RNA-Binding Proteins: A matter of balance
Amyotrophic lateral sclerosis (ALS) is a cruel disease where the death of motor neurons in the brain and spinal cord causes loss of mobility, dexterity, speech, and the ability to swallow. ALS is ultimately fatal, usually within about three years of the onset of symptoms (Taylor et al., 2016). In another neurodegenerative disease, frontotemporal dementia (FTD), neurons from the frontal and temporal lobes of the brain die, leading to a range of cognitive and behavioral symptoms such as personality changes (Ling et al., 2013). The clinical overlap between ALS and FTD has become apparent in recent years: for example, clumps of an RNA-binding protein called TDP-43 build up in almost all cases of ALS and half of FTD cases (Neumann et al., 2006). In 2011, the discovery that a mutation in a gene called C9orf72 is the most common genetic cause of both ALS and FTD confirmed the connection between the diseases (DeJesus-Hernandez et al., 2011; Renton et al., 2011; ).
C9orf72 is a peculiar gene – its name literally stands for the 72nd open reading frame on chromosome 9 – and the disease-causing mutation is even stranger. One of the gene's introns normally contains between two and 23 repeats of a six-nucleotide segment (GGGGCC), but this number can rise to hundreds (or even thousands) in the mutant gene. This increase in repeat numbers could cause disease in several ways: the repeated segments could, for example, be transcribed into RNA molecules that soak up RNA-binding proteins and prevent these proteins from carrying out their functions (Gitler and Tsuiji, 2016).
Researchers have identified RNA-binding proteins that might be disrupted in this way. In a previous eLife paper, researchers at Columbia University reported that one of these proteins, called hnRNP H, attaches to the repeat RNA in C9orf72 and forms clumps within the nucleus. In patients with C9orf72 mutations, this binding hinders how the protein regulates alternative splicing, a mechanism where specific portions of mRNA are edited out or reshuffled (Conlon et al., 2016). Now, a collaboration between the Columbia team and researchers at the New York Genome Center – including Erin Conlon as first author and James Manley as corresponding author – reports a new and unexpected twist to the story (Conlon et al., 2018).
The team hoped to use the splicing defects they identified in the mRNA targets of hnRNP H to create a ‘signature’ that would help them identify which cases are caused by mutations in C9orf72. To begin, they studied 18 of these targets in 50 postmortem samples from the brains of people with ALS, FTD, or both. None of the patients harbored C9orf72 mutations, or any other known mutation. Unexpectedly, the mRNA sequences were still dysregulated in more than half of the samples: if there was no repeat RNA to trap hnRNP H, how could this be the case?
To answer this question, Conlon explored the solubility of hnRNP H in an effort to determine if it was sequestered or somewhat dysfunctional. The samples were split into two groups depending on whether they showed a splicing signature similar to the one present in C9orf72 mutation cases. The team found that hnRNP H was twice as insoluble in the group with the splicing signature compared to the samples without these RNA splicing defects. What drives this insolubility when the mutations in C9orf72 are absent?
Conlon et al. reasoned that hnRNP H could be corrupted by proteins with similar properties that had turned insoluble; and indeed, they discovered three other RNA-binding proteins which had become less soluble. They then turned their attention to one of these proteins, TDP-43, whose loss had been studied before (Polymenidou et al., 2011; Tollervey et al., 2011). This protein was distributed similarly in the brains of all the patients: in other words, histopathology (or looking at the samples under the microscope) was unable to distinguish between the two groups. However, when measuring the mRNA targets of TDP-43, it appeared that TDP-43 had lost much more of its function in the samples with the splicing signature that matches the C9orf72 mutation carriers. The insolubility of TDP-43 (how it behaves biochemically) was therefore more important to identify the two groups.
Collectively, these findings support the idea that the splicing defects found in ALS and FTD appear when any of several RNA-binding proteins becomes insoluble. As such, Conlon et al. infer that we should reconsider how we view these conditions, and move away from the idea that they are simply TDP-43 diseases. Their model predicts the existence of an equilibrium between an array of RNA-binding proteins, each at a different concentration and in a physically distinct state. Even subtle mutations could change the concentration and the solubility of any of these proteins, which could tip the scale and culminate in various defects in RNA processing. Therapeutic interventions that reestablish the RNA-binding protein balance could significantly slow or stop the course of the diseases.
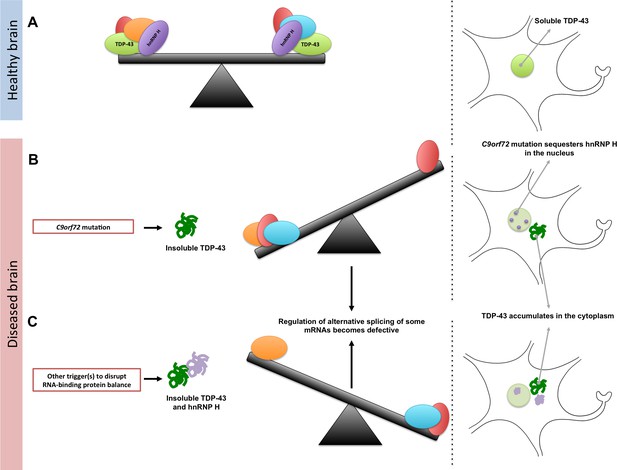
Maintaining the balance of RNA-binding proteins.
(A) RNA-binding proteins (red, green, orange, purple, and blue), such as TDP-43 (green), regulate various RNA processing pathways, like the alternative splicing of mRNA. Many of these proteins are also prone to aggregating and becoming insoluble. As represented by the seesaw, this means that their concentration, solubility, and interactions with proteins must be controlled to maintain balance in the cell (right). Under normal conditions, TDP-43 is soluble and is mainly localized in the nucleus of the cell. (B) A mutation in a gene called C9orf72 can result in a reduction in the solubility of an RNA-binding protein known as hnRNP H (purple). This can also lead to changes in the concentration and solubility of other RNA-binding proteins (including TDP-43), which disrupts alternative splicing and other processes in the cell. The reduction in the solubility of TDP-43 causes it to accumulate in the cytoplasm, which is a hallmark of ALS and FTD. (C) Other triggers, such as genetic and environmental factors, can change the concentration and solubility of RNA-binding proteins (unbalanced seesaw); this causes them to misfold, become insoluble and accumulate in the cytoplasm.
References
-
Characterizing the RNA targets and position-dependent splicing regulation by TDP-43Nature Neuroscience 14:452–458.https://doi.org/10.1038/nn.2778
Article and author information
Author details
Publication history
Copyright
© 2018, Gitler et al.
This article is distributed under the terms of the Creative Commons Attribution License, which permits unrestricted use and redistribution provided that the original author and source are credited.
Metrics
-
- 2,673
- views
-
- 331
- downloads
-
- 5
- citations
Views, downloads and citations are aggregated across all versions of this paper published by eLife.
Download links
Downloads (link to download the article as PDF)
Open citations (links to open the citations from this article in various online reference manager services)
Cite this article (links to download the citations from this article in formats compatible with various reference manager tools)
Further reading
-
- Biochemistry and Chemical Biology
- Genetics and Genomics
Yerba mate (YM, Ilex paraguariensis) is an economically important crop marketed for the elaboration of mate, the third-most widely consumed caffeine-containing infusion worldwide. Here, we report the first genome assembly of this species, which has a total length of 1.06 Gb and contains 53,390 protein-coding genes. Comparative analyses revealed that the large YM genome size is partly due to a whole-genome duplication (Ip-α) during the early evolutionary history of Ilex, in addition to the hexaploidization event (γ) shared by core eudicots. Characterization of the genome allowed us to clone the genes encoding methyltransferase enzymes that catalyse multiple reactions required for caffeine production. To our surprise, this species has converged upon a different biochemical pathway compared to that of coffee and tea. In order to gain insight into the structural basis for the convergent enzyme activities, we obtained a crystal structure for the terminal enzyme in the pathway that forms caffeine. The structure reveals that convergent solutions have evolved for substrate positioning because different amino acid residues facilitate a different substrate orientation such that efficient methylation occurs in the independently evolved enzymes in YM and coffee. While our results show phylogenomic constraint limits the genes coopted for convergence of caffeine biosynthesis, the X-ray diffraction data suggest structural constraints are minimal for the convergent evolution of individual reactions.
-
- Biochemistry and Chemical Biology
- Structural Biology and Molecular Biophysics
The SARS-CoV-2 main protease (Mpro or Nsp5) is critical for production of viral proteins during infection and, like many viral proteases, also targets host proteins to subvert their cellular functions. Here, we show that the human tRNA methyltransferase TRMT1 is recognized and cleaved by SARS-CoV-2 Mpro. TRMT1 installs the N2,N2-dimethylguanosine (m2,2G) modification on mammalian tRNAs, which promotes cellular protein synthesis and redox homeostasis. We find that Mpro can cleave endogenous TRMT1 in human cell lysate, resulting in removal of the TRMT1 zinc finger domain. Evolutionary analysis shows the TRMT1 cleavage site is highly conserved in mammals, except in Muroidea, where TRMT1 is likely resistant to cleavage. TRMT1 proteolysis results in reduced tRNA binding and elimination of tRNA methyltransferase activity. We also determined the structure of an Mpro-TRMT1 peptide complex that shows how TRMT1 engages the Mpro active site in an uncommon substrate binding conformation. Finally, enzymology and molecular dynamics simulations indicate that kinetic discrimination occurs during a later step of Mpro-mediated proteolysis following substrate binding. Together, these data provide new insights into substrate recognition by SARS-CoV-2 Mpro that could help guide future antiviral therapeutic development and show how proteolysis of TRMT1 during SARS-CoV-2 infection impairs both TRMT1 tRNA binding and tRNA modification activity to disrupt host translation and potentially impact COVID-19 pathogenesis or phenotypes.