 cells. Cells expressing PRE10-GFP (panel A) or RPN5-GFP (panel B) with or without the Δblm10, Δspg5 and/or Δubp3 mutations were grown on nutrient-rich (+N +C) medium and then switched to –C medium for 24 hr before imaging by confocal fluorescence microscopy. N, nucleus; V, vacuole; P, PSG. Scale bar, 2 µm. (C) Accelerated proteaphagy of the CP (but not the RP) in carbon-starved Δblm10 cells is blocked by deletion of Ubp3. PRE10-GFP or RPN5-GFP cells with or without the Δblm10, Δspg5, and/or Δubp3 mutations were switched from +N +C medium to –C medium for the indicated times. Total protein extracts were assayed for GFP release by immunoblot analysis with anti-GFP antibodies, as shown in Figure 1A. Open and closed arrowheads locate the GFP fusion and free GFP, respectively. Immunodetection of histone H3 was used to confirm near equal protein loading. (D) Autophagic degradation of the CP in Δblm10 cells starved for carbon requires active Ubp3 and its co-factor Bre5. PRE10-GFP Δblm10 cells containing the Δbre5 or Δubp3 mutations with or without rescue with HA-tagged Bre5, Ubp3, or mutated versions of Ubp3 lacking the active site cysteine (C469A) or the Bre5 binding site (LFIN-AAAA), were switched from +N +C medium to –C medium for the indicated times and assayed for GFP release by immunoblotting as in panel (C). Accumulation of the Bre5-HA, Ubp3-HA, Ubp3(C469A)-HA and Ubp3(LFIN-AAAA)-HA proteins was confirmed by immunoblotting with anti-HA antibodies.
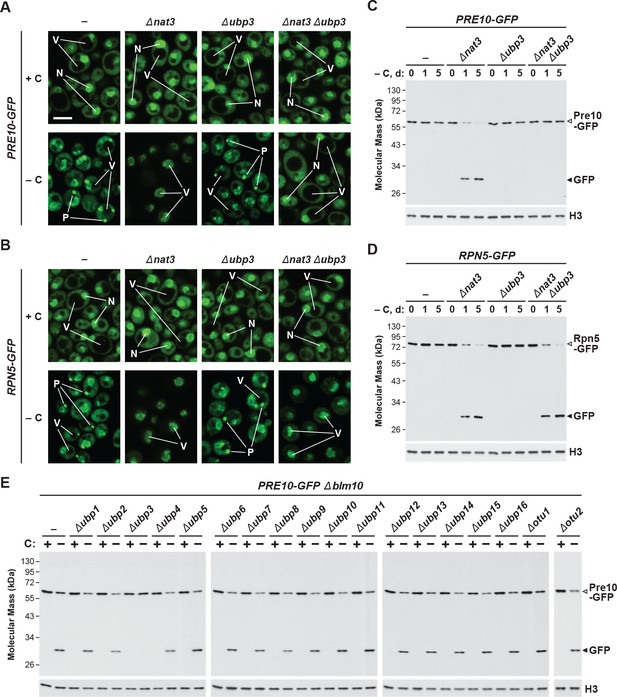
Carbon starvation-induced proteaphagy of the CP in the absence of Nat3 requires Ubp3, but deletion of other deubiquitylating enzymes (DUBs) does not impact carbon starvation-induced CP degradation.
(A and B) Elimination of Ubp3 suppresses transport of the CP (but not the RP) sub-complex to the vacuole in carbon-starved Δnat3 cells. Cells expressing PRE10-GFP (panel A) or RPN5-GFP (panel B) with or without the Δnat3 and/or Δubp3 mutations were grown on nutrient-rich (+N +C) medium and then switched to –C medium for 24 hr before imaging by confocal fluorescence microscopy. N, nucleus; V, vacuole; P, PSG. Scale bar, 2 µm. (C and D) Accelerated proteaphagy of the CP (but not the RP) in carbon-starved Δnat3 cells is blocked by deletion of Ubp3. PRE10-GFP (panel C) or RPN5-GFP (panel D) cells with or without the Δnat3 and/or Δubp3 mutations were switched from +N +C medium to –C medium for the indicated times. Total protein extracts were assayed for GFP release by immunoblot analysis with anti-GFP antibodies, as shown in Figure 1A. Open and closed arrowheads locate the GFP fusion and free GFP, respectively. Immunodetection of histone H3 was used to confirm near equal protein loading. (E) Only the Ubp3 DUB is required for carbon starvation-induced proteaphagy of the CP. PRE10-GFP cells containing the Δblm10 mutation together with the indicated DUB deletions were switched from nutrient-rich (+N +C) medium to medium lacking carbon (–C) and cell aliquots were taken after 0 or 24 hr. Total protein extracts were assayed for GFP release by immunoblot analysis, as in panel (C).
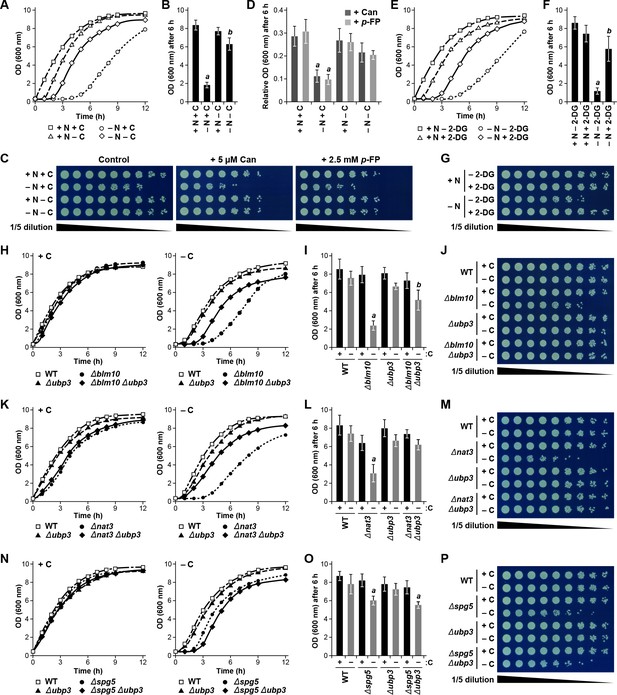
Protecting yeast proteasomes in PSGs upon starvation increases cell fitness.
(A) Delayed resumption of yeast cell growth following nitrogen starvation is reversed by simultaneous carbon starvation. Cells were grown in nutrient-rich (N + C) medium and then switched to either medium lacking nitrogen (–N), carbon (–C), or both (–N –C) for 24 hr. Near equal numbers of cells were then re-suspended in +N +C medium, and monitored for the resumption of cell growth by measuring culture density at OD600 over the next 12 hr. (B) Quantification of cell growth following nutrient starvation. Cells were grown as in panel (A), and cell growth was quantified by measuring culture density at OD600 6 hr after re-suspension in +N +C medium. (C) Reduced cell growth and increased susceptibility to amino acid analogs following nitrogen starvation is reversed by simultaneous carbon starvation. Cells were treated as in panel (A), and near equal numbers of cells were re-suspended in +N +C medium. Five-fold serial dilutions were then spotted onto synthetic complete medium with or without 5 μM canavanine (Can) or 25 mM p-fluorophenylalanine (p–FP) and incubated at 30°C for 36 hr. (D) Effects of amino acid anaolgs on cell growth following nutrient starvation. Cells were grown and treated as in panel (A), re-suspended in +N +C medium, and the resumption of cell growth in the presence or absence of 5 μM Can or 25 mM p-FP was monitored by measuring culture density at OD600 after 6 hr. The OD600 values in the presence of each analog were then normalized to those in the absence of the analogs. (E) Delayed resumption of cell growth following nitrogen starvation is reversed by pre-treatment with 2-DG. Cells were grown in +N +C medium with or without 5 mM 2-DG and 2 mM NaN3, and then switched to medium lacking nitrogen for 24 hr. Near equal numbers of cells were then re-suspended in +N +C medium, and the resumption of cell growth was monitored as in panel (A). (F) Quantification of cell growth during nitrogen starvation after a pre-treatment with 2-DG. Cells were grown and treated as in panel (E), and cell growth was quantified as in panel (B). (G) Reduced cell growth following nitrogen starvation is reversed by pre-treatment with 2-DG. Cells were treated as in panel (E), and near equal numbers of cells were re-suspended in +N +C medium. Five-fold serial dilutions were then spotted onto synthetic complete medium and incubated at 30°C for 36 hr. (H) Cells lacking BLM10 delay resumption of growth following carbon starvation, which is reversed by simultaneous deletion of UBP3. Cells were grown in +N +C medium and then switched to –C medium for 24 hr. Near equal numbers of cells were then re-suspended in +N +C medium, and the resumption of cell growth was monitored as in panel (A). Left panel, non-starved cells; right panel, carbon-starved cells. (I) Quantification of cell growth for strains lacking BLM10 and/or UBP3 following carbon starvation. Cells were grown and treated as in panel (H), and cell growth was quantified as in panel (B). (J) Reduced growth of Δblm10 cells following carbon starvation is reversed by deletion of UBP3. Cells were treated as in panel (H), and near equal numbers of cells were re-suspended, spotted onto synthetic complete medium and incubated as in panel (G). (K) Cells lacking NAT3 delay resumption of growth following carbon starvation, which is reversed by simultaneous deletion of UBP3. Cells were grown and treated as in panel (H), and the resumption of cell growth was monitored as in panel (A). Left panel, non-starved cells; right panel, carbon-starved cells. (L) Quantification of cell growth for strains lacking NAT3 and/or UBP3 following carbon starvation. Cells were grown and treated as in panel (H), and cell growth was quantified as in panel (B). (M) Reduced growth of Δnat3 cells following carbon starvation is reversed by deletion of UBP3. Cells were treated as in panel (H), and near equal numbers of cells were re-suspended, spotted onto synthetic complete medium and incubated as in panel (G). (N) Cells lacking SPG5 have slightly delayed resumption of growth following carbon starvation, but this resumption is not reversed by simultaneous deletion of UBP3. Cells were grown and treated as I panel (H), and the resumption of cell growth was monitored as in panel (A). Left panel, non-starved cells; right panel, carbon-starved cells. (O) Quantification of cell growth for strains lacking SPG5 and/or UBP3 following carbon starvation. Cells were grown and treated as in panel (H), and cell growth was quantified as in panel (B). (P) Reduced growth of Δspg5 cells following carbon starvation is not reversed by deletion of UBP3. Cells were treated as in panel (H), and near equal numbers of cells were re-suspended, spotted onto synthetic complete medium and incubated as in panel (G). Bars in panels B, D, F, I, L and O represent the mean (±SD) of three independent biological replicates. Letters represent data points that are statistically significantly different from the control (p<0.05).
-
Figure 8—source data 1
Source data for Figure 8A, B, D, E, F, H, I, K, L, N and O.
- https://doi.org/10.7554/eLife.34532.027
-
Figure 8—source data 2
Source data for Figure 8—figure supplement 1B, C, E, F, G, H, J, K, M and N.
- https://doi.org/10.7554/eLife.34532.028

Defects in cell fitness caused by failure to form PSGs in the Δblm10, Δspg5 and Δnat3 mutants can be rescued by expression of wild-type transgenes, or by blocking autophagy.
(A) A schematic illustrating the experimental design and time courses used for the yeast growth assays shown in Figure 8 and Figure 8—figure supplement 1. (B) The delayed resumption of Δblm10 cell growth following carbon starvation is rescued by expression of mCherry-BLM10. Cells were grown in nutrient-rich (+N +C) medium and then switched to medium containing (+C) or lacking (–C) carbon for 24 hr. Near equal numbers of cells were then re-suspended in +N +C medium, and the resumption of cell growth was monitored by measuring culture density at OD600 over the next 12 hr. (C) Quantification of cell growth for strains lacking BLM10 and/or expressing mCherry-BLM10 following carbon starvation. Cells were grown and treated as in panel (B), and cell growth was quantified by measuring culture density at OD6006 hr after resumption of growth in +C medium. (D) Reduced growth of Δblm10 cells following carbon starvation is reversed by expression of mCherry-BLM10. Cells were treated as in panel (B), and near equal numbers of cells were re-suspended in +C medium. Five-fold serial dilutions were then spotted onto synthetic complete medium and incubated at 30°C for 36 hr. (E) The growth of Δblm10 Δubp3 cells following carbon starvation is delayed by expression of wild-type UBP3-HA, but not of the catalytically inactive UBP3(C469A)-HA version or the UBP3(LFIN-AAAA)-HA variant defective in binding Bre5. Cells were grown, treated and monitored as in panel (B). Left panel, non-starved cells; right panel, carbon-starved cells. (F) Quantification of cell growth for strains lacking BLM10 and/or UBP3, with or without expression of wild-type UBP3-HA or the UBP3(C469A)-HA or UBP3(LFIN-AAAA)-HA variants, following carbon starvation. Cells were grown and treated as in panel (B), and cell growth was quantified as in panel (C). (G) The delayed resumption of Δspg5 cell growth following carbon starvation is rescued by expression of mCherry-SPG5. Cells were grown, treated and monitored as in panel (B). Left panel, non-starved cells; right panel, carbon-starved cells. (H) Quantification of cell growth for strains lacking SPG5 and/or expressing mCherry-SPG5 following carbon starvation. Cells were grown and treated as in panel (B), and cell growth was quantified as in panel (C). (I) Reduced growth of Δspg5 cells following carbon starvation is reversed by expression of mCherry-SPG5. Cells were treated as in panel (B), and near equal numbers of cells were re-suspended, spotted onto synthetic complete medium and incubated as in panel (D). (J) The delayed resumption of Δnat3 cell growth following carbon starvation is rescued by expression of NAT3-HA, but not the catalytically inactive NAT3(C97A)-HA variant. Cells were grown, treated and monitored as in panel (B). Left panel, non-starved cells; right panel, carbon-starved cells. (K) Quantification of cell growth for strains lacking NAT3, and/or expressing NAT3-HA or NAT3(C97A)-HA, following carbon starvation. Cells were grown and treated as in panel (B), and cell growth was quantified as in panel (C). (L) Reduced growth of Δnat3 cells following carbon starvation is reversed by expression of NAT3-HA, but not the catalytically inactive NAT3(C97A)-HA variant. Cells were treated as in panel (B), and near equal numbers of cells were re-suspended, spotted onto synthetic complete medium and incubated as in panel (D). (M) The delayed resumption of Δblm10 cell growth following carbon starvation is partially rescued by the Δatg7 mutant that eliminates autophagy. Cells were grown, treated and monitored as in panel (B). Left panel, non-starved cells; right panel, carbon-starved cells. (N) Quantification of yeast cell growth for strains lacking BLM10 and/or ATG7 following carbon starvation. Cells were grown and treated as in panel (B), and cell growth was quantified as in panel (C). (O) Reduced growth of Δblm10 cells following carbon starvation is reversed by disruption of autophagy. Cells were treated as in panel (B), and near equal numbers of cells were re-suspended, spotted onto synthetic complete medium and incubated as in panel (D). Bars in panels C, F, H, K, and N represent the mean (±SD) of three independent biological replicates. Letters represent data points that are statistically significantly different from the control (p<0.05).
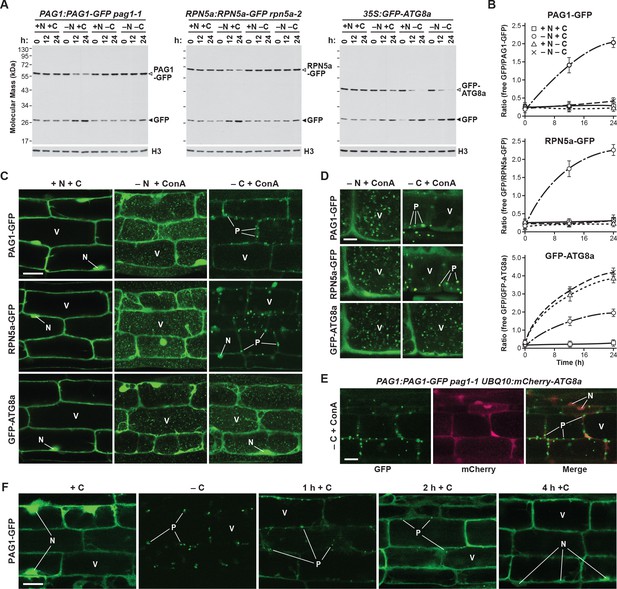
Fixed-carbon starvation selectively suppresses proteaphagy and promotes the formation of PSG-like structures in Arabidopsis.
(A) Measurement of proteaphagy upon nitrogen and/or fixed-carbon starvation in 5 day-old Arabidopsis seedlings by monitoring the release of free GFP from the CP and RP subunits PAG1-GFP or RPN5a-GFP, respectively. PAG1:PAG1-GFP pag1-1 and RPN5a:RPN5a-GFP rpn5a-2 seedlings were switched from growth in the light on nutrient-rich (+N +C) medium to either growth in the light on medium lacking nitrogen (–N), or growth in the dark on media lacking either carbon alone (–C) or both nitrogen and carbon (–N –C). Total protein extracts prepared from seedlings harvested at the indicated times were assayed for GFP release by immunoblot analysis with anti-GFP antibodies. Open and closed arrowheads locate the GFP fusion and free GFP, respectively. Immunodetection of histone H3 was used to confirm near equal protein loading. Rates of bulk autophagy were measured by the release of GFP from GFP-ATG8a in the same manner as above (right panel). (B) Quantification of the free GFP/GFP fusion ratios of the PAG1-GFP, RPN5a-GFP and GFP-ATG8a reporters upon switching from +N +C medium to –N, –C, or –N –C media. Levels of the GFP fusion and free GFP were determined by densitometric scans of the immunoblots shown in panel (A). Each data point represents the mean (±SD) of three independent biological replicates. (C) Proteasomes accumulate in autophagic bodies within the vacuole upon nitrogen starvation, but not fixed-carbon starvation. Five-day-old seedlings expressing PAG1-GFP, RPN5a-GFP or GFP-ATG8a were grown on +N +C medium and then switched to –N or –C media and treated with 1 µM ConA for 16 hr before imaging of the root lower elongation zone by confocal fluorescence microscopy. Scale bar, 10 µm. (D) Proteasomes assemble into large cytoplasmic PSG-like structures upon fixed-carbon starvation, instead of the smaller vacuolar puncta seen upon nitrogen starvation. Five-day-old seedlings expressing PAG1-GFP, RPN5a-GFP or GFP-ATG8a were grown, treated and imaged as in panel C, but focusing on cells closer to the root tip. Scale bar, 2 µm. (E) The PSG-like structures that form upon fixed-carbon starvation are not decorated with ATG8a. Roots from 5-day-old seedlings expressing PAG1-GFP and mCherry-ATG8a were grown, treated and imaged as in panel C. Shown are the GFP, mCherry and merged fluorescence channels. Scale bar, 5 µm. (F) The accumulation of PSG-like structures upon fixed-carbon starvation is rapidly reversible upon replenishment of the carbon source. Roots from 5 day-old seedlings expressing PAG1-GFP were grown on +N +C medium, switched to –C medium for 16 hr, and then returned to +N +C medium for the indicated times before imaging as in panel C. Scale bar, 10 µm. In panels C, D, E and F: N, nucleus; V, vacuole; P, PSG.
-
Figure 9—source data 1
Source data for Figure 9B.
- https://doi.org/10.7554/eLife.34532.030
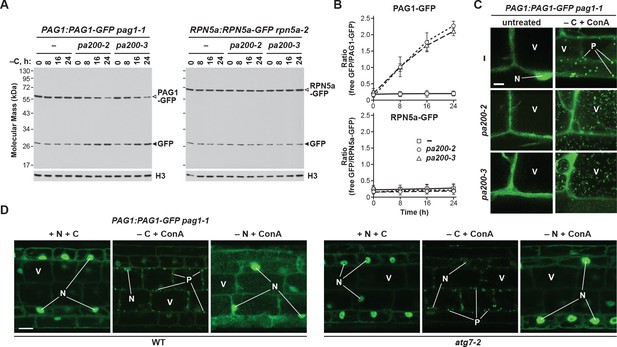
The formation of PSG-like structures in Arabidopsis upon fixed-carbon starvation requires the Blm10 ortholog PA200 and is independent of autophagy.
(A) Elimination of PA200 accelerates proteaphagy of the CP, but not the RP, in response to fixed-carbon starvation. PAG1:PAG1-GFP pag1-1 and RPN5a:RPN5a-GFP rpn5a-2 seedlings with or without the pa200-2 or pa200-3 mutations were switched from growth in the light on nutrient-rich (+N +C) medium to either growth in the light on medium lacking nitrogen (–N), or growth in the dark on media lacking either carbon alone (–C) or both nitrogen and carbon (–N –C). Total protein extracts prepared from seedlings harvested at the indicated times were assayed for GFP release by immunoblot analysis with anti-GFP antibodies. Open and closed arrowheads indicate the GFP fusion and free GFP, respectively. Immunodetection of histone H3 was used to confirm near equal protein loading. (B) Quantification of the free GFP/GFP fusion ratios of the PAG1-GFP and RPN5a-GFP reporters in wild-type (WT), pa200-2 or pa200-3 seedlings upon switching to –C medium. Levels of the GFP fusion and free GFP were determined by densitometric scans of the immunoblots shown in panel (A). Each data point represents the mean (±SD) of three independent biological replicates. (C) PAG1-GFP fails to coalesce into cytoplasmic PSG-like structures upon fixed-carbon starvation in the absence of PA200, and instead appears in vacuolar autophagic bodies. Five-day-old PAG1:PAG1-GFP pag1-1 seedlings with or without the pa200-2 or pa200-3 mutations were grown on +N +C medium and then transferred to –C medium containing 1 µM ConA and subjected to darkness for 16 hr. Cells were imaged by confocal fluorescence microscopy. Scale bar, 2 µm. (D) The cytoplasmic PSG-like structures containing PAG1-GFP form independently of autophagy. PAG1:PAG1-GFP pag1-1 seedlings with or without the atg7-2 mutation were grown on +N +C medium and then transferred to –N or –C media (in the light or dark, respectively) containing 1 µM ConA for 16 hr. Cells were imaged by confocal fluorescence microscopy as in panel (C). Scale bar, 10 µm. In panels C and D: N, nucleus; V, vacuole; P, PSG.
-
Figure 10—source data 1
Source data for Figure 10B.
- https://doi.org/10.7554/eLife.34532.033
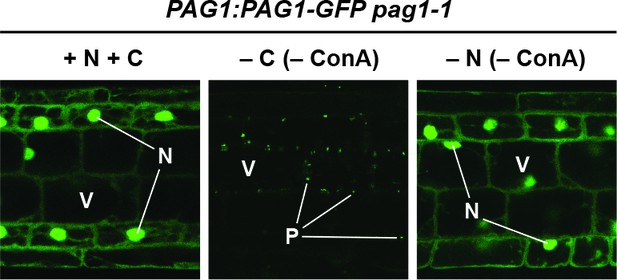
Cytosolic PSG-like structures form in Arabidopsis in the absence of concanamycin A (ConA) treatment.
Proteasomes accumulate in PSG-like foci upon fixed-carbon starvation even in the absence of treatment with ConA, which is required to stabilize autophagic bodies within the vacuole. Five-day-old PAG1:PAG1-GFP pag1-1 seedlings were switched from growth in the light on nutrient-rich (+N +C) medium to either growth in the light on medium lacking nitrogen (–N), or growth in the dark on media lacking carbon (–C) for 24 hr before imaging of the root lower elongation zone by confocal fluorescence microscopy. Whereas PSG-like foci are observed in the cytosol upon fixed-carbon starvation, vacuolar autophagic bodies are not observed upon nitrogen starvation due to their rapid degradation in the vacuole in the absence of ConA. Scale bar, 10 µm.
Tables
Reagent type or resource | Designation | Source or reference | Identifiers | Additional information |
---|---|---|---|---|
Strain (Saccharomyces cerevisiae) | Wild-type strains (BY4741; BY4742; SEY6210; SUB62; W303-1B) | Other | See additional information | Provided by Daniel Finley (Harvard Medical School), Audrey P. Gasch (University of Wisconsin) and Mark Hochstrasser (Yale University); allS. cerevisiae strains are listed in Supplementary file 1-Table S1 |
Strain (Arabidopsis thaliana) | Wild type ecotype Columbia-0 (Col-0) | Arabidopsis Biological Resource Center (ABRC) | CS60000 | N/A |
Genetic reagent (S. cerevisiae) | Yeast GFP clone collection strains (PRE10-GFP; RPN5-GFP; BLM10-GFP) | Thermo Fisher Scientific; PMID 14562095 | See additional information | All S. cerevisiae strains are listed in Supplementary file 1-Table S1 |
Genetic reagent (S. cerevisiae) | Yeast gene knockout collection strains (multiple) | GE Healthcare; PMID 10436161 | See additional information | All S. cerevisiae strains are listed in Supplementary file 1-Table S1 |
Genetic reagent (S. cerevisiae) | GFP-ATG8; GFP-APE1;OM45-GFP; PEX14-GFP;RPL25-GFP | PMID 15138258; PMID 25042851 | YTS187; KL095; KL099; KL282; KL285 | Provided by Stefan Jentsch (Max Planck Institut für Biochemie) and Daniel J. Klionsky (University of Michigan); all S. cerevisiae strains are listed in Supplementary file 1-Table S1 |
Genetic reagent (S. cerevisiae) | PHO8Δ60 | PMID 7741731 | TN124 | Provided by Daniel J. Klionsky (University of Michigan); all S. cerevisiae strains are listed in Supplementary file 1-Table S1 |
Genetic reagent (S. cerevisiae) | rpn11-m1; rpn11-m5 | PMID 18172023; PMID 19773362; PMID 23936414 | N/A | Provided by Agnès Delahodde (Université Paris-Sud); all S. cerevisiae strains are listed in Supplementary file 1-Table S1 |
Genetic reagent (S. cerevisiae) | PRE1-TEV-ProA; RPN11-TEV-ProA | PMID: 12408819 | SDL133; SDL135 | Provided by Daniel Finley (Harvard Medical School); allS. cerevisiae strains are listed in Supplementary file 1-Table S1 |
Genetic reagent (A. thaliana) | atg7-2 | GABI-Kat, Universität Bielefeld; PMID 20136727 | GABI_655_B06 | N/A |
Genetic reagent (A. thaliana) | pa200-2; pa200-3 | ABRC; PMID 20516081 | SALK_095870; SALK_070184 | N/A |
Genetic reagent (A. thaliana) | PAG1:PAG1-GFP pag1-1 | PMID 26004230 | SALK_114864 forpag1-1 | N/A |
Genetic reagent (A. thaliana) | RPN5a:RPN5a-GFP rpn5a-2 | PMID 26004230 | SALK_010840 forrpn5a-2 | N/A |
Genetic reagent (A. thaliana) | 35S:GFP-ATG8a | PMID 16040659 | N/A | N/A |
Genetic reagent (A. thaliana) | UBQ10:mCherry-ATG8a | PMID 21984698 | N/A | N/A |
Genes (A. thaliana and S. cerevisiae) | See additional information | Saccharomyces Genome Database or the Arabidopsis Information Resource | See additional information | All gene accession numbers are listed in Supplementary file 1-Table S2 |
Antibody | Anti-FLAG (mouse monoclonal) | Sigma-Aldrich | F3165, RRID:AB_259529 | 1:10,000 |
Antibody | Anti-GFP (mixture of mouse monoclonals) | Sigma-Aldrich | 11814460001, RRID:AB_390913 | 1:5000 |
Antibody | Anti-H3 (rabbit polyclonal) | Abcam | AB1791, RRID:AB_302613 | 1:3000 |
Antibody | Anti-HA (mouse monoclonal) | Covance | MMS-101R, RRID:AB_2314672 | 1:5000 |
Antibody | Anti-mCherry (mouse monoclonal) | Abcam | AB125096, RRID:AB_11133266 | 1:1000 |
Antibody | Anti-Pre4 (rabbit polyclonal) | Other | N/A | 1:1,000; provided by Daniel Finley (Harvard Medical School) |
Antibody | Anti-Rpn5 (rabbit polyclonal) | PMID 19252082 | N/A | 1:3000 |
Antibody | Anti-Rpn8 (rabbit polyclonal) | Other | N/A | 1:1,000; provided by Daniel Finley (Harvard Medical School) |
Antibody | Anti-Rpt1 (rabbit polyclonal) | Other | N/A | 1:1,000; provided by Daniel Finley (Harvard Medical School) |
Antibody | Goat anti-mouse HRP conjugate | Sercare | 074–1806, RRID:AB_2307348 | 1:10,000 |
Antibody | Goat anti-rabbit HRP conjugate | Sercare | 074–1506, RRID:AB_2721169 | 1:10,000 |
Recombinant DNA reagent | pAG424GPD-ccdB; pAG424GPD-ccdB-HA | Addgene; PMID 17583893 | 14152; 14248 | Provided by Susan Lindquist (Whitehead Institute for Biomedical Research) via Addgene |
Recombinant DNA reagent | RNQ1-mCherry | PMID 18756251 | pESC::GAL1- RNQ1-mCherry | Provided by Shay Ben-Aroya (Bar-Ilan University) |
Recombinant DNA reagent | mCherry-BLM10 | This paper | pAG424::GPD1- mCherry-BLM10 | The mCherry-BLM10 coding sequence (CDS) cloned into pAG424GPD-ccdB |
Recombinant DNA reagent | mCherry-SPG5 | This paper | pAG424::GPD1- mCherry-SPG5 | The mCherry-SPG5 CDS cloned into pAG424GPD-ccdB |
Recombinant DNA reagent | RPN11-FLAG | This paper | pAG424::GPD1- RPN11-FLAG | The RPN11-FLAG CDS cloned into pAG424GPD-ccdB |
Recombinant DNA reagent | BRE5-HA | This paper | pAG424::GPD1- BRE5-HA | The BRE5 CDS cloned into pAG424GPD-ccdB-HA |
Recombinant DNA reagent | NAT3-HA and derivatives | This paper | pAG424::GPD1-NAT3- HA and derivatives | The NAT3 CDS (and derivatives) cloned into pAG424GPD-ccdB-HA |
Recombinant DNA reagent | UBP3-HA and derivatives | This paper | pAG424::GPD1-UBP3- HA and derivatives | The UBP3 CDS (and derivatives) cloned into pAG424GPD-ccdB-HA |
Sequence-based reagent | See additional information | Integrated DNA Technologies | See additional information | All oligonucleotide primer sequences are listed in Supplementary file 1-Table S3 |
Recombinant protein | 6His-TEV protease | Other | N/A | Provided by E. Sethe Burgie (Washington University in St. Louis) |
Commercial assay or kit | LightCycler 480 SYBR Green I Master Mix | Roche Diagnostics | 04707516001 | N/A |
Commercial assay or kit | Pierce BCA protein assay kit | Thermo Fisher Scientific | 23225 | N/A |
Commercial assay or kit | SuperSignal West Pico Plus Chemiluminescent Substrate | Thermo Fisher Scientific | 34578 | N/A |
Chemical compound | 2-deoxyglucose | Sigma-Aldrich | D8375 | N/A |
Chemical compound | Canavanine sulphate salt | Sigma-Aldrich | C9758 | N/A |
Chemical compound | Carbonyl-cyanide-3- chlorophenylhydrazone | Sigma-Aldrich | C2759 | N/A |
Chemical compound | Concanamycin A | Santa Cruz Biotechnology | SC-202111A | N/A |
Chemical compound | LFP | GenScript; PMID 16337593 | See additional information | Custom synthesis |
Chemical compound | MG132 | Selleckchem | S2619 | N/A |
Chemical compound | N-succinyl-LLVY-7-amido- 4-methylcoumarin | Sigma-Aldrich | S6510 | N/A |
Chemical compound | p-fluorophenylalanine | Sigma-Aldrich | F5251 | N/A |
Chemical compound | p-nitrophenol | Sigma-Aldrich | 1048 | N/A |
Chemical compound | p-nitrophenyl phosphate disodium salt hexahydrate | Sigma-Aldrich | N4645 | N/A |
Software | Adobe Illustrator CC; Adobe Photoshop CC | Adobe Systems | N/A | N/A |
Software | Nikon Elements Imaging Software | Nikon | N/A | N/A |
Software | Total Lab Quant | Non-linear Dynamics | N/A | N/A |
Other | Immobilon-P PVDF Transfer Membrane | EMD Millipore | IPVH00010 | N/A |
Other | Murashige and Skoog basal salt micronutrient solution | Sigma-Aldrich | M0529 | N/A |
Other | Nickel-nitrilotriacetic acid-agarose beads | Qiagen | 30230 | N/A |
Other | Protease inhibitor cocktail | Sigma-Aldrich | P9599 | N/A |
Other | Rabbit whole molecule IgG antigen affinity gel | MP Biomedicals | 0855961 | N/A |
Other | Yeast nitrogen base without amino acids and ammonium sulphate | Sigma-Aldrich | Y1251 | N/A |
Additional files
-
Supplementary file 1
Table S1: Saccharomyces cerevisiae strains used in this study.
Table S2: Accession numbers of genes used in this study. Table S3: Oligonucleotide primers used in this study.
- https://doi.org/10.7554/eLife.34532.034
-
Transparent reporting form
- https://doi.org/10.7554/eLife.34532.035