HIV-1 Latency: Coloring hidden viruses
Current antiviral therapies can suppress HIV-1 in the bloodstream to almost undetectable levels. Yet, if this therapy is interrupted, the number of viruses can start to increase again and go on to conquer the defenses of the immune system. This occurs when viruses that are in a dormant, or ‘latent’, state become reactivated. Characterized by limited gene expression and zero viral replication, latent viruses remain hidden from the immune system and are not affected by antiviral drugs, unless they are reactivated.
Latent HIV-1 is integrated within the genomes of immune cells, mainly resting CD4+ T cells and, to a lesser extent, macrophages (Churchill et al., 2016). Cells that represent latent reservoirs of HIV-1 are usually very rare and difficult to separate from the neighboring non-infected cells. This means that few of these cells have been available for study, which in turn has severely hampered our understanding of the mechanisms behind viral reactivation. Now, in eLife, Eric Verdin and colleagues – including Emilie Battivelli as first author – report on an improved ‘dual-color virus’ that allows cells harboring hidden latent viruses to be identified and isolated (Battivelli et al., 2018).
Over the past decade, much HIV-1 research has looked for ways to eliminate the latent viral reservoirs by first using pharmacological molecules to reverse latency in a ‘shock and kill’ approach (Deeks et al., 2012). The increased levels of gene expression in the reactivated viruses should lead to viral antigens being presented on the surface of latently infected cells. This in turn would allow the immune system to find and clear these cells and, typically, make the viruses susceptible to antiviral therapy again (Churchill et al., 2016; Margolis et al., 2016). However, this shock and kill approach only had limited success, mostly because it could not completely reactivate the virus from its latent state.
To develop more effective approaches for reactivating viruses we first need better ways to obtain resting CD4+ T cells that contain latent viruses in order to study them. So-called dual-color viruses – which have two fluorescent reporters under the control of different promoters – can help with this (Calvanese et al., 2013; Chavez et al., 2015). With the original version of this reporter virus (called HIVDuoFluoI), infected cells would glow red, making them clearly distinct from non-infected cells. If the integrated virus was active, a green fluorescent protein was also produced and the cells appeared both red and green. Latently infected cells (i.e., those with integrated yet inactive virus) could thus easily be distinguished by their pure red color.
Although this is how the tool should have worked in theory, there was room for improvement. Some of the sequences used in HIVDuoFluoI could readily recombine, meaning this dual-color virus was prone to losing its reporters, which made it impossible to track reliably. Battivelli et al. – who are based at the Gladstone Institutes, UCSF, the Buck Institute for Research on Aging and other institutes across the United States, Sweden and Brazil – overcame this specific issue by changing some sequences to create an improved dual-color virus. The new version, called HIVGKO, contains a different green reporter (a codon-switched eGFP) under the control of the HIV-1 specific promoter. It also has an unrelated orange, rather than red, fluorescent protein (mutated Kusubira Orange) under the control of the constitutive promoter.
HIVGKO allowed Battivelli et al. to examine the integration sites of latent viruses that could be reactivated and to understand how the genetic material around those sites was packaged in the nucleus (also known as the ‘chromatin context’). They could then compare these results to those from the viruses that could not be reactivated. Battivelli et al. designed their study to compare the potency of several drugs that were known to reactivate latent reservoirs via different mechanisms (Barton et al., 2016; Conrad et al., 2017; Mehla et al., 2010).
All the drugs tested showed limited reactivation unless they were used in combination. Battivelli et al. then mapped HIV-1 insertions from three different groups of infected cells. The first group contained cells with an integrated virus that was actively producing copies of itself – or productively infected cells (Figure 1). The second and third groups were non-reactivated and reactivated latently infected cells, respectively. Battivelli et al. further defined the chromatin context of the integrated viruses, and found that viruses within both productively infected cells and reactivated latently infected cells reside mainly in the active chromatin of transcribed genes and enhancers (Chen et al., 2017). Their analysis showed that viruses found in non-reactivated latently infected cells are detected within large genomic regions that interact with the dense meshwork of proteins that line the inner surface of the nucleus envelope, the nuclear lamina (Guelen et al., 2008; Marini et al., 2015). Some repressed viruses that could be reactivated were also found in the same region in reactivated latently infected cells.
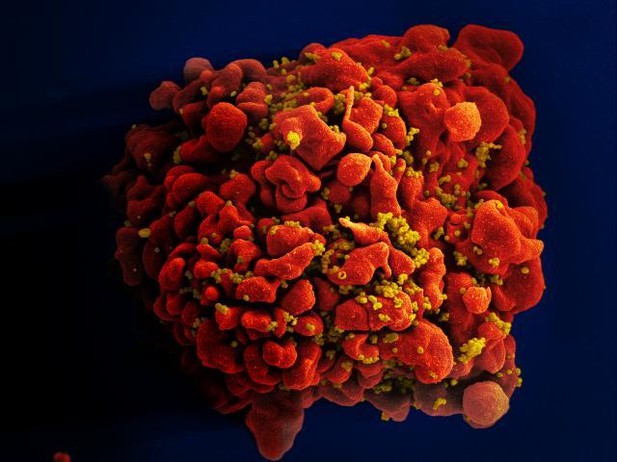
Scanning electron micrograph of an HIV-infected T cell.
The human immunodeficiency virus (HIV; yellow) buds from the surface of a productively infected T cell (red).
IMAGE CREDIT: NIAID.
The fact that all the drugs tested only partially reactivated a small portion of latent viruses implies that latent reservoirs of HIV-1 are heterogeneous in nature. This finding also clearly points to the fact that transcriptional repression of HIV-1 can be influenced by the context of where it integrates in the host cell’s genome. As this is a first study where this context could be connected directly to the fate of HIV-1 infection, it becomes clear that there are many lessons to be learned about how HIV-1 explores the human genome (especially in T cells) to integrate and persist.
References
-
Broad activation of latent HIV-1 in vivoNature Communications 7:12731.https://doi.org/10.1038/ncomms12731
-
Position effects influence HIV latency reversalNature Structural & Molecular Biology 24:47–54.https://doi.org/10.1038/nsmb.3328
-
HIV reservoirs: what, where and how to target themNature Reviews Microbiology 14:55–60.https://doi.org/10.1038/nrmicro.2015.5
-
Towards an HIV cure: a global scientific strategyNature Reviews Immunology 12:607–614.https://doi.org/10.1038/nri3262
Article and author information
Author details
Publication history
- Version of Record published: May 29, 2018 (version 1)
Copyright
© 2018, Lusic
This article is distributed under the terms of the Creative Commons Attribution License, which permits unrestricted use and redistribution provided that the original author and source are credited.
Metrics
-
- 1,692
- views
-
- 157
- downloads
-
- 0
- citations
Views, downloads and citations are aggregated across all versions of this paper published by eLife.
Download links
Downloads (link to download the article as PDF)
Open citations (links to open the citations from this article in various online reference manager services)
Cite this article (links to download the citations from this article in formats compatible with various reference manager tools)
Further reading
-
- Evolutionary Biology
- Microbiology and Infectious Disease
HERV-K(HML-2), the youngest clade of human endogenous retroviruses (HERVs), includes many intact or nearly intact proviruses, but no replication competent HML-2 proviruses have been identified in humans. HML-2-related proviruses are present in other primates, including rhesus macaques, but the extent and timing of HML-2 activity in macaques remains unclear. We have identified 145 HML-2-like proviruses in rhesus macaques, including a clade of young, rhesus-specific insertions. Age estimates, intact ORFs, and insertional polymorphism of these insertions are consistent with recent or ongoing infectious activity in macaques. 106 of the proviruses form a clade characterized by an ~750 bp sequence between env and the 3' LTR, derived from an ancient recombination with a HERV-K(HML-8)-related virus. This clade is found in Old World monkeys (OWM), but not great apes, suggesting it originated after the ape/OWM split. We identified similar proviruses in white-cheeked gibbons; the gibbon insertions cluster within the OWM recombinant clade, suggesting interspecies transmission from OWM to gibbons. The LTRs of the youngest proviruses have deletions in U3, which disrupt the Rec Response Element (RcRE), required for nuclear export of unspliced viral RNA. We show that the HML-8 derived region functions as a Rec-independent constitutive transport element (CTE), indicating the ancestral Rec-RcRE export system was replaced by a CTE mechanism.
-
- Microbiology and Infectious Disease
The target of rapamycin (TOR) signaling pathway is highly conserved and plays a crucial role in diverse biological processes in eukaryotes. Despite its significance, the underlying mechanism of the TOR pathway in Aspergillus flavus remains elusive. In this study, we comprehensively analyzed the TOR signaling pathway in A. flavus by identifying and characterizing nine genes that encode distinct components of this pathway. The FK506-binding protein Fkbp3 and its lysine succinylation are important for aflatoxin production and rapamycin resistance. The TorA kinase plays a pivotal role in the regulation of growth, spore production, aflatoxin biosynthesis, and responses to rapamycin and cell membrane stress. As a significant downstream effector molecule of the TorA kinase, the Sch9 kinase regulates aflatoxin B1 (AFB1) synthesis, osmotic and calcium stress response in A. flavus, and this regulation is mediated through its S_TKc, S_TK_X domains, and the ATP-binding site at K340. We also showed that the Sch9 kinase may have a regulatory impact on the high osmolarity glycerol (HOG) signaling pathway. TapA and TipA, the other downstream components of the TorA kinase, play a significant role in regulating cell wall stress response in A. flavus. Moreover, the members of the TapA-phosphatase complexes, SitA and Ppg1, are important for various biological processes in A. flavus, including vegetative growth, sclerotia formation, AFB1 biosynthesis, and pathogenicity. We also demonstrated that SitA and Ppg1 are involved in regulating lipid droplets (LDs) biogenesis and cell wall integrity (CWI) signaling pathways. In addition, another phosphatase complex, Nem1/Spo7, plays critical roles in hyphal development, conidiation, aflatoxin production, and LDs biogenesis. Collectively, our study has provided important insight into the regulatory network of the TOR signaling pathway and has elucidated the underlying molecular mechanisms of aflatoxin biosynthesis in A. flavus.