Normal cognitive and social development require posterior cerebellar activity
Figures
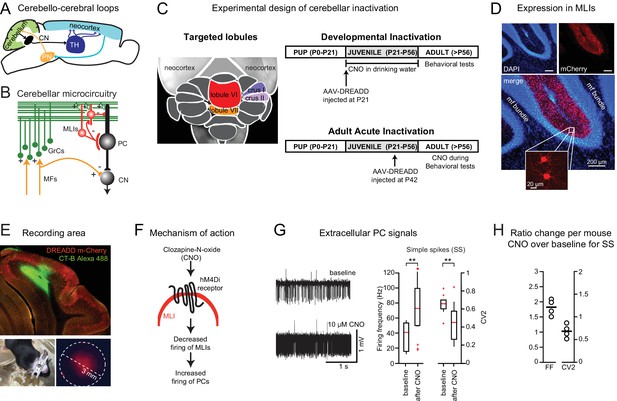
Chemogenetic perturbation of cerebellar activity.
(A) Cerebello-cerebral loops, defined by an ascending pathway via thalamus and a descending reciprocal pathway via pontine nuclei. (B) Simplified diagram of cerebellar microcircuitry. Molecular layer interneurons (MLIs) receive incoming excitation from the mossy fiber (MF)-granule cell (GrC) pathway and in turn inhibit Purkinje cells (PCs), the output neurons of the cerebellar cortex which then inhibit neurons of the cerebellar nuclei (CN). (C) Left, dorsal view of cerebellum with the four targeted lobules indicated in color. Right, experimental design for developmental and acute perturbation. (D) Expression of the chemogenetic DREADD probe hM4D(Gi)-mCherry in MLIs (red). Note the absence of mCherry signal in the granule cell layer or the mossy fiber bundle visualized by DAPI staining (blue) (see Video 1). (E) Top, a sagittal cerebellar section showing an example recording location in the in vivo awake experiment. The recording location was marked by cholera toxin subunit B conjugated to Alexa 488 staining (green); DREADD expression marked by mCherry (red). Bottom left, removable implant used for in vivo electrophysiology. Bottom right, mCherry expression imaged through the implant silicone plug. (F) The activating ligand clozapine-N-oxide (CNO) binds to the hM4Di receptor, which decreases firing of MLIs (see Figure 1—figure supplement 1) and thus removes synaptic inhibition from PCs. (G) Left, extracellular recording of PC activity from awake mice before and after CNO application. Right, CNO (10 μM) leads to an increase in the simple-spike firing frequency and a decrease in the local coefficient of variation (CV2). **, different from baseline by paired t-test, p<0.05 (H) CNO-to-baseline ratios of the measures, plotted on a cell-by-cell basis.
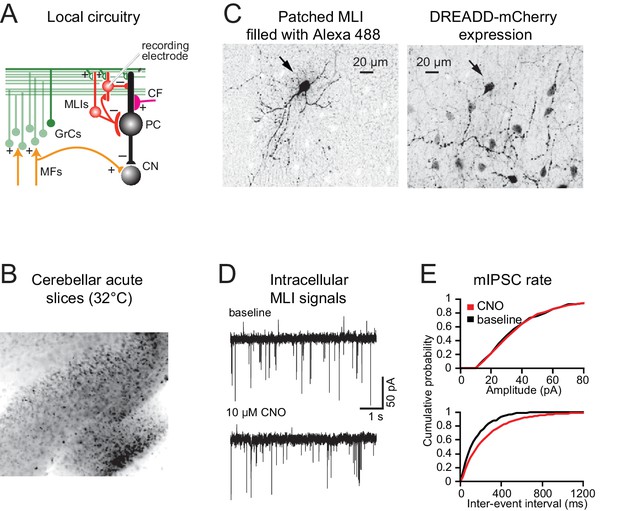
CNO administration alters cerebellar activity in vitro.
(A) Schematic diagram of cerebellar circuitry depicting the position of the recording electrode in the slice preparation. (B) Molecular layer interneurons (MLIs) expressing DREADDs (black) in a brain slice imaged under epifluorescence at 10x. Image is inverted in intensity. (C) Left, example of a patched MLI filled with Alexa 488 and imaged on a confocal microscope at 40x. Right, same cell showing DREADD-mCherry expression. Note the presence of many mCherry-positive MLIs. Image is inverted in intensity. (D) Whole-cell voltage-clamp patch recording of an MLI before and after CNO application. (E) CNO administration blocks MLI activity (n = 2 mice, 7 cells).
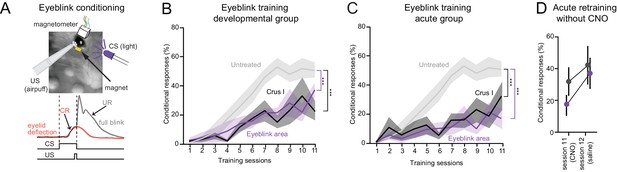
Juvenile-life perturbation disrupts the development of cerebellar-dependent eyeblink conditioning.
(A) Top, eyeblink conditioning performed using an unconditional stimulus (US, corneal airpuff) delivered at the end of a conditional stimulus (CS, LED). Bottom, learned anticipatory eyelid deflection (conditional response, CR; red), followed by an unconditional reflex (UR) blink. (B) Reduced frequency of CRs after developmental CNO activation of DREADDs in the eyeblink area of lobule VI (p<10−10, two-way ANOVA) and in crus I (p<10−6) compared to controls (see Figure 2—figure supplement 1). (C) Reduced frequency of conditional responses after acute CNO activation of DREADDs in lobule VI eyeblink area (p<10−10, two-way ANOVA) and in crus I (p<10−6). (D) In adult-disrupted mice, removal of CNO after 11 sessions of training resulted in recovery of conditional responses in both affected acute groups (crus I and eyeblink region). Error bars show mean ±SEM.
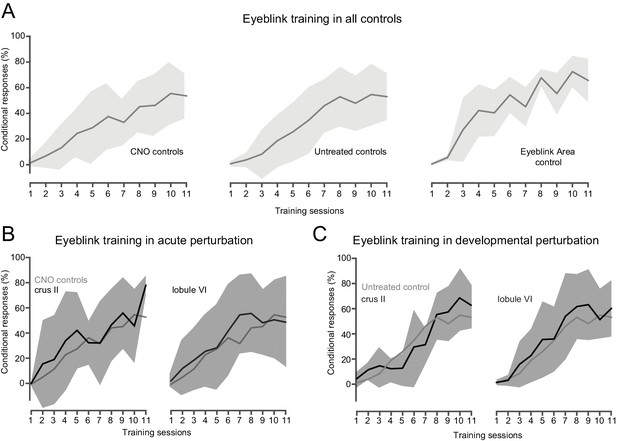
Blockade of eyeblink conditioning by DREADD activation in eyeblink-relevant regions.
(A) All control groups showed normal acquisition of eyeblink conditioning. Conditional responses (CRs) were quantified as normalized eyelid closure relative to US response, measured from CS-US paired trials. Experimental data represent averages (solid lines) and standard deviations (shaded areas) of all control mice used in this study segregated by the type of controls (untreated controls: 16 adult C57BL/6J male mice that underwent headplate surgery and no drug administration; Eyeblink Area controls: five adult C57BL/6J male mice that underwent AAV8-hSyn-hM4D(Gi)-mCherry injections into the eyeblink zone, headplate surgeries and were administered saline throughout training; CNO controls: nine adult C57BL/6J male mice that received headplate surgeries and were administered CNO throughout training). (B) No differences in eyeblink acquisition were observed in mice expressing DREADDs in crus II and lobule VI under acute CNO treatment. (C) Administration of CNO during development in mice expressing DREADDs in crus II and lobule VI had no effect on learning.
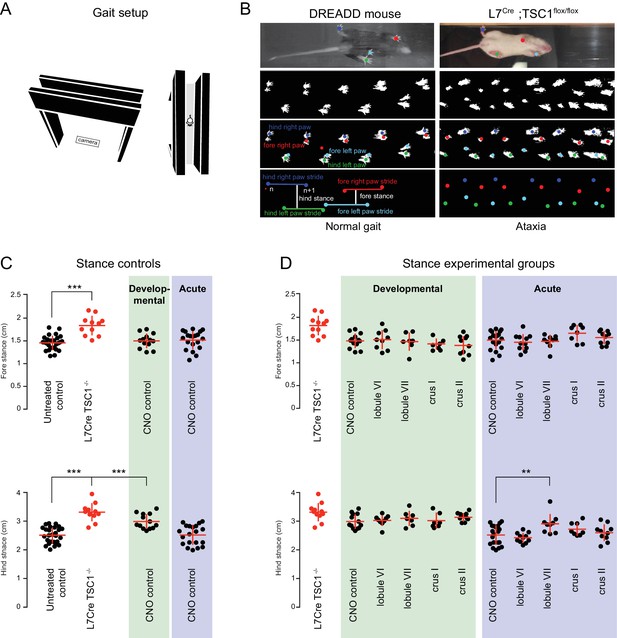
Mild gait impairments arise from prolonged CNO exposure.
(A) Schematic of gait apparatus. A camera was placed below the plexiglas to record a mouse running the length of the track. (B) Raw gait videos were processed using a MATLAB graphical user interface to detect sequences of mouse locomotion. Left, images from a mouse expressing DREADDs in lobule VII after acute CNO administration. Right, images from an ataxic L7Cre;Tsc1flox/flox mutant adult mouse used as a positive control. Stride lengths for each paw were calculated as the average distance between successive paw placements (see Video 2). Fore and hind stances were calculated as the average distance between fore and hind paws measured in the direction of the locomotion. (C) Stance measurements revealed no acute (blue shading) or developmental (green shading) effects of CNO on fore stance when compared to untreated controls (no shading). L7Cre;Tsc1flox/flox mice were used as positive controls (red). Developmental exposure to CNO resulted in an broader hind stance (CNO control developmental vs. control p<0.0001, one-way ANOVA). Hind stance in the L7Cre;Tsc1flox/flox mice was more severely affected (p<10−9). (D) Acute perturbation to lobule VII caused a mild broadening of hind (but not fore) stance when compared to CNO exposure time matched control (p<0.01, one-way ANOVA, see Video 3).
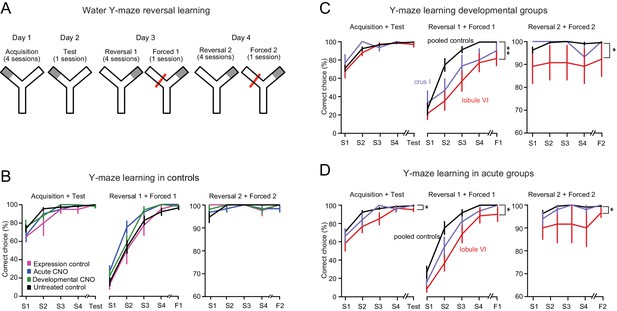
Impairment of swimming Y-maze reversal learning.
(A) Protocol for the Y-maze reversal assay consisting of initial training (day 1), test (day 2), and reversal (days 3 and 4). (B) All control groups showed normal reversal learning. Data represent averages of all control mice segregated by the type of controls. (C) Reversal learning was impaired by developmental activation of DREADDs in lobule VI and crus I but not in control mice (see Figure 4—figure supplement 1 and Videos 4 and 5). (D) Reversal learning was impaired by acute activation of DREADDs in lobule VI. Data with error bars are plotted as mean ±SEM. *p<0.05; **p<0.01.
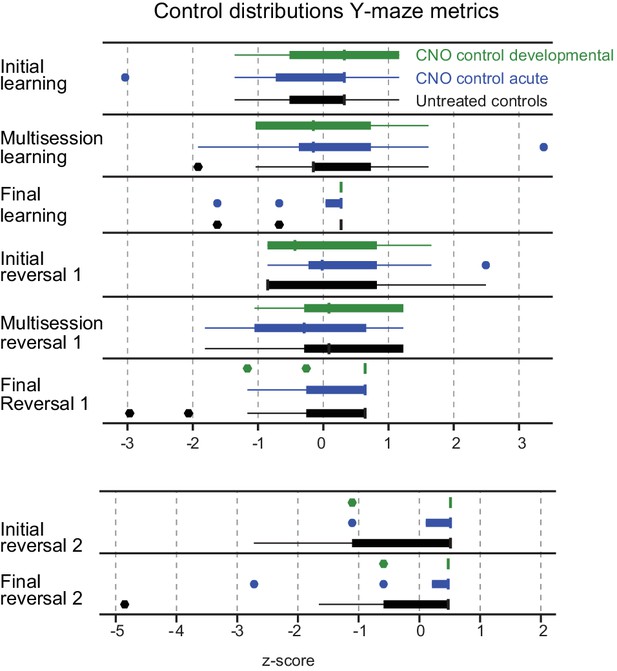
Control distributions of y-maze metrics.
No differences were detected in distributions of three z-score normalized control groups for any of eight Y-maze assay measures (as defined in Table 2). Boxplot outliers were defined as points outside the interquartile range (IQR) by more than 1.5 IQR.
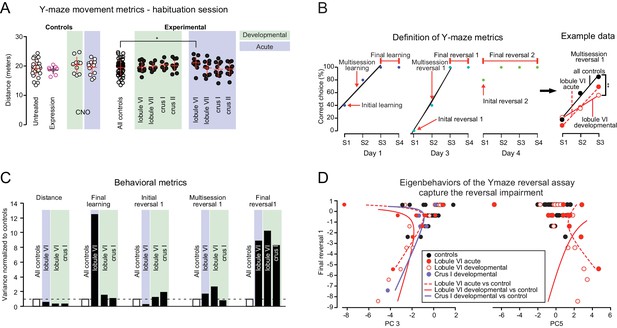
Specific impairment of learning parameters in the swimming Y-maze.
(A) Distance swum by control and experimental groups during the habituation phase of the swimming Y-maze. The only change observed was a mild increase after acute perturbation of lobule VI (p<0.05, one-way ANOVA). (B) Nonmotor, learning-related performance measures (for exact definitions see Table 2). (C) In experimental groups, variance in behavioral metrics is greater for learning metrics than for movement. (D) Principal component analysis (see Figure 5—figure supplement 1) reveals eigenbehaviors that capture concerted nonmotor impairments in lobule VI acute, lobule VI development, and crus I development groups.
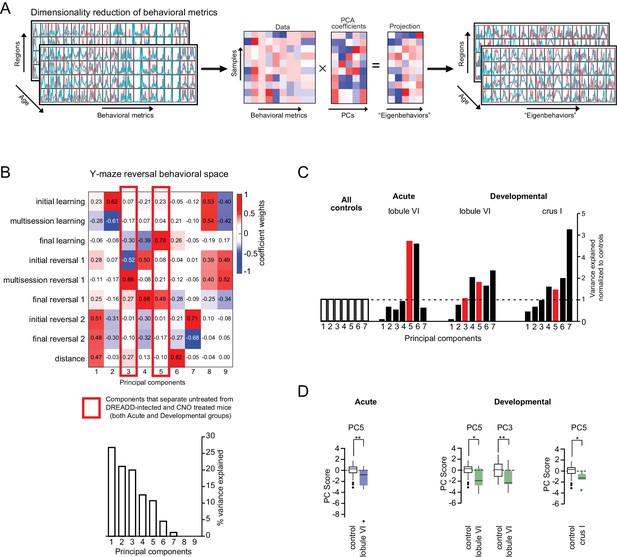
Dimensionality reduction of behavioral metrics and PCs of the Y-maze assay.
(A) Principal components (PCs) were computed from the control distributions to identify covariation in groups of behavioral metrics; the resulting components can be considered ‘eigenbehaviors’. The experimental data was then projected onto the eigenbehaviors and only dimensions that captured significant variance were kept. The reprojected data captures the original variation of the raw behavioral data using fewer dimensions. (B) Control-group principal components of Y-maze measures. Colors indicate coefficient weights corresponding to behavioral measures from each assay (as defined in Table 2). PCs were computed using normalized control-mouse measures (pooled controls, acute and developmental CNO controls). Red outlines indicate PCs for Y-maze metrics that separated the control and cerebellar-perturbation groups. (C) The variance captured by specific PCs, normalized to the control group PC variance, demonstrates that experimental groups vary in dimensions orthogonal to the control group. (D) The PC z-scores for PC3 and PC5 contributions show statistically significant separation between controls and lobule VI acute; and crus I and lobule VI developmental perturbation. *p<0.05; **p<0.01; ***p<0.001.
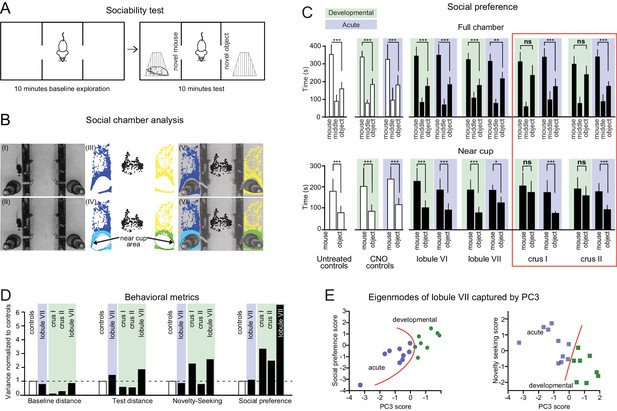
Cerebellar developmental impairment in a three-chamber social preference task.
(A) The three-chamber social preference test. (B) Interaction times with under-cup mouse and object were quantified using automated detection of near-cup approaches (see Figure 6—figure supplement 1). (C) Specific impairments in social preference after developmental perturbation of crus I or crus II but not other lobules, as measured by both time spent anywhere in the chamber and time spent near the cup. Error bars indicate mean ±SD. (D) Variance in social behavior metrics points to a stronger effect of cerebellar perturbation on non-motor metrics than on movement. (E) Separation of individual mice according to principal component analysis (see Figure 6—figure supplement 2). Orange curves indicate a boundary between opposite effects in acutely and developmentally perturbed lobule VII mice. Individual behavioral metrics were insufficient to separate the two groups (see Figure 6C). *p<0.05; **p<0.01; ***p<0.001.
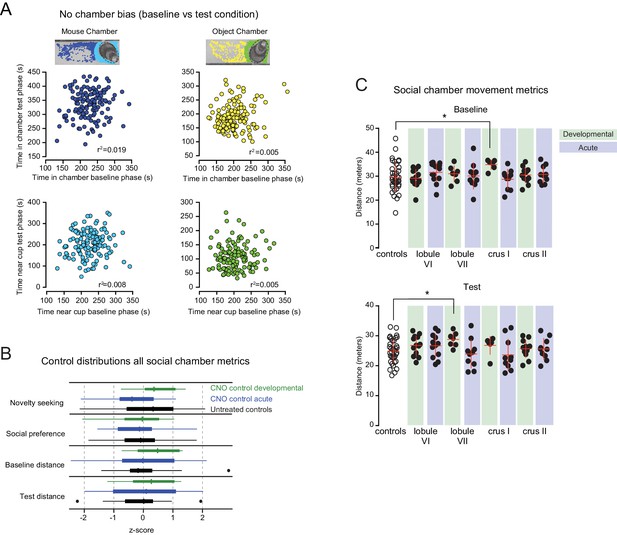
Social chamber metrics.
(A) Lack of correlation between test phase bias and baseline bias, indicating the lack of identifiable environmental influence. (B) No differences were detected in distributions of the three z-score-normalized control groups for any social chamber assay measures (as defined in Table 2). Plotted outliers were defined as points outside the interquartile range (IQR) by more than 1.5 IQR. (C) Movement in social chamber, measured by distance traveled, was assessed during baseline and test phases. Crus I developmental mice displayed increased distance traveled compared to controls in baseline. In the test phase, lobule VII developmental mice displayed increased distance traveled compared to controls. No other differences were detected.
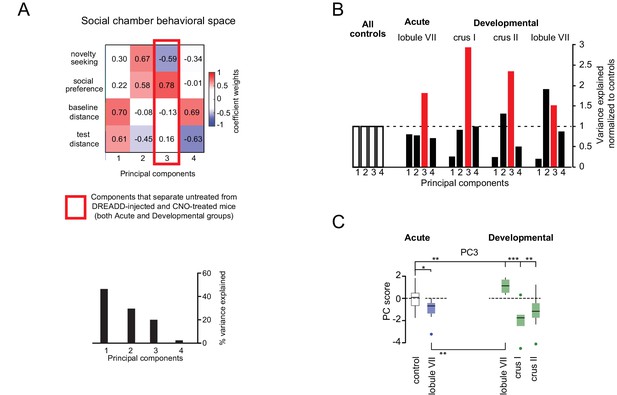
Social chamber principal component analysis.
(A) Control-group principal components of social chamber assay measures (as defined in Table 2). The red outline indicates the one eigenbehavior (PC3), representing strong negative covariation of social preference and novelty-seeking, which was significantly affected by lobule-specific perturbations. (B) Variance contribution of lobule-specific PCs, normalized to controls. (C) Mouse-by-mouse z-scored contributions from PC3 showing statistically significant separation between controls and lobule VII, crus I, and crus II developmental perturbation. *p<0.05; **p<0.01; ***p<0.001.
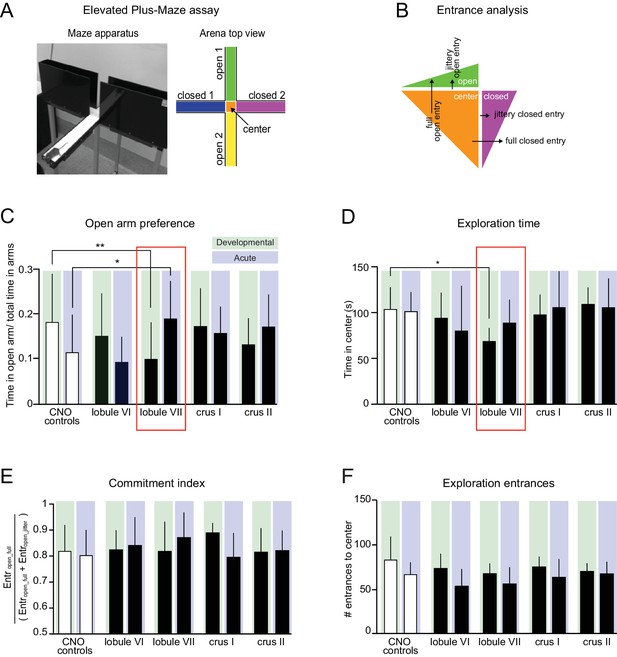
Lobule VII-dependent developmental and acute impairment of exploratory behavior.
(A) Elevated plus-maze (EPM). Right, schematic view. (B) Entrance analysis. Entrances were defined as either full entries or jittery entries. (C) Opposite effects on open-arm preference were found between developmental and acute groups. Developmental perturbation of lobule VII led to reduced open-arm preference. Acute perturbation of lobule VII led to increased open-arm preference (one-way ANOVA, p<0.05, d = 0.92, Šidák’s multiple comparisons post-hoc test p=0.02). (D) Developmental perturbation of lobule VII led to reduced exploration time. (E) Analysis of exploration time commitment index (as defined in Table 2) found no difference between groups. (F) Analysis of exploration entrances in EPM found no difference between groups. Error bars indicate mean ±SD. (Figure 7—figure supplement 1 and Figure 7—figure supplement 2).
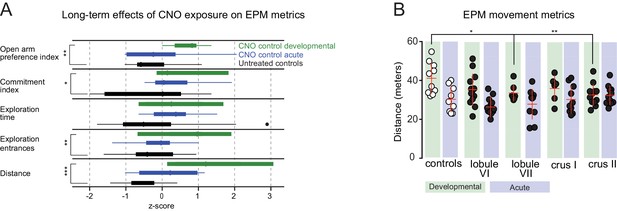
EPM metrics.
(A) CNO developmental controls displayed increased open-arm preference, commitment index, exploration entrance, and distance traveled compared to untreated controls in distributions of the three z-score-normalized control groups. (B) In EPM, no differences in distance traveled were detected for acutely perturbed mice. Lobule VII and crus II perturbations displayed reduced distance traveled compared to controls.
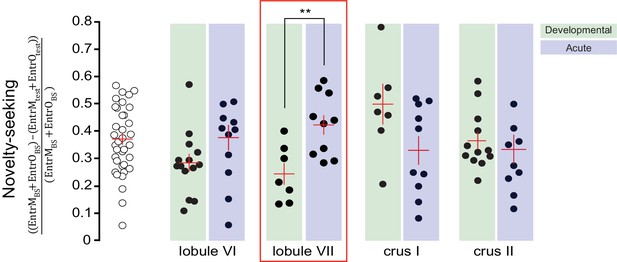
Novelty-seeking shows opposite effects of acute and developmental perturbation to lobule VII.
Novelty-seeking was defined as the difference between summed entrances to mouse (EntrM) and object (EntrO) chambers in test (test) and baseline (BS) sessions relative to baseline session. Novelty-seeking was oppositely affected by acute vs. developmental perturbation to lobule VII (p<0.01, t-test).
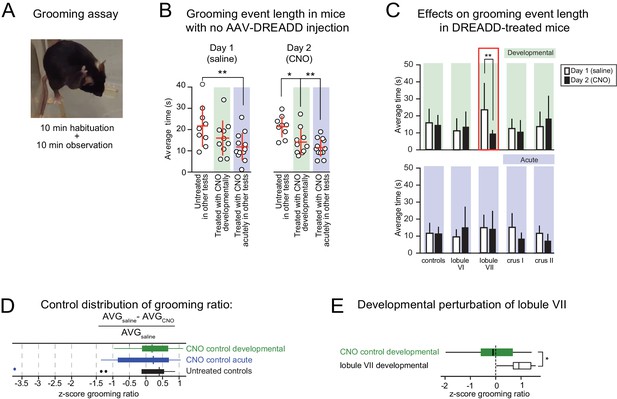
Lobule VII-dependent developmental perturbation of grooming.
(A) Grooming behavior was manually scored during a 10-min observation window and video-recorded for post-hoc corroboration. A grooming event was defined as a unilateral or bilateral stroke or full-body cleaning (Kalueff et al., 2016). Grooming events of less than 1-s duration were excluded from analysis. (B) Average grooming time in response to (left) saline injection on day 1 and (right) CNO injection on day 2 of testing in untreated mice (no AAV-DREADD injection or CNO) and mice that received CNO developmentally or acutely (color-shaded regions). (C) Injection-triggered grooming in DREADD-treated mice and corresponding controls. Bar graphs show average grooming time in response to the same 2-day injection protocol as in (B). The controls show the same data as the color shaded regions in (B). Only in developmental lobule VII mice was grooming time altered when CNO was injected rather than saline. (D) There were no differences in distributions of the z-score normalized three control groups for grooming ratio defined as the difference between average grooming bout length in ‘saline’ and CNO conditions relative to ‘saline’ condition. Outliers defined as points outside the interquartile range (IQR) by more than 1.5 IQR. (E) The change in grooming bout length between CNO and baseline condition was significant only in the developmental perturbation of lobule VII. **p<0.01.
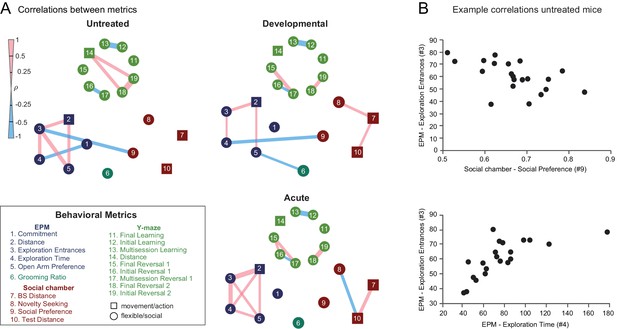
Developmental and acute perturbations induce new correlation structure between behavioral metrics.
(A) Mouse-by-mouse correlations between pairs of behavioral measures in control mice, developmentally DREADD-activated, and acutely DREADD-activated mice. Significant correlations (p<0.01, t-test) between individual behavioral metrics are indicated by colored bands whose thickness corresponds to Spearman's ρ. Chemogenetic perturbation induced within-task and between-task correlations not seen in untreated mice. (B) Scatter plots showing example relationships between pairs of behavioral measures.
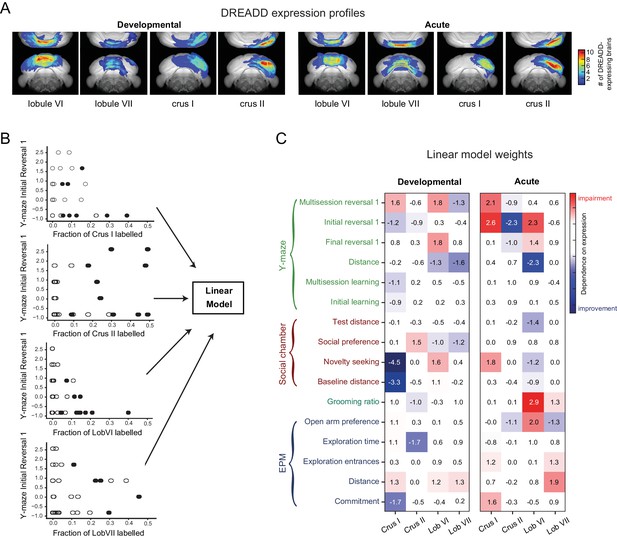
Quantitative contributions of lobules to behavioral metrics revealed using a linear model.
(A) Whole-brain reconstructions of recovered DREADD-mCherry expression. mCherry-positive voxels were recovered from reconstructions from serial two-photon tomographic images of developmentally and acutely perturbed lobule VI, lobule VII, crus I, and crus II mice (Figure 10—figure supplement 1 and Video 5). The color scale indicates how many brains showed expression at a particular location. (B) Linear models were used to evaluate the influence of fraction-of-lobule DREADD expression on each behavioral metric. Scatter plots demonstrate example relationships between fraction-of-lobule expression and individual behavioral metrics. Each dot represents one animal. Filled circles represent mice in which the majority of DREADD expression was found in the indicated lobule. (C) Regression weights of the best-fit model for each behavioral metric, normalized by the standard error of the weight estimate.
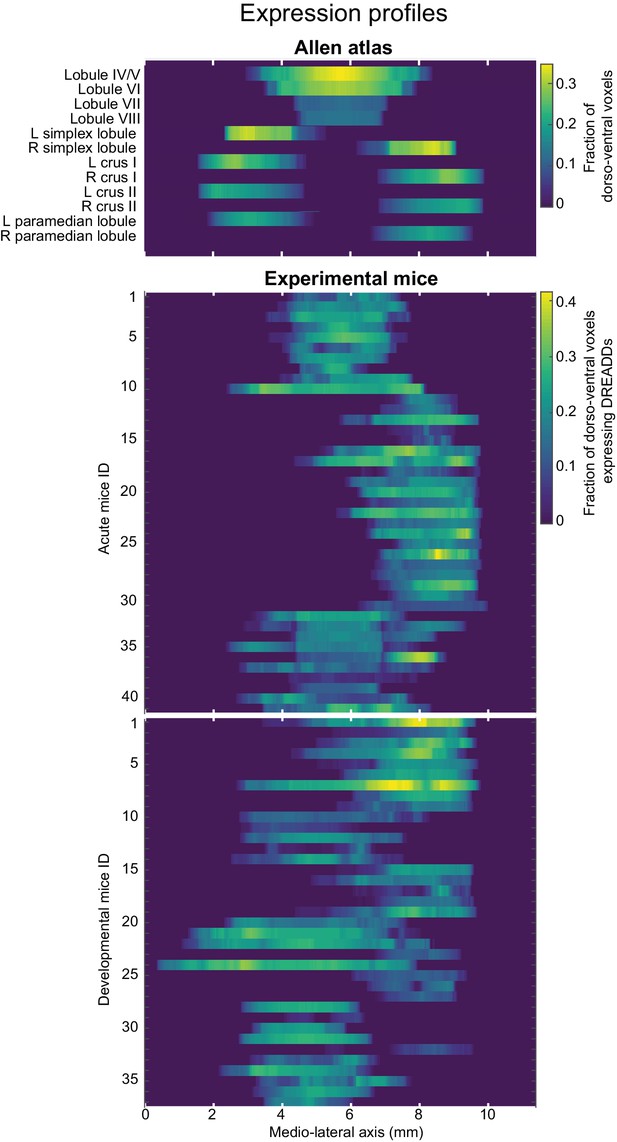
Whole-brain reconstructions of recovered DREADD-mCherry expression.
DREADD-positive distribution displayed along the medio-lateral axis by maximum projection of binary voxels over the anterior-posterior axis and then averaging over the dorsal-ventral axis as compared to the Allen Brain Atlas space. DREADD-positive voxels were recovered from reconstructed mCherry co-expression of serial two-photon tomographic images of lobule VI, lobule VII, crus I, crus II (top to bottom).
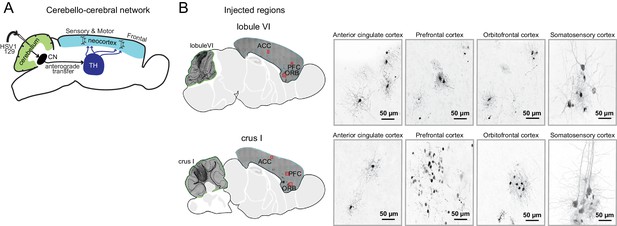
Transsynaptic tracing of cerebello-cortical projections.
(A) Cerebello-cortical pathways. HSV-H129 anterograde tracer injected into lobule VI or crus I reveals specific projections to neocortex (Figure 11—figure supplement 1). (B) Left, Example confocal microscopy images (10x) showing anti-GFP immunoreactivity for HSV-H129 positive cells in Top, lobule VI (lateral 0.36 mm) and Bottom, crus I (lateral 2.40 mm) to forebrain regions (anterior cingulate cortex, ACC; prefrontal cortex, PFC; orbitofrontal cortex, ORB; somatosensory cortex, SS). Right, Example confocal microscopy images (40x) of anti-GFP immunoreactivity for HSV-H129 positive cells in forebrain regions contralateral to the injection site (anterior cingulate cortex, ACC; prefrontal cortex, PFC; orbitofrontal cortex, ORB; somatosensory cortex, SS).
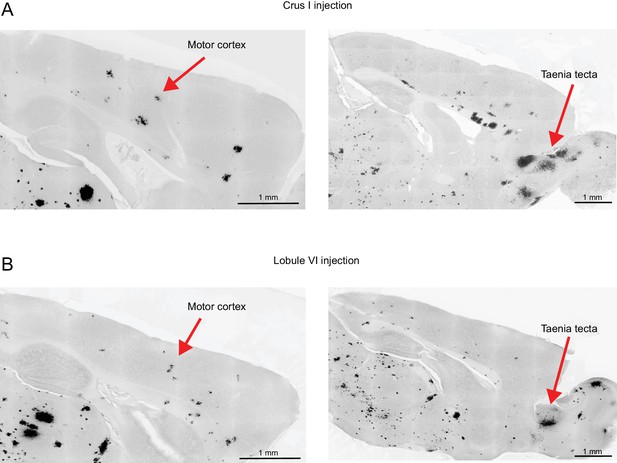
Transsynaptic tracing of cerebello-cortical projections to motor cortex and taenia tecta.
HSV-H129 anterograde tracer injected into (A) lobule VI or (B) crus I, revealing projections to motor cortex and taenia tecta.
Videos
Dense expression of hM4D(Gi)-mCherry in molecular layer interneurons.
https://doi.org/10.7554/eLife.36401.004Example of gait analysis.
Top. Raw movie played at four fps. Middle. Thresholded movie. Bottom. Tracked paws using ‘Manual Tracker’ plugin in Fiji.
Example of gait measurement.
https://doi.org/10.7554/eLife.36401.010Example of Y-maze reversal impairment.
https://doi.org/10.7554/eLife.36401.025Registration of the reconstructed cerebellum to the Allen Brain Atlas space.
Regions of interest were manually traced in from serial section two-photon images to yield volumetric reconstructions of both anatomical subdivisions as well as the injection spread. After registration to the Allen Brain Atlas, coordinates of the traced regions were transformed to the isotropic reference space.
Example of Y-maze tracking.
https://doi.org/10.7554/eLife.36401.033Tables
Summary of all mice.
https://doi.org/10.7554/eLife.36401.005Experimental group | Injection site | Behavioral assays | |||||
---|---|---|---|---|---|---|---|
Grooming | Social chamber | Y-maze | Elevated Plus Maze | Gait | Eyeblink | ||
Adult | Lobule VI | 12 | 12 | 12 | 12 | 12 | 8 |
Lobule VII | 10 | 10 | 7* | 10 | 10 | - | |
Crus I | 10 | 10 | 10 | 10 | 10 | 8 | |
Crus II | 11 | 11 | 11 | 11 | 11 | 6 | |
Eyeblink | - | - | - | - | - | 9 | |
*not counting three mice excluded from group comparison during habituation phase | |||||||
Developmental | Lobule VI | 13 | 13 | 13 | 13 | 13 | 6** |
Lobule VII | 8 | 8 | 8 | 8 | 8 | - | |
Crus I | 7 | 7 | 7 | 7 | 7 | 6** | |
Crus II | 12 | 12 | 12 | 12 | 12 | 3 | |
**not counting four mice (1 crus I, 3 lobule VI) excluded due to eyeblink-zone spillover expression | |||||||
Controls | Type | ||||||
Adult | DREADDs (eyeblink zone)+saline injections | - | - | - | - | - | 5 |
Saline i.p. | 9 | 9 | 9 | 9 | 9 | - | |
CNO alone | 10 | 10 | 10 | 10 | 10 | 9 | |
No treatment | 8 | 8 | 17 | 18 | 18 | 16 | |
GCaMP6f injections + CNO | - | - | 8 | - | - | - | |
Developmental | CNO alone | 10 | 10 | 10 | 10 | 10 | - |
Definitions of behavioral metrics.
https://doi.org/10.7554/eLife.36401.022# | Measure | Description | Quantification | Direction indicating impairment |
---|---|---|---|---|
Elevated Plus Maze metrics (EPM_) | ||||
1 | Commitment | Full entrances to the open arms relative to sum of full entrances and jittery entrances to the open arms () | experimental < controls | |
2 | Distance | Distance traveled (cm) during the ten minutes in the Elevated Plus Maze | experimental < controls | |
3 | Exploration Entrances | Entrances into the crossroads central area | experimental < controls | |
4 | Exploration Time | Time in the crossroads central area | experimental < controls | |
5 | Open-Arm Preference | Time in the open arms () relative to total time in closed and open arms | experimental < controls | |
Grooming metrics (GR_) | ||||
6 | Grooming Ratio | Difference betweenaverage grooming bout length in CNO and SALINE condition relative to SALINE condition | experimental > controls | |
Social Chamber metrics (SC_) | ||||
7 | Baseline Distance | Distance traveled (m) during baseline phase (10 min free exploration of the empty social chamber apparatus) | experimental < controls | |
8 | Novelty-Seeking | Difference between summed entrances to mouse and object chambers in test and baseline sessions, relative to baseline session | experimental < controls | |
9 | Social Preference | Time spent interacting with the novel mouse () relative to total time interacting with either the novel mouse or novel object () | experimental < controls | |
10 | Test Distance | Distance traveled (m) during test phase (10 min exploration of the social chamber apparatus with the novel mouse and object present) | experimental < controls | |
Y-maze metrics (YM_) | ||||
11 | Final Learning | Mean of the percent correct trials in acquisition sessions 3 and 4 | experimental < controls | |
12 | Initial Learning | Percent correct trials in acquisition session 1 | experimental < controls | |
13 | Multisession Learning | Slope of the linear regression of acquisition sessions 1 (ACQS1), 2 (ACQS2), and 3 (ACQS3) | linear regression slope of (ACQS1; ACQS2; ACQS3) | experimental < controls |
14 | Distance | Combined distance swum (m) in the three habituation trials (HAB1, HAB2 and HAB3) (60 s each) of free swimming in the empty Y-maze apparatus | + + | experimental < controls |
15 | Final Reversal 1 | Mean of the percent correct trials in reversal day 1 sessions 3 () and 4 | experimental < controls | |
16 | Initial Reversal 1 | Percent correct trials in reversal day 1 session 1 (RD1S1) | RD1S1 | experimental < controls |
17 | Multisession Reversal 1 | Slope of the linear regression of reversal day 1 sessions 1 (RD1S1), 2 (RD1S2) and 3 (RD1S3) | linear regression slope of (RD1S1; RD1S2; RD1S3) | experimental < controls |
18 | Final Reversal 2 | Mean of the percent correct trials in reversal day 2 sessions1 (RD2S1), 2 (RD2S2), 3 (RD2S3) and 4 (RD2S4) | experimental < controls | |
19 | Initial Reversal 2 | Percent correct trials in reversal day 2 session 1 | RD2S1 | experimental < controls |
Cohen’s d effect size of motor and non-motor metrics.
https://doi.org/10.7554/eLife.36401.023Flexible/Social/Learning metrics | |||||||||
---|---|---|---|---|---|---|---|---|---|
Y-maze reversal | Three-chamber | Elevated plus-maze | Grooming | Eyeblink conditioning | |||||
Learning(Finallearning) | Persistive behavior (Multisession reversal1) | Social preference | Novelty-seeking | Open-arm preference | Exploratory behavior | Grooming ratio | % Conditional responses session 11 | ||
Lobule VI | Developmental | 0.1 | 1.1 | −0.1 | 0.7 | 0.3 | 0.4 | −0.6 | −0.3 |
Acute | 0.5 | 0.9 | 0.2 | 0.0 | 0.3 | 0.6 | 0.1 | 0.1 | |
Lobule VII | Developmental | 0.6 | −0.3 | −0.3 | 1.1 | 0.9 | 1.7 | 1.2 | nd |
Acute | −0.6 | 0.3 | 0.9 | −0.4 | −0.9 | 0.5 | 0.2 | nd | |
Crus I | Developmental | 0.4 | 0.6 | 1.2 | −0.8 | 0.1 | 0.3 | 0.0 | 1.6 |
Acute | 0.0 | 0.3 | 0.0 | 0.3 | −0.6 | −0.1 | 0.9 | 1.0 | |
Crus II | Developmental | 0.1 | −0.5 | 1.2 | 0.0 | 0.6 | −0.3 | −0.5 | −0.5 |
Acute | −0.6 | −0.6 | 0.0 | 0.2 | −0.8 | −0.2 | 0.9 | 0.3 |
Movement metrics | |||||
---|---|---|---|---|---|
Gait | Y-maze reversal | Three-chamber | EPM | ||
Stance | Distance | Distance Baseline | Distance | ||
Lobule VI | Developmental | 0.1 | −0.1 | 0.1 | 0.7 |
Acute | −0.3 | −0.7 | −0.4 | 0.7 | |
Lobule VII | Developmental | 0.2 | −0.2 | −0.3 | 1.2 |
Acute | 0.5 | 0.1 | −0.1 | 0.3 | |
Crus I | Developmental | −0.3 | −0.4 | −1.1 | 0.7 |
Acute | 0.7 | 0.2 | 0.2 | 0.0 | |
Crus II | Developmental | 0.1 | −0.3 | −0.2 | 1.2 |
Acute | 0.3 | 0.2 | −0.2 | −0.3 |
-
Effect size, calculated in units (Cohen, 1988) of the two-sample pooled standard deviation, of perturbations on key behavioral parameters. Values in Bold indicate statistical significance. Colored fields indicate large effect sizes (d≥0.8); Blue for improvement of function and red for impairment as defined in Table 2.
-
Table 3—source data 1
Extended Table 3.
- https://doi.org/10.7554/eLife.36401.024
Relative neocortical expression resulting from long-distance tracing.
https://doi.org/10.7554/eLife.36401.032Injection site | ||
---|---|---|
Lobule VI | Crus I | |
Target Region | Relative expression | Relative expression |
Motor cortex | 1.00 | 1.00 |
Somatosensory | 0.66 | 1.23 |
Prelimbic | 0.71 | 0.32 |
Orbitofrontal | 0.72 | 0.22 |
Anterior cingulate | 0.42 | 0.59 |
Infralimbic | 0.28 | 0.28 |
Visual cortex | 0.25 | 0.27 |
Parietal association | 0.21 | 0.02 |
Retrosplenial | 0.11 | 0.17 |
Agranular insular | 0.03 | 0.00 |
Reagent type (species) or resource | Designation | Source or reference | Identifiers | |
---|---|---|---|---|
Gene | NA | |||
Strain, strain background | Mouse: C57BL/6J | The Jackson Laboratory, Bar Harbor, ME | Stock#: 00664 |Black6 https://www.jax.org/strain/000664 | |
Strain, strain background | Mouse: L7Cre;Tsc1flox/flox | From Tsai et al. (2012);Kloth et al., 2015 | ||
Genetic reagent | NA | |||
Cell line | NA | |||
Transfected construct | NA | |||
Biological sample | NA | |||
Antibody | anti-GFP Chicken | Aves Labs | Cat#GFP-1020; RRID: AB_10000240 | |
Antibody | Goat anti-Chicken IgY (H + L) Secondary Antibody, Alexa Fluor 647 | ThermoFisher | Cat#A-21449; RRID: AB_2535866 | |
Recombinant DNA reagent | AAV8-hSyn-hM4D(Gi)-mCherry | University of North Carolina Vector Core | ||
Recombinant DNA reagent | AAV1.Syn.GCaMP6f.WPRE.SV40 | Penn Vector Core | lot AV-1-PV2822 | |
Recombinant DNA reagent | HSV1 strain H129 | Wojaczynski et al. (2015); DOI: 10.1007/s00429-014- 0733-9 | H129 772 | |
Sequence-based reagent | NA | |||
Peptide, recombinant protein | NA | |||
Commercial assay or kit | Elevated Plus Maze (EPM) | Noldus | http://www.noldus.com/animal-behavior-research/solutions/research-small-lab-animals/elevated-plus-maze-set | |
Chemical compound, drug | Clozapine-N-oxide (CNO) | SIGMA-ALDRICH | Cat# C0832 | |
Chemical compound, drug | Dako Fluorescence Mounting Medium | Agilent | Cat# S302380-2 | |
Chemical compound, drug | 15% D-mannitol | SIGMA-ALDRICH | Cat# M4125 | |
Chemical compound, drug | DPBS | ThermoFisher | Cat#14190136 | |
Chemical compound, drug | White tempera paint | Artmind, Tempera Paint | Cat#10091773 | |
Chemical compound, drug | Rimadyl [carprofen] | Zoetis, Florham Park, NJ | http://www.zoetisus.com | |
Chemical compound, drug | Cholera toxin subunit B (CT-B), AlexaFluor 488 Conjugate | ThermoFisher | Cat# C34775 | |
Chemical compound, drug | Ketamine/xylazine | Met-Vet International/Akorn | RXV CIII (3N)/Cat# 59399-111-50 | |
Software, algorithm | Illustrator CS | Adobe | ||
Excel | Microsoft | |||
Ethovision ET | Noldus | |||
MATLAB | MathWorks | |||
Python 2.7.14 | Python | |||
ImageJ | NIH | |||
Neurolucida | (MBF Bioscience, VT, USA) | |||
Allen Brain Atlas | (Oh et al., 2014). | http://www.brain-map.org | ||
MiniAnalysis program | Synaptosoft Inc, Decatur, GA, USA | http://www.synaptosoft.com/ | ||
Other |
Additional files
-
Transparent reporting form
- https://doi.org/10.7554/eLife.36401.034