Dlk1-Dio3 locus-derived lncRNAs perpetuate postmitotic motor neuron cell fate and subtype identity
Figures
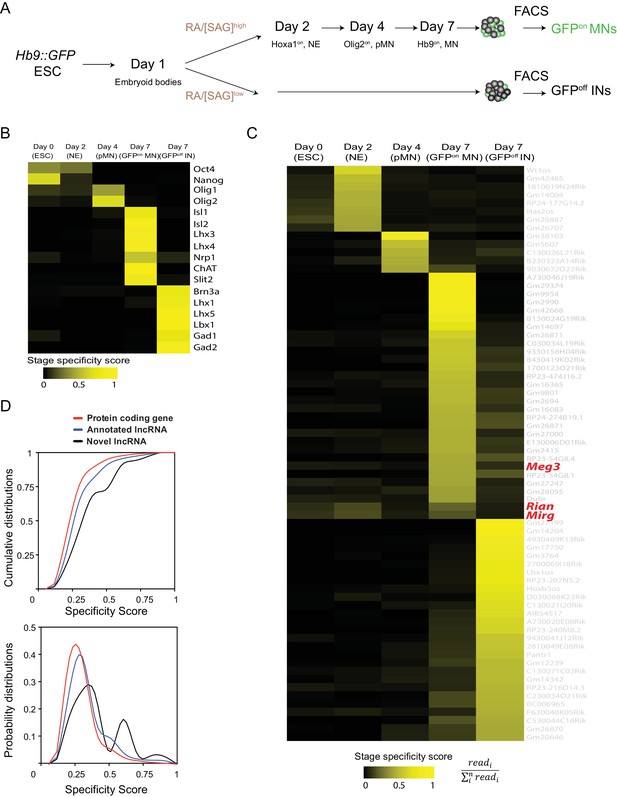
Identification of cell-type-specific lncRNAs during motor neuron differentiation
(A) Schematic illustration of the differentiation process from Hb9::GFP ESCs to spinal MNs. RA: retinoic acid. SAG: Smoothened agonist. ESC: embryonic stem cell. NE: neural epithelium. pMN: motor neuron progenitor. MN: motor neuron. IN: interneuron. (B and C) Heatmaps presenting the abundances of known cell transcription factors (B) and the abundances of lncRNA signatures (C) across each stage from ESCs to postmitotic MNs and INs (color indicates specificity scores). (D) Cumulative distributions (above) and probability distributions (below) of the stage specificity score of different categories of genes (protein coding genes [red], annotated lncRNAs [blue] and novel lncRNAs [black]), representing a measure of differential expression for each transcript across the cell types. The distribution reveals that annotated lncRNAs and novel lncRNAs manifest significantly higher specificity (according to Kolmogorov-Smirnov tests) than protein-coding genes.
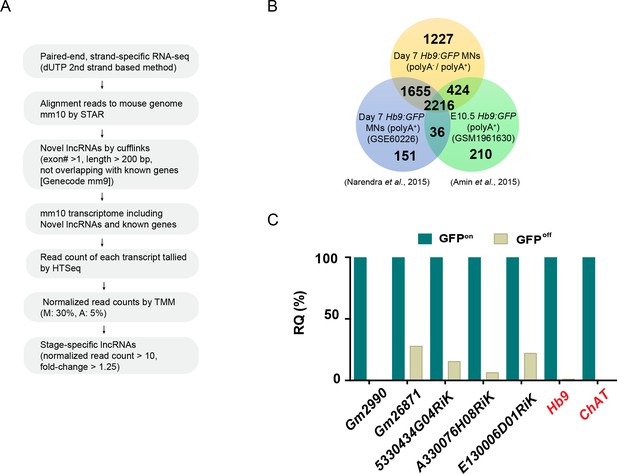
Systematic identification and verification of motor neuron signature lncRNAs
(A) Workflow of the RNA-seq analysis to identify cell-type-enriched lncRNAs. (B) The strand-specific RNA-seq performed in this study (orange) identified many more lncRNAs compared to previously published studies (blue and green) that used polyA+ selection RNA-seq. (C) Identified MN-signature lncRNAs were verified by qPCR from FACS-sorted GFPon MNs, whereas GFPoff INs were used to reflect their specificity and relative quantity (RQ). Hb9 and ChAT are mature MN markers.
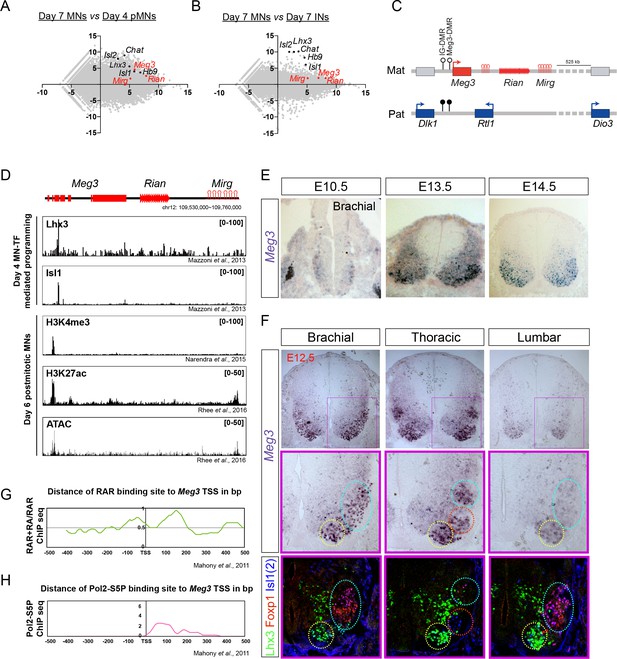
Dlk1-Dio3 locus-derived lncRNAs are enriched in postmitotic motor neurons
(A and B) MA plots demonstrate that Meg3, Rian, and Mirg are postmitotic (day 7 MNs vs. day 4 pMNs; A) MN signature (day 7 MNs vs. day 7 INs; B) lncRNAs. X-axis: mean abundance; Y-axis: log2 fold-change. (C) Illustration of the imprinted Dlk1-Dio3 locus. The lncRNAs of the maternally-inherited allele (in red) are on mouse chromosome 12. The miRNA and C/D snoRNA genes are shown by hairpin loops and triangles, respectively. (D) Comparison of ChIP-seq for MN transcription factors (Lhx3 and Isl1), H3K4me3, and H3K27ac, together with ATAC-seq of the Dlk1-Dio3 locus. (E) In situ hybridization shows that Meg3 is gradually enriched and restricted in postmitotic MNs in the developing spinal cord. (F) In situ hybridization together with adjacent sections of immunostaining on E12.5 segmental spinal cords demonstrate that Meg3 is enriched at brachial and thoracic MNs (Isl1/2on), but no preference for columnar MN subtypes was revealed for Foxp1 or Lhx3. (G and H) RAR binding and recruitment of the elongating form of Pol2 to the Meg3 transcription start site (TSS) occur within 8 hr of retinoic acid (RA) exposure.
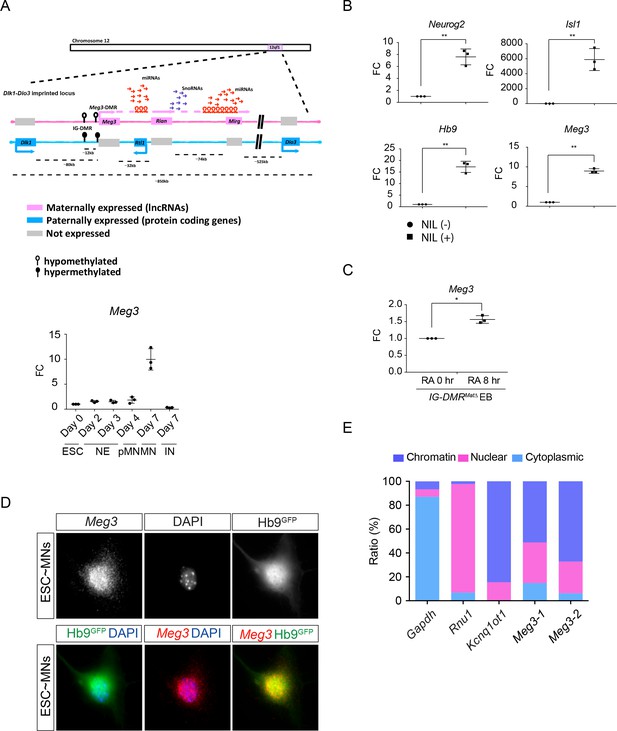
Characterization of the lncRNAs in the Dlk1-Dio3 locus
(A) Illustration of the imprinted Dlk1-Dio3 locus. The lncRNAs on the maternally-inherited allele (in pink) and protein-coding genes from the paternally-inherited allele (in blue) are depicted. miRNA genes are shown by hairpin loops. Arrows indicate the transcription directions. The IG-DMR site is hypomethylated (open circles) on the maternally-inherited allele, but hypermethylated (filled circles) on the paternally-inherited allele. Lower panel shows the qPCR analysis of Meg3 expression in ESCs, NEs, pMNs, MNs and INs (n = 3 independent experiments; FC: fold-change). (B and C) qPCR reveals that MN transcription factors (Neurog2-Isl1-Lhx3), as long as adding RA, can induce Meg3 expression in the IG-DMRmatΔ ESCs (FC: fold-change; error bars represent SD, n = 3, * p-value<0.05, ** p-value<0.01 by Student’s t-test). (D) Panels show single molecule RNA FISH of Meg3 in Hb9::GFP ESC~MNs. (E) Subcellular fractionation of ESC~MNs revealed that Meg3 is not only enriched in the nuclei, but also highly chromatin-associated. Two independent Meg3 primers were used. Gapdh, Rnu1, and Kcnq1ot1 were used to reflect the purity of cytoplasmic, nuclear, and chromatin-associated fractions, respectively.
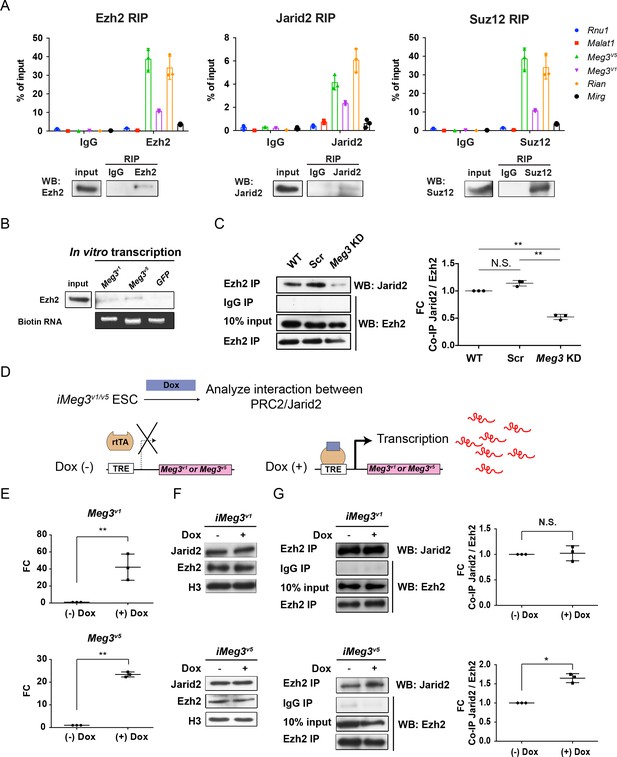
Meg3 facilitates the non-stoichiometric interaction of the PRC2 complex and Jarid2
(A) Ezh2, Jarid2, and Suz12 immunoprecipitation specifically retrieves Meg3 RNA isoforms (v1 and v5). Rnu1 RNA and Malat1 lncRNA are negative controls. 10% input was used to normalize the retrieval efficiency (error bars represent SD, n = 3 independent experiments). Immunoblotting reflects the recovery of Ezh2, Jarid2 and Suz12 proteins using the corresponding antibodies. (B) In vitro-transcribed, biotinylated Meg3 RNA isoforms retrieved Ezh2. (C) Ezh2 interacts with Jarid2 in ESC~MNs, but knockdown of Meg3 impairs this interaction. The abundance of Jarid2 is shown on the right (N.S.: not significant; error bars represent SD, n = 3 independent experiments; ** p-value<0.01 by Student’s t-test). (D and E) The design of inducible ‘Tet-On’ ESC lines expressing Meg3v1 or Meg35 under the doxycycline (Dox)-regulated promoter. In the presence of Dox, the reverse tetracycline-controlled transactivator (rtTA) is recruited to the TRE (tetracycline response element), thereby initiating robust transcription of Meg3v1 or Meg3v5, respectively. (F) Overexpression of Meg3v1 or Meg3v5 does not alter the protein levels of Ezh2 or Jarid2. (G) Meg3v5 but not Meg3v1 stimulates more Ezh2 and Jarid2 interaction. The abundances of Jarid2 are shown on the right (FC: fold-change; N.S.: not significant; error bars represent SD, n = 3 independent experiments; * p-value<0.05, ** p-value<0.01 by Student’s t-test).
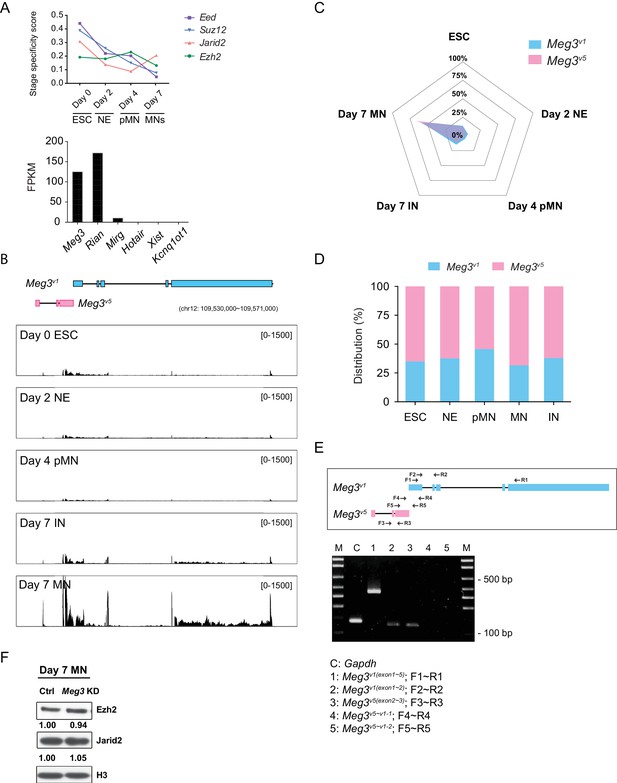
Characterization of Meg3 isoforms
(A) Upper panel: Time-series expression of the PRC2 subunits (Eed, Suz12, Jarid2, and Ezh2) during ESC~MN differentiation. The levels of PRC2 complex progressively decreased during differentiation, whereas Jarid2 is reactivated in day 7 postmitotic MNs. Lower panel: The abundances of the known lncRNAs that interact with PRC2 in MNs, as revealed by RNA-seq. (B) RNA-seq analysis of ESC~MNs. Reads from ESCs, RA-induced nascent neural epithelium (NE at day 2), MN progenitors (pMN at day 4), postmitotic interneurons (IN at day 7), and postmitotic MNs (MN at day 7). Reads are normalized to the total number of mappable reads per sample. RNA-seq revealed that Meg3v1 (blue boxes) and Meg3v5 (pink boxes) are the most abundant Meg3 isoforms in postmitotic MNs (GENCODE version M9). (C) Radar chart reveals that day 7 postmitotic MNs have the highest distribution of Meg3v5 during ESC~MN differentiation. (D) Histogram plot indicates that the Meg3v1 and Meg3v5 isoforms account for more than 99% of Meg3 transcripts during ESC~MN differentiation. (E) Schematic diagram of RT-PCR primer locations within the Meg3v1 and Meg3v5 regions. Expression of Meg3 according to different primer combinations for Meg3 isoforms suggests that Meg3v1 and Meg3v5 are independent transcripts. Gapdh as a loading control. (F) Western blot shows that the loss of Meg3 imprinted lncRNAs does not affect the protein abundance of Ezh2 and Jarid2 in ESC~MNs.
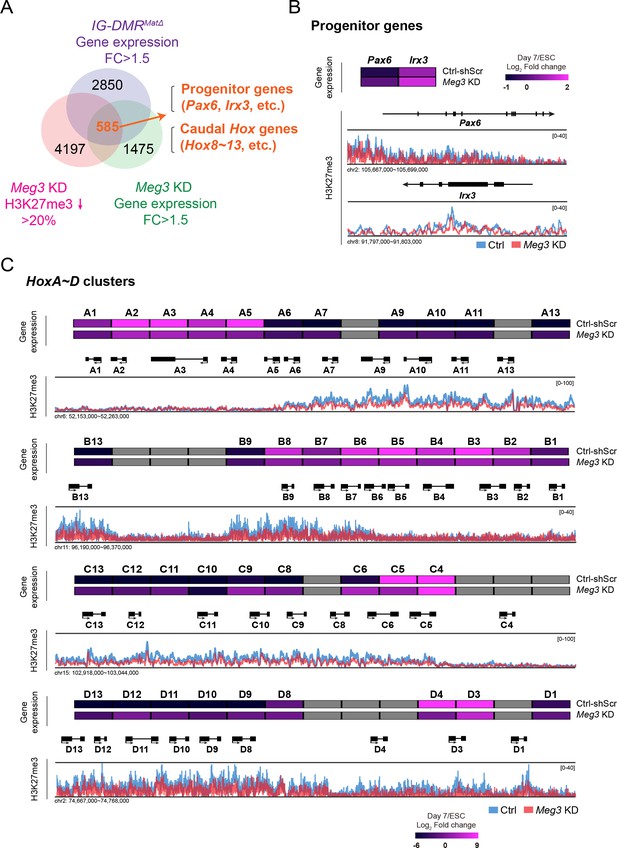
Loss of Dlk1-Dio3 locus-derived lncRNAs in MNs leads to dysregulation of neural progenitor and caudal Hox genes
(A) Genome-wide profiling of H3K27me3 by ChIP-seq and gene expression by Agilent microarray in control, IG-DMRmatΔ, and Meg3 KD ESC~MNs. Venn diagram shows the number of genes that are up-regulated in both IG-DMRmatΔ and Meg3 KD MNs while also displaying the reduction of H3K27me3 epigenetic landscape. Loss of Meg3 imprinted lncRNAs is related to the up-regulation of progenitor genes, as well as Hox genes. (B and C) Heatmaps illustrating the expression profiles of ESC~MNs in control scrambled and Meg3 KD lines. The color indicates the log2 fold-change in signal intensity between ESCs and ESC~MNs. Genes in light grey are not represented in the microarrays. Loss of Meg3 imprinted lncRNAs results in ectopic expression of progenitor genes in (B), and a majority of caudal Hox genes (Hox8~13) in (C), with concomitant down-regulation of H3K27me3 levels.
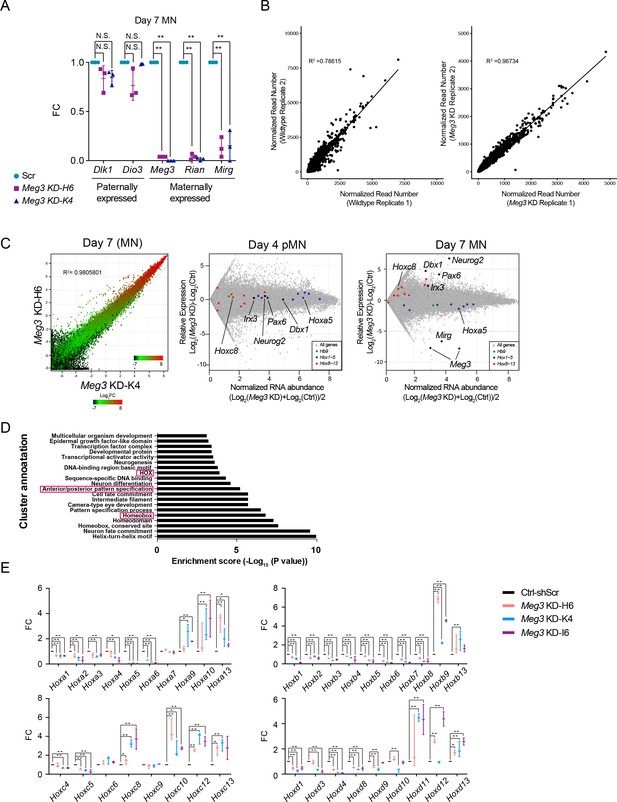
Characterization of Meg3 KD ESCs
(A) Expression of paternally-expressed protein coding genes (Dlk1 and Dio3) and maternally-expressed lncRNAs (Meg3, Rian, and Mirg) within the imprinted Dlk1-Dio3 locus in ESC~MNs, as revealed by qPCR (FC: fold-change; N.S.: not significant; error bars represent SD, n = 3 independent experiments; * p-value<0.05, ** p-value<0.01 by Student’s t-test). (B) The correlation plots and associated R2 values are indicated by comparing H3K27me3 of MN samples from two independent controls and Meg3 KD lines. (C) Left panel: The correlation plots and associated R2 values indicate that the two Meg3 KD lines infected by independent shRNAs are almost identical. Middle and right panels: MA plot of control versus Meg3 KD pMNs (middle) and MNs (right). At the postmitotic stage (day 7), Meg3 KD leads to up-regulation of the neural progenitor genes Pax6, Dbx1, Neurog2, Irx3 and caudal Hox genes (Hox8~13), as well as down-regulation of rostral Hox genes (Hox1~5). Two biological replicate experiments were used to generate the data. The generic MN marker Hb9 is unaffected, whereas Meg3 and Mirg are the most down-regulated genes after Meg3 KD. (D) Dysregulated genes are grouped by gene ontology (GO). (E) Loss of Meg3 leads to ectopic expression of most caudal Hox genes (Hox8~13), with concomitant down-regulation of rostral Hox genes (Hox1~5), as verified by qPCR. A third shRNA (I6) was further assessed and Hox expression was normalized against Gapdh expression levels (FC: fold-change; error bars represent SD, n = 3; * p-value<0.05, ** p-value<0.01 by Student’s t-test).
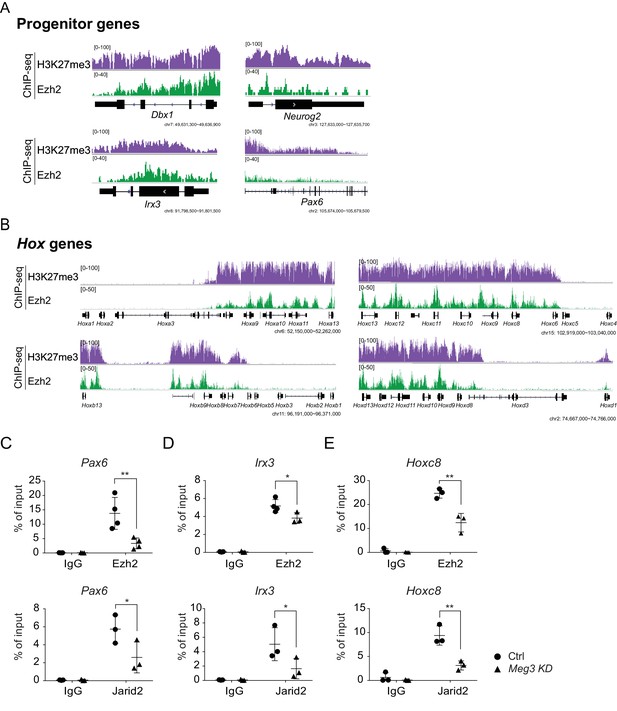
Ezh2 binding and the H3K27me3 landscape in ESC~MNs
(A and B) Concordant enrichments of Ezh2 and H3K27me3 in progenitor genes (A) and caudal Hox genes (B).(C~E) ChIP-qPCR verifies compromised H3K27me3 occupancy of Ezh2 and Jarid2 for the Pax6, Irx3, and Hoxc8 loci upon Meg3 KD. 1% input was used to normalize the retrieval efficiency (error bars represent SD, n = 3 independent experiments; * p-value<0.05, ** p-value<0.01 by Student’s t-test).
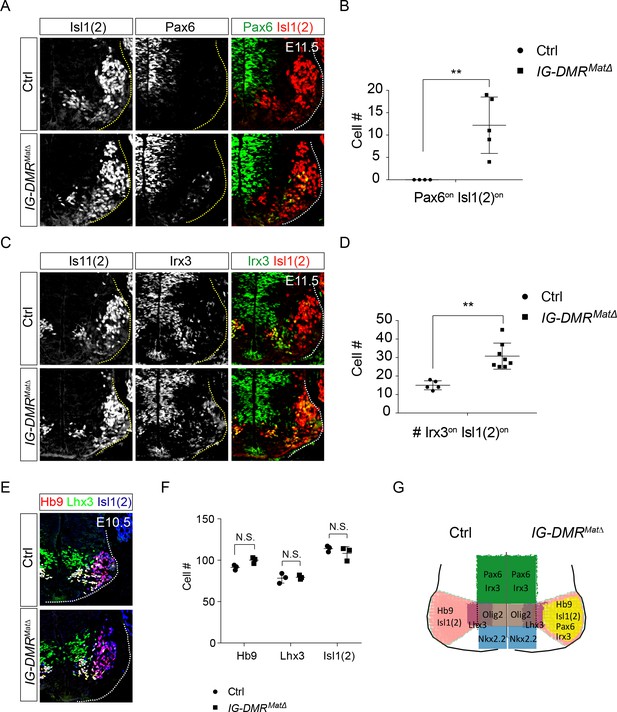
IG-DMRmatΔ mice ectopically turn on progenitor genes in postmitotic MNs
(A ~ D) IG-DMRmatΔ embryos display ectopic Pax6on and Irx3on cells in the postmitotic Is11(2)on MNs (partial penetrance for Pax6, n = 5/11 at E11.5; whereas Irx3 displays 100% penetrance, n = 8/8. Error bars represent SD, ** p-value<0.01 by Student’s t-test). (E) Generation of generic MNs (Hb9on, Lhx3on, and Isl1/2on) is not affected in spinal cords of IG-DMRmatΔ mice at E10.5. (F) Quantification of postmitotic MNs (number of positive cells per 15 μm brachial ventral-half sections) in wild type control and IG-DMRmatΔ embryos (error bars represent SD, n = 3 embryos at E10.5; N.S.: not significant by Student’s t-test). (G) Summary of ectopic progenitor gene expression in postmitotic MNs of the IG-DMRmatΔ embryos.
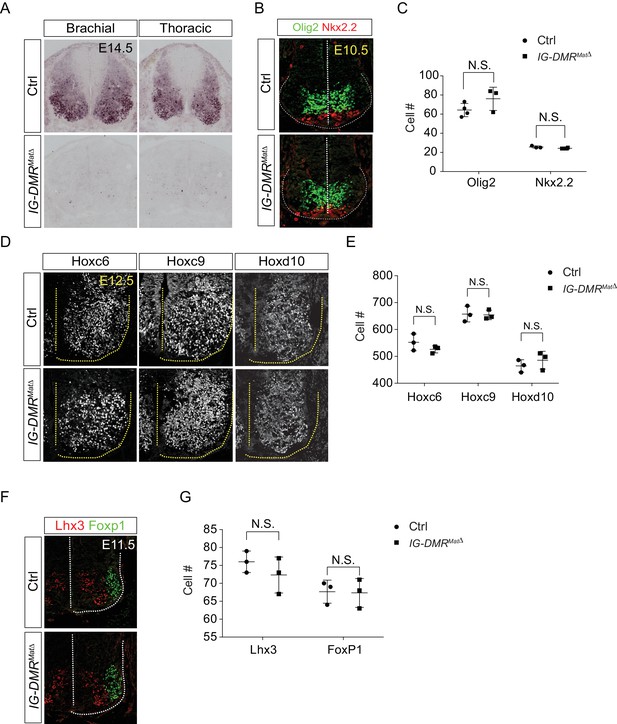
IG-DMRmatΔ mutant phenotype analysis
(A) Meg3 is absent from the spinal cords of IG-DMRmatΔ embryos at E14.5, as revealed by in situ hybridization. (B and C) Immunostaining analysis of MN progenitors Nkx2.2 and Olig2 shows comparable numbers in wild type control and IG-DMRmatΔ spinal cord sections at E10.5. Quantification of MN progenitors (number of positive cells per 15 μm brachial spinal cord ventral-half sections) in wild type control and IG-DMRmatΔ embryos revealed no significant differences amongst different segments from the spinal cord (error bars represent SD, n = 3 embryos at E10.5; N.S.: not significant) (D and E) Immunostaining analysis of segmental MNs (Hoxc6on at brachial, Hoxc9on at thoracic, and Hoxd10on at lumbar regions) shows comparable MN numbers for E12.5 wild type control and IG-DMRmatΔ spinal cord sections. Quantification of segmental MNs (number of positive cells per 15 μm brachial spinal cord ventral-half sections) in wild type control and IG-DMRmatΔ embryos revealed no significant differences amongst different segments from the spinal cord (error bars represent SD, n = 3 embryos at E12.5; N.S.: not significant). (F and G) Columnar axial MNs (Lhx3on) and limb-innervating MNs (Foxp1on) are unaffected in the IG-DMRmatΔ embryos. Quantification of columnar MNs (number of positive cells per 15 μm brachial spinal cord ventral-half sections) in wild type control and IG-DMRmatΔ embryos revealed no significant differences amongst different segments from the spinal cord (error bars represent SD, n = 3 embryos at E11.5; N.S.: not significant).
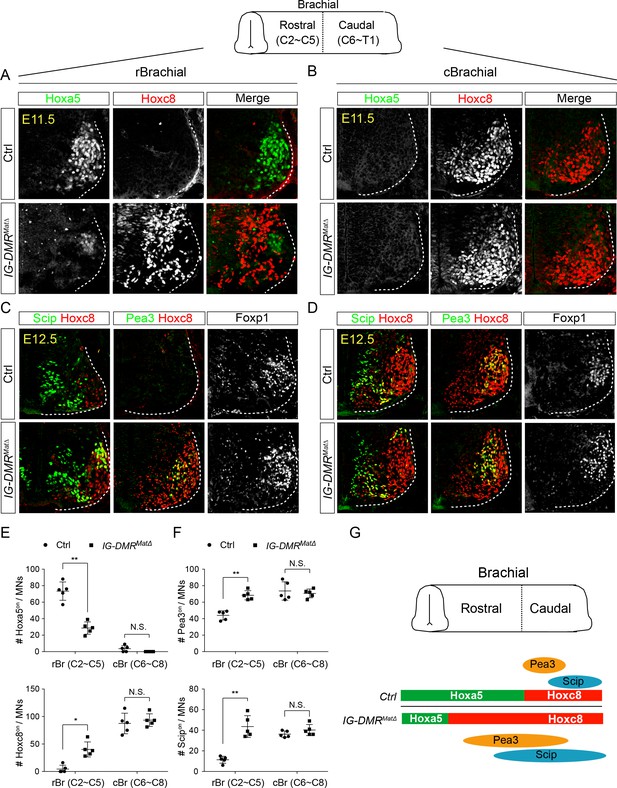
IG-DMRmatΔ mice manifest switched MN subtype identity
(A ~ D) Ectopic expansion of Hoxc8 and downstream Pea3on and Scipon MN pools in the rostral brachial segment, with a concomitant decrease of Hoxa5on MNs for E11.5~E12.5 IG-DMRmatΔ embryonic spinal cord sections. Expression of Hoxc8 in the caudal brachial region remains unaffected (B and D). (E and F) Quantification of the numbers and distributions of Hoxa5on, Hoxc8on, Pea3on, and Scipon MNs in the control and IG-DMRmatΔ mice from serial sections along the rostrocaudal axis (N.S.: not significant; error bars represent SD, n = 5; * p-value<0.05, ** p-value<0.01 by Student’s t-test). (G) Summary of the motor neuron phenotype in the IG-DMRmatΔ embryos.
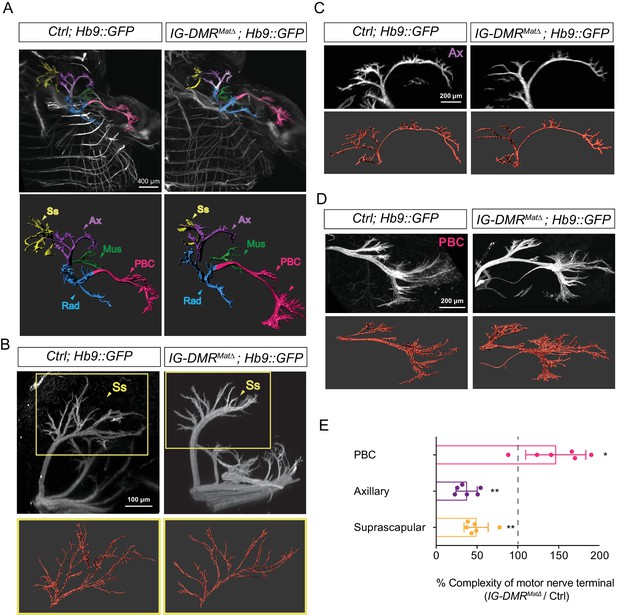
Motor axon innervation defects in the IG-DMRmatΔ embryos
(A) Panoramic views from different angles of control and IG-DMRmatΔ; Hb9::GFP embryos at E13.5 using light sheet microscopy (Upper panel). Details of each viewing angle are illustrated in Videos 1 and 2. Reconstruction of motor nerve positions by Imaris (see Materials and methods for details) is illustrated in the lower panel. Suprascapular nerve (Ss, yellow); axillary nerve (Ax, purple); musculocutaneous nerve (Mus, green); radial nerve (Rad, blue); posterior brachial cutaneous nerve (PBC, pink). (B ~ D) Higher magnification of MN innervations in the forelimbs of E13.5 control and IG-DMRmatΔ; Hb9::GFP mice. Mutant mice display defects in Ss and Ax axonal branching, concomitant with more PBC axonal branching. Semi-automatic highlighting of the axonal branching and nerve trajectories is used and quantified by Imaris. (E) Quantification of the axonal branching and nerve trajectories for E13.5 control and IG-DMRmatΔ; Hb9::GFP mice by Imaris (see Materials and ethods for details) (n = 6, p<0.01, Mann–Whitney U test).
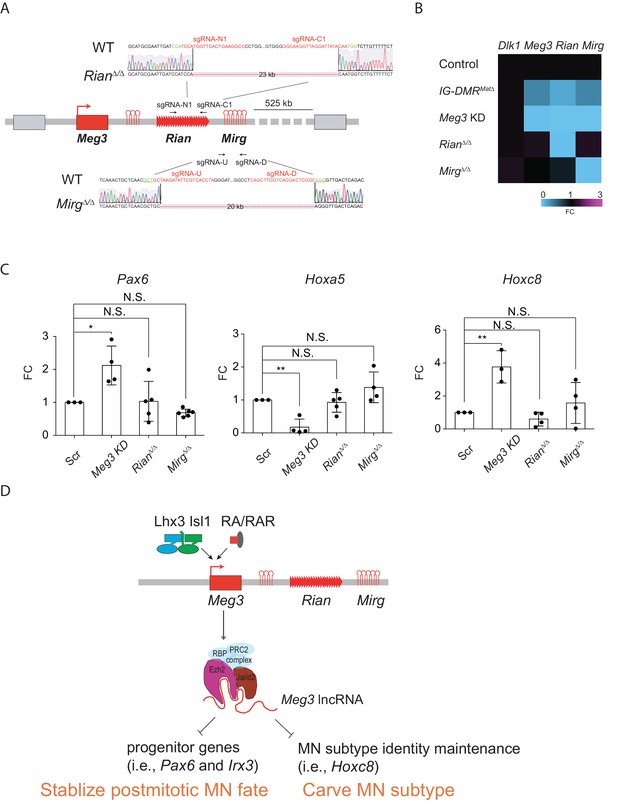
Dissection of individual roles of lncRNAs in the Meg3-Rian-Mirg locus in ESC~MNs
(A) Illustration of the sgRNAs target sites and sequences for the sgRNA:Cas9-mediated Rian and Mirg deletions respectively. Deleted sequences shown in RianΔ/Δ and MirgΔ/Δ ESC lines. The PAM sites are displayed in green; the sgRNA target sequences are reflected in red. (B) Heatmaps representing the abundances of the paternal gene (Dlk1) and maternal lncRNAs (Meg3, Rian, and Mirg) in control, Meg3 KD, and KOs (IG-DMRmatΔ, RianΔ/Δ, and MirgΔ/Δ) ESCs respectively. (C) Rostral Hox gene Hoxa5 is significantly down-regulated in the Meg3 KD line, whereas the expression of Hoxa5 is comparable between RianΔ/Δ and MirgΔ/Δ when compared to the controls. Conversely, the expressions of progenitor gene Pax6, as well as caudal Hox gene Hoxc8, are significantly up-regulated in the Meg3 KD line, while their expressions are restored between RianΔ/Δ and MirgΔ/Δ when compared to the controls. (FC: fold-change; error bars represent SD, n = 3~5, * p-value<0.05, ** p-value<0.01 by Student’s t-test). (D) Summary of the functions of lncRNAs from the imprinted Dlk1-Dio3 locus in ESC~MNs. Meg3 and other lncRNAs from the Dlk1-Dio3 locus are directly activated by MN-TFs (i.e., Lhx3 and Isl1) and RAR, leading to enrichment of Meg3 in the rostral segment of postmitotic MNs. One major function of Meg3 and other lncRNAs from the Dlk1-Dio3 locus is to stimulate Ezh2/Jarid2 interactions. Loss of these lncRNAs compromises the H3K27me3 epigenetic landscape and leads to aberrant expressions of progenitor and caudal Hox genes in postmitotic MNs. Our model illustrates that the lncRNAs of the imprinted Dlk1-Dio3 locus (Meg3 particularly) play a critical role in maintaining postmitotic MN cell fate by repressing progenitor genes, and that they shape MN subtype identity by regulating Hox genes.
Videos
Panoramic views from different angles of forelimb in control Hb9::GFP embryos at E13.5 using light sheet microscopy.
https://doi.org/10.7554/eLife.38080.016Panoramic views from different angles of forelimb in IG-DMRmatΔ ; Hb9::GFP embryos at E13.5 using light sheet microscopy.
https://doi.org/10.7554/eLife.38080.017Tables
Reagent type (species) or resource | Designation | Source or reference | Identifiers | Additional information |
---|---|---|---|---|
Antibody | Rabbit polyclonal anti-Lhx3 | Abcam | Cat# ab14555, RRID:AB_301332 | ICC (1:2000) |
Antibody | Rabbit polyclonal anti-Foxp1 | Abcam | Cat# ab16645, RRID:AB_732428 | ICC (1:20000) |
Antibody | Mouse monoclonal anti-Isl1(2) | DSHB | Cat# 39.4D5, RRID:AB_2314683 | ICC (1:1000) |
Antibody | Rabbit polyclonal anti-Hoxa5 | Sigma-Aldrich | Cat# HPA029319, RRID:AB_10601430 | ICC (1:5000) |
Antibody | Rabbit polyclonal anti-Hoxa5 | Jeremy Dasen (NYU) | ICC (1:16000) | |
Antibody | Guinea pig polyclonal pig anti-Hoxa5 | Jun-An Chen (Academia Sinica) | AB_2744661 | ICC (1:20000) |
Antibody | Mouse monoclonal anti-Hoxc8 | DSHB | Cat# PCRP-HOXC8-1D3, RRID:AB_2618723 | ICC (1:1000) |
Antibody | Rabbit polyclonal anti-Hoxc8 | Sigma-Aldrich | Cat# HPA028911, RRID:AB_10602236 | ICC (1:5000) |
Antibody | Rabbit polyclonal anti-Pea3 | Thomas Jessell (Columbia University) | Cat# C115, RRID:AB_2631446 | ICC (1:2000) |
Antibody | Guinea pig polyclonal anti-Scip | Thomas Jessell (Columbia University) | Cat# CU822, RRID:AB_2631303 | ICC (1:2000) |
Antibody | Sheep polyclonal anti-GFP | AbD Serotec | Cat# 4745–1051, RRID:AB_619712 | ICC (1:1000) |
Antibody | Rabbit polyclonal anti-Irx3 | Thomas Jessell (Columbia University) | ICC (1:16000) | |
Antibody | Rabbit anti-Pax6 | Covance | Cat# PRB-278P, RRID:AB_291612 | ICC (1:300) |
Antibody | Guinea pig polyclonal anti-Hb9 | Hynek Wichterle (Columbia University) | ICC (1:1000) | |
Antibody | Mouse monoclonal anti-Hb9 | DSHB | Cat# 81.5C10, RRID:AB_2145209 | ICC (1:200) |
Antibody | Mouse monoclonal anti-Nkx2.2 | DSHB | Cat# 74.5A5, RRID:AB_53179 | ICC (1:100) |
Antibody | Rabbit polyclonal anti-Olig2 | Millipore | Cat# AB9610, RRID:AB_570666 | ICC (1: 20000) |
Antibody | Goat polyclonal anti-Hoxc6 | Santa Cruz | Cat# sc-46135, RRID:AB_2119751 | ICC (1:1000) |
Antibody | Rabbit polyclonal anti-Hoxc9 | Thomas Jessell (Columbia University) | Cat# Rabbit one anti-HoxC9, RRID:AB_2631312 | ICC (1:1000) |
Antibody | Goat polyclonal anti-Hoxd10 | Santa Cruz | Cat# sc-33005, RRID:AB_648462 | ICC (1:1000) |
Antibody | Mouse monoclonal anti-Ezh2 | Millipore | Cat# 17–662, RRID:AB_1977568 | WB (1:2000); RIP/ChIP/IP (2 μg) |
Antibody | Rabbit polyclonal anti-Jarid2 | Novus | Cat# NB10 0-2214, RRID:AB_10000529 | WB (1:1000); RIP/ChIP (5 μg) |
Antibody | Rabbit polyclonal anti-Suz12 | Abcam | Cat# ab12073, RRID:AB_442939 | WB (1:3000); RIP (2.5 μg) |
Antibody | Mouse monoclonal anti-Histone H3 | Abcam | Cat# ab24834, RRID:AB_470335 | WB (1:1000) |
Antibody | Mouse monoclonal anti-H3K27me3 | Abcam | Cat# ab6002, RRID:AB_305237 | ChIP (2 μg) |
cell line | Mouse: Hb9::GFP ESC | PMID:12176325 | Dr. Hynek Wichterle (Columbia University) | |
Cell line | Mouse: Meg3 KD H6 ESC | this paper | ||
Cell line | Mouse: Meg3 KD K4 ESC | this paper | ||
Cell line | Mouse: Meg3 KD I6 ESC | this paper | ||
Cell line | Mouse: iMeg3v1 ESC | this paper | ||
Cell line | Mouse: iMeg3v5 ESC | this paper | ||
Cell line | Mouse: IG-DMRmatΔ ESC | this paper | ||
Cell line | Mouse: RianΔ/Δ ESC | this paper | ||
Cell line | Mouse: MirgΔ/Δ ESC | this paper | ||
Genetic reagent (M. musculus) | Hb9::GFP | PMID:12176325 | Dr. Hynek Wichterle (Columbia University) | |
Genetic reagent (M. musculus) | IG-DMRmatΔ | PMID:12937418 | Dr. Ann Fergusson Smith (University of Cambridge) | |
Commercial assay or kit | Biotin RNA Labeling Mix | Roche | 11685597910 | |
Commercial assay or kit | Oligo Clean and Concentrator | Zymo Research | D4060 | |
Commercial assay or kit | RNA-Binding Protein Immunoprecipitation Kit | Millipore | 17–700 | |
Commercial assay or kit | TruSeq ChIP Library Preparation Kit | Illumina | IP-202–1012 | |
Software, algorithm | Imaris 8.4.0 | RRID:SCR_007370 |
Additional files
-
Supplementary File 1
Cell type enriched lncRNAs from ESC to ESC~MNs
Values indicate the stage-specific scores (see Materials and methods), 70 stage-signature lncRNAs during the ESC~MN differentiation process are uncovered (ESC, NE, pMN, MN, and IN showed in Figure 1C).
- https://doi.org/10.7554/eLife.38080.024
-
Supplementary File 2
MN-enriched lncRNAs uncovered from RNA-seq.
Only the highly expressed (TMM normalized read count >10 in all samples) lncRNAs are shown.
- https://doi.org/10.7554/eLife.38080.025
-
Transparent reporting form
- https://doi.org/10.7554/eLife.38080.026