TAPBPR mediates peptide dissociation from MHC class I using a leucine lever
Figures
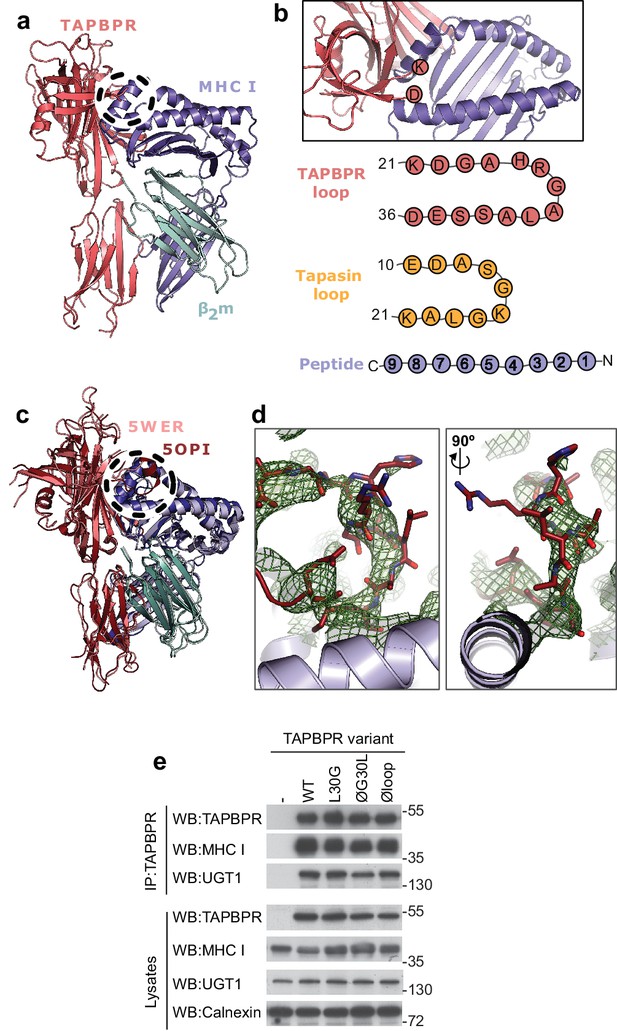
TAPBPR loop interactions with MHC I.
(a) Model of TAPBPR (pink) docked onto MHC I (blue) and β2m (cyan) based on interaction studies (Hermann et al., 2013). (b) Top panel, illustration of the proximity of the TAPBPR loop region to the peptide binding groove (viewed from the top of complex shown in panel a). Lower panel, schematic diagrams of the amino acid composition of the TAPBPR and tapasin loops compared to the length and orientation of a peptide. (c) Overlay of two recent X-ray structures of TAPBPR in complex with MHC I (Jiang et al., 2017; Thomas and Tampé, 2017) (PDB ID 5WER and 5OPI) oriented and coloured to illustrate the similarity to our TAPBPR:MHC I complex (panel a). The position of the TAPBPR loop is circled (black dashed line). (d) The electron density map (2Fo-Fc, green mesh) and the built model (maroon sticks, residues D23-E34) are shown for the loop region of TAPBPR (PDB ID 5OPI). Two views of the loop and density are shown rotated by 90 degrees. (e) Expression of TAPBPR loop variants in IFNγ treated HeLaM-TAPBPRKO and their interaction with MHC I and UGT1. Western blotting for calnexin is included as a loading control. Representative of three independent experiments.
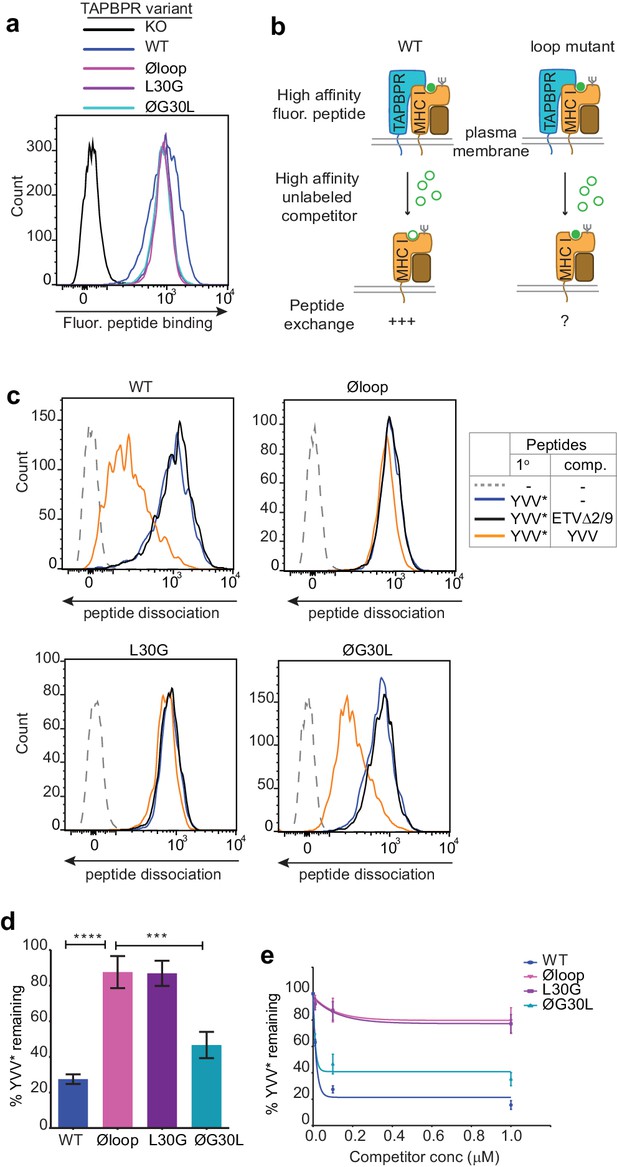
The TAPBPR K22-D35 loop is critical for peptide exchange.
(a) Typical peptide binding when cells gated for expressing high levels of surface TAPBPR were incubated with 10 nM YVVPFVAK*V peptide for 15 min at 37°C on IFNγ treated HeLaM-TAPBPRKO cells -/+expression of TAPBPRWT, TAPBPRØloop, TAPBPRL30G or TAPBPRØG30L. (b) Schematic representation of the experimental workflow used to compare the efficiency of peptide exchange by plasma membrane bound TAPBPRWT with the plasma membrane bound TAPBPR loop mutants. (c) Histograms show the level of dissociation of YVVPFVAK*V (YVV*) in the absence (blue line) and presence of 100 nM non-labelled competitor peptide YVVPFVAKV (YVV)(orange line) or EGVSKQSNG (ETVΔ2/9), a peptide in which the anchors which permit HLA-A*68:02 binding are mutated to produce a non-binding derivative (black line). Similar patterns of dissociation were found on cells incubated at 4°C demonstrating that the peptide exchange occurs directly on the cell surface (see Figure 2—figure supplement 1). (d-e) Graphs show the percentage of fluorescent peptide YVVPFVAK*V (YVV*) remaining in the presence of (d) 100 nM or (e) increasing concentrations of the non-labelled competitor peptide YVVPFVAKV as a percentage of the bound YVVPFVAK*V observed in the absence of competitor peptide from four independent experiments. Error bars show -/+SD.****p≤0.0001, ***p≤0.001 using unpaired two-tailed t-tests.
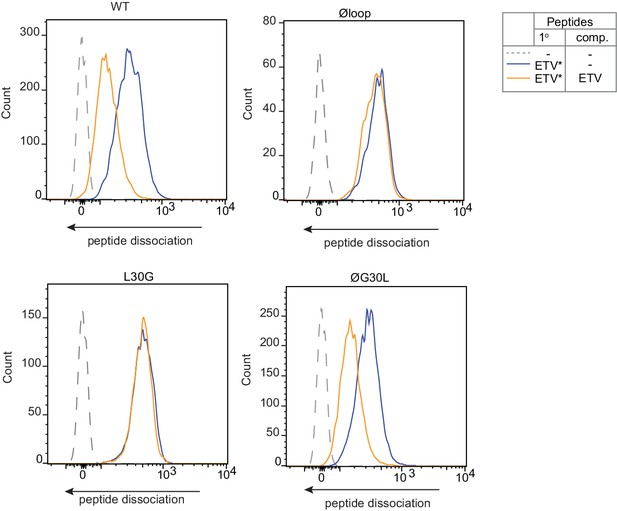
Peptide Exchange at 4°C.
To ensure peptide exchange was occurring directly on the surface MHC I rather than in intracellular vesicles, peptide exchange assays were performed on cells incubated at 4°C to inhibit membrane trafficking. HeLa-TAPBPRKO cells reconstituted with the panel of TAPBPR loop mutant were cooled to 4°C then incubated with 5 µM ETVSK*QSNV (ETV*) for 60 min at 4°C, followed by washing thoroughly in cold PBS to remove unbound peptide. Dissociation of the fluorescent peptide was subsequently monitored in the absence (blue line) or presence of 1 µM ETVSEQSNV (ETV) (orange line) for 60 min at 4°C.
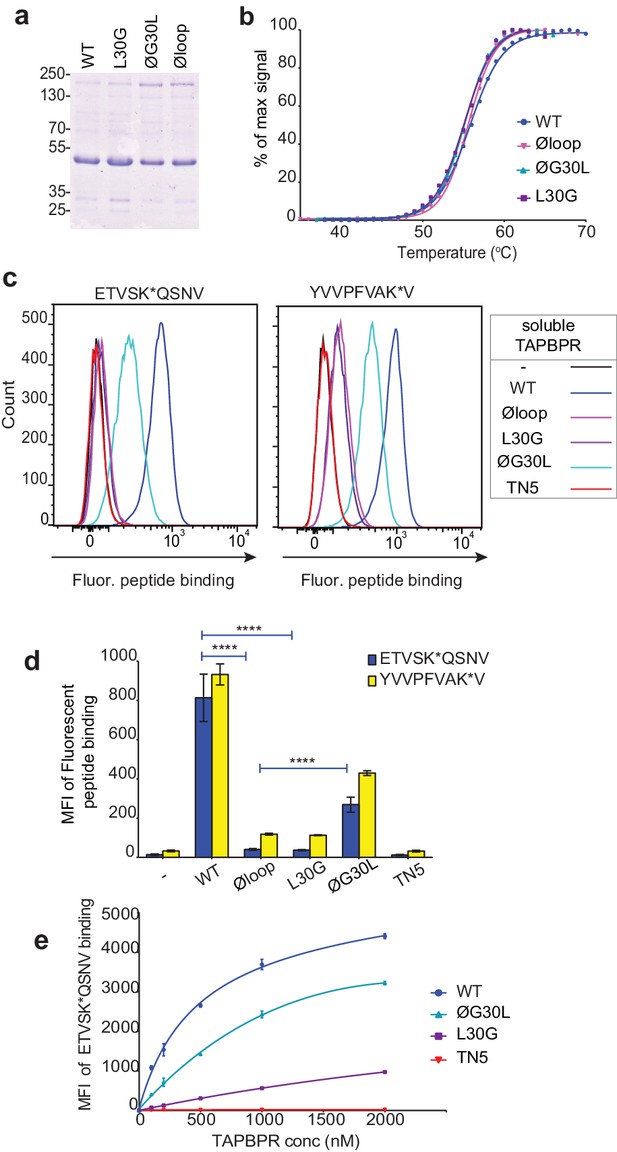
Soluble TAPBPR loop variants exhibit reduced ability to mediate peptide exchange on surface HLA-A*68:02 molecules.
(a) Expression and purity of soluble forms of WT, L30G, ØG30L, and Øloop TAPBPR variants after their purification from the culture supernatant using Ni-affinity. (b) Differential scanning fluorimetry demonstrates the three TAPBPR loop mutants have equivalent thermal denaturation profiles as TAPBPRWT. (c) Histograms of the typical fluorescent peptide binding to IFNγ-treated HeLaM cells incubated -/+100 nM exogenous soluble TAPBPR variant for 15 min at 37°C, followed by incubation with 10 nM ETVSK*QSNV or YVVPFVAK*V for an additional 15 min. TAPBPRTN5, in which isoleucine at position 261 is mutated to lysine, to produce a TAPBPR variant which cannot bind to MHC I, is included as a control (d) Bar graphs show the reproducibility of results in (c). (e) Dose response curves of fluorescent peptide binding to IFN-γ treated HeLaM cells incubated with increasing concentrations of the soluble TAPBPR variants prior to the addition of 10 nM ETVSK*QSNV. Error bars represent MFI -/+SD from four independent experiments. ****p≤0.0001 using unpaired two-tailed t-tests.
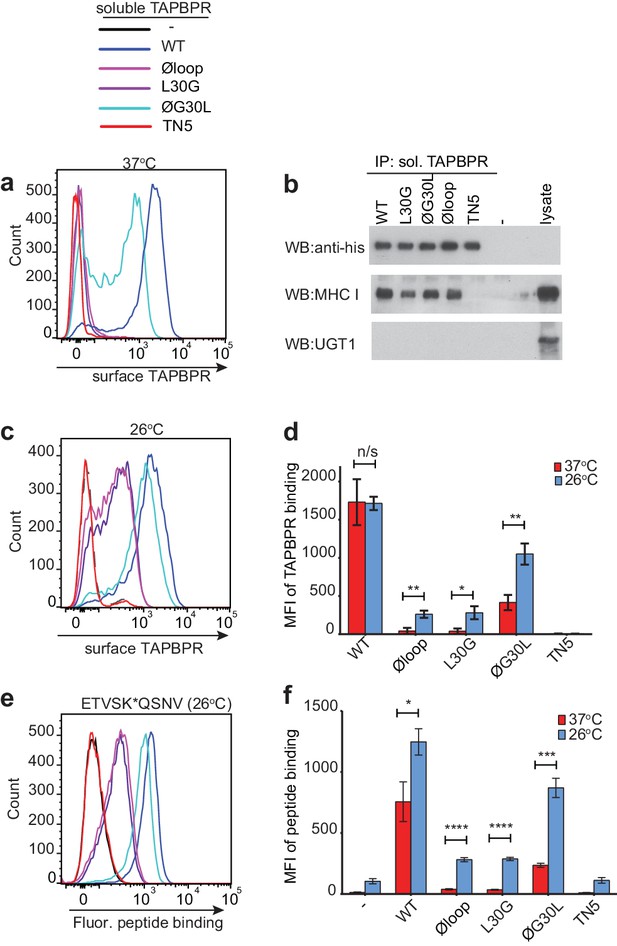
Residues K22-D35 are essential for soluble TAPBPR to bind peptide-loaded MHC I.
(a and c) Histograms of soluble TAPBPR loop variant binding to HeLaM-HLA-ABCKO cells expressing HLA-A*68:02 incubated with 100 nM TAPBPR at (a) 37°C or (c) 26°C for 30 min. TAPBPRTN5, a TAPBPR variant which cannot bind to MHC I, is included as a negative control. (b) TAPBPR pull-downs on IFNγ-treated HeLaM-TAPBPRKO cells incubated with soluble TAPBPR loop mutants reveal all variants are capable of binding to MHC I, but do not bind to UGT1. TAPBPRTN5 is included as a non-MHC binding control. Data is representative of three independent experiments. (d) Bar graph comparing soluble TAPBPR variant binding to HeLaM-HLA-ABCKO+A*68:02 cells at 37°C with 26°C from three independent experiments. Error bars represent -/+SD. (e) Histograms show typical fluorescent peptide binding to IFNγ induced HeLaM cells treated -/+100 nM soluble TAPBPR variants for 15 min at 26°C, followed by incubation with 10 nM ETVSK*QSNV for 15 min at 26°C. (f) Bar graph compares ETVSK*QSNV peptide binding to HeLaM cells treated -/+ soluble TAPBPR variants at 37°C with 26°C from three independent experiments. Error bars represent -/+SD. n/s = not significant, *p≤0.05, **p≤0.01, ***p≤0.001, ****p≤0.0001, using unpaired two-tailed t-tests.
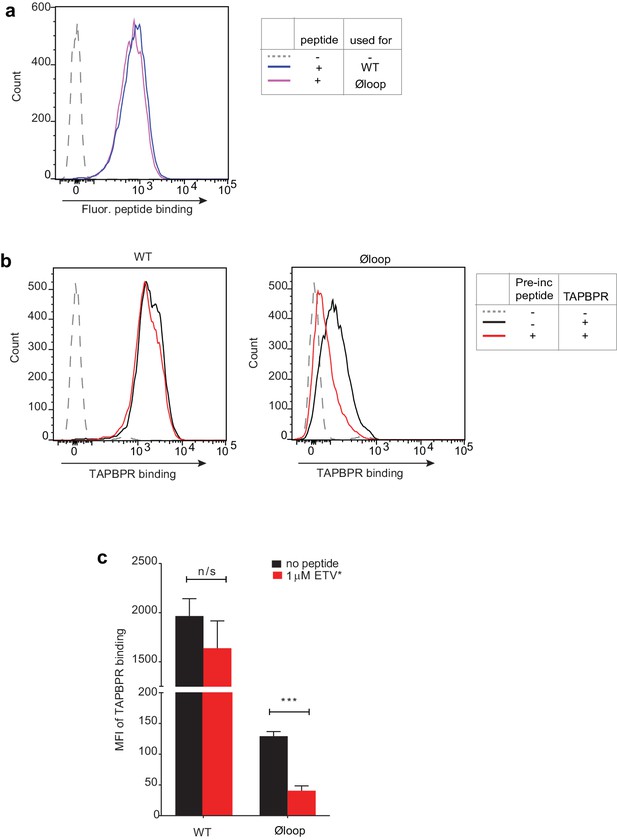
Pre-incubation with high affinity peptide inhibits TAPBPRØloop binding to HLA-A*68:02 molecules at 26°C.
HeLaM-HLA-ABCKO cells reconstituted with HLA-A*68:02 were incubated at 26°C for 12 hr, then subsequently treated -/+1 µM ETVSK*QSNV peptide for 30 min at 26°C, followed by washing twice in PBS to remove unbound peptide. Cells were then incubated cells with -/+100 nM TAPBPRWT or TAPBPRØloop at 26°C for 15 min, washed in PBS, then cooled to 4°C for detection of bound TAPBPR using the mAb PeTe4. (a) Histograms show the fluorescent peptide binding to the cells incubated at 26°C used to test soluble TAPBPRWT and TAPBPRØloop binding. (b) Histograms show the typical TAPBPRWT and TAPBPRØloop binding to cells incubated at 26°C with (red line) and without (black line) pre-incubation with the HLA-A*68:02-binding peptide. (c) Bar chart show the MFI of TAPBPR binding from three independent experiments. Error bars represent -/+SD. The data show that pre-incubation of cells with high-affinity peptide significantly reduces the ability of soluble TAPBPRØloop to bind to HLA-A*68:02 (***p≤0.001) but does not significantly inhibit the ability of TAPBPRWT to bind to HLA-A*68:02.
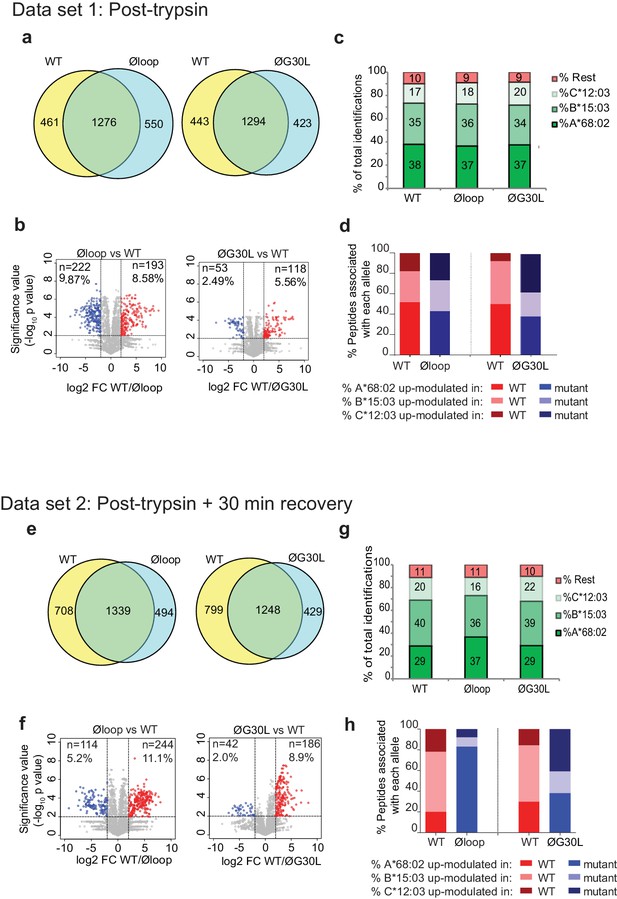
Mutation of the K22-D35 loop of TAPBPR changes the peptide repertoire presented on cells.
Peptides eluted from W6/32-reactive MHC I complex isolated from IFNγ treated HeLaM-TAPBPRKO expressing either TAPBPRWT, TAPBPRØloop or TAPBPRØG30L were analysed using LC-MS/MS. In dataset 1 (a–d), cells were frozen immediately post-trypsination while in dataset 2 (e–h) cells were allowed to recover in media for 30 min after trypsination, before freezing. The sequences of identified peptides are listed in Figure 5—source datas 2–7. The comparison of all five technical replicates for the two datasets is shown in Figure 5—figure supplement 1. (a,e) Venn diagrams compare all the identified peptides using a presence/absence approach. (b,f) Volcano plots graphically summarise label-free quantitation, displaying modulated peptides between two cells lines. Colour circles highlight the peptide which are differentially expressed between two cell lines after applying an adjusted p-value of <0.01. The list of these peptides is available in Figure 5—source datas 8 and 9. n = number of significantly modulated peptides, % demonstrates the fraction of significantly modulated peptides in a specific cell line compare to all peptides in the comparison. (c,d,g,h) Bar graphs summarise the MHC I molecules (HLA-A*68:02, -B*15:03 or –C*12:03) that the (c,g) identified peptides in a/e, and (d,h) the significantly modulated peptides identified in b/f were matched to using the NetMHCpan-4.0. In (c) and (g), peptides not successfully assigned are indicated in orange (rest). Analysis of the peptide repertoire from a further TAPBPR-loop mutant lacking L30 and from a third biological repeat can be found in Figure 5—figure supplements 2 and 3 respectively. Analysis of the predicted affinity of peptides differential modulated upon mutation of the loop (i.e those in b) and (f) can be found in Figure 5—figure supplement 4.
-
Figure 5—source data 1
Binding of TAPBPR to the individual HLA molecules found in HeLaM cells.
Soluble TAPBPRWT or soluble TAPBPRØloop were incubated with the indicated LABScreen single antigen HLA class I beads (from One Lambda, Canoga Park, California) for 60 min at RT. After washing 3 times in PBS, bound TAPBPR was detected using the TAPBPR-specific mAb PeTe4 and a goat anti-mouse PE-conjugated secondary antibody. Samples were analysed using the Luminex Fluoroanalyser system. The table shows the MFI of PeTe4 staining ± SD for the indicated HLA beads incubated in the absence and presence of 1 µM of TAPBPRWT or TAPBPRØloop. The expression of MHC I (detected using W6/32) provided by One Lambda for the specific lot of LABScreen beads used is included as a control.
- https://doi.org/10.7554/eLife.40126.014
-
Figure 5—source data 2
Dataset 1 - peptides eluted from W6/32-reactive MHC I complexes from IFNγ treated HeLaM-TAPBPRKO cells expressing TAPBPRWT.
- https://doi.org/10.7554/eLife.40126.015
-
Figure 5—source data 3
Dataset 1 - peptides eluted from W6/32-reactive MHC I complexes from IFNγ treated HeLaM-TAPBPRKO cells expressing TAPBPRØloop.
- https://doi.org/10.7554/eLife.40126.016
-
Figure 5—source data 4
Dataset 1 - peptides eluted from W6/32-reactive MHC I complexes from IFNγ treated HeLaM-TAPBPRKO cells expressing TAPBPRØG30L.
- https://doi.org/10.7554/eLife.40126.017
-
Figure 5—source data 5
Dataset 2 - peptides eluted from W6/32-reactive MHC I complexes from IFNγ treated HeLaM-TAPBPRKO cells expressing TAPBPRWT.
- https://doi.org/10.7554/eLife.40126.018
-
Figure 5—source data 6
Dataset 2 - peptides eluted frm W6/32-reactive MHC I complexes from IFNγ treated HeLaM-TAPBPRKO cells expressing TAPBPRØloop.
- https://doi.org/10.7554/eLife.40126.019
-
Figure 5—source data 7
Dataset 2 - peptides eluted from W6/32-reactive MHC I complexes from IFNγ treated HeLaM-TAPBPRKO cells expressing TAPBPRØG30L.
- https://doi.org/10.7554/eLife.40126.020
-
Figure 5—source data 8
Dataset 1 - analysis of eluted peptides used to generate volcano plots.
- https://doi.org/10.7554/eLife.40126.021
-
Figure 5—source data 9
Dataset 2 - analysis of eluted peptides used to generate volcano plots.
- https://doi.org/10.7554/eLife.40126.022
-
Figure 5—source data 10
Peptides eluted from W6/32-reactive MHC I complexes from IFNγ treated HeLaM-TAPBPRKO cells expressing TAPBPRM29.
- https://doi.org/10.7554/eLife.40126.023
-
Figure 5—source data 11
Dataset 3 - peptide list for third biological repeat for TAPBPRWT expressing cells.
- https://doi.org/10.7554/eLife.40126.024
-
Figure 5—source data 12
Dataset 3 - peptide list for third biological repeat for TAPBPRØloop expressing cells.
- https://doi.org/10.7554/eLife.40126.025
-
Figure 5—source data 13
Dataset 3 - peptides list for third biological repeat for TAPBPRØG30L expressing cells.
- https://doi.org/10.7554/eLife.40126.026
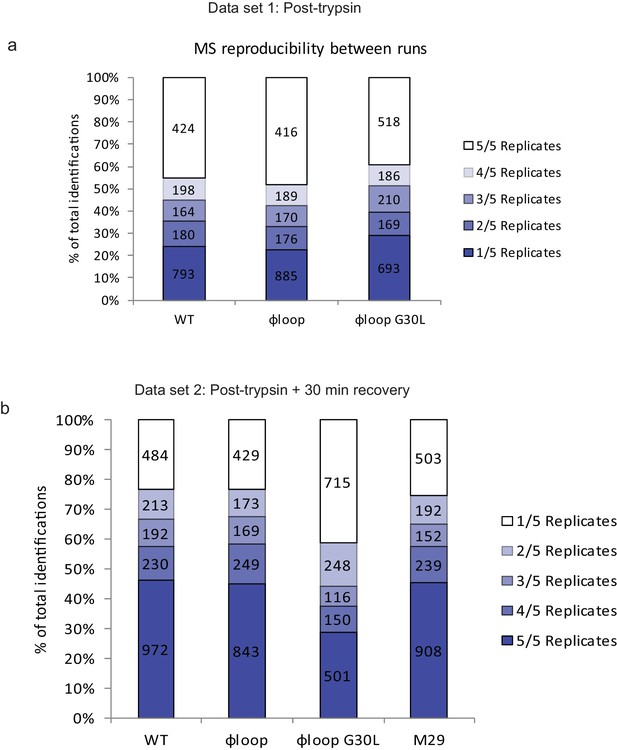
Technical reproducibility of LC-MS/MS measurement.
Peptide elution experiments were performed in five technical replicates for all cell lines. Column analysis reveals the percentage of peptides identified in 1–5 out of 5 technical replicates for (a) dataset 1 (cells frozen immediately post-trypsinisation) and (b) dataset 2 (cells allowed to recover for 30 min in media post-trypsinisation before freezing).
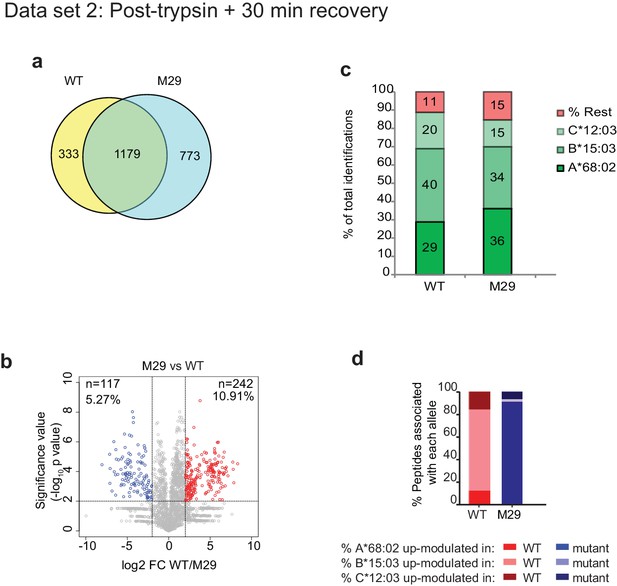
Mutation of residue A29-D35 in the loop of TAPBPR changes the peptide repertoire presented on cells.
In addition to the TAPBPRWT, TAPBPRØloop or TAPBPRØG30L analysed in Figure 5e–h, we also performed peptidomic analysis for an additional loop mutation of TAPBPR in which residues A29-D35 where mutated from ALASSED to GGSGGAA (TAPBPRM29). Thus, this mutant also lacks the leucine 30 residue. Peptides eluted from W6/32-reactive MHC I complex isolated from IFNγ-treated HeLaM-TAPBPRKO expressing either TAPBPRWT and TAPBPRM29 were analysed using LC-MS/MS as in dataset two in Figure 5 (with recovery in media for 30 min after trypsination, before freezing). The sequences of identified peptides for TAPBPRM29 are listed in Figure 5—source data 10. (a) Venn diagrams compare all the identified peptides using a presence/absence approach. (b) Volcano plots graphically summarise label-free quantitation, displaying modulated peptides between two cells lines. Colour circles highlight the peptide which are differentially expressed between two cell lines after applying an adjusted p-value of <0.01. n = number of significantly modulated peptides, % demonstrates the fraction of significantly modulated peptides in a specific cell line compare to all peptides in the comparison. (c) Bar graphs summarise the MHC I molecules (HLA-A*68:02, -B*15:03 or –C*12:03) that the (c) identified peptides in (a) and (d) the significantly modulated peptides identified in (b) and were matched to using the NetMHCpan-4.0. In c, peptides not successfully assigned are indicated in orange (rest).
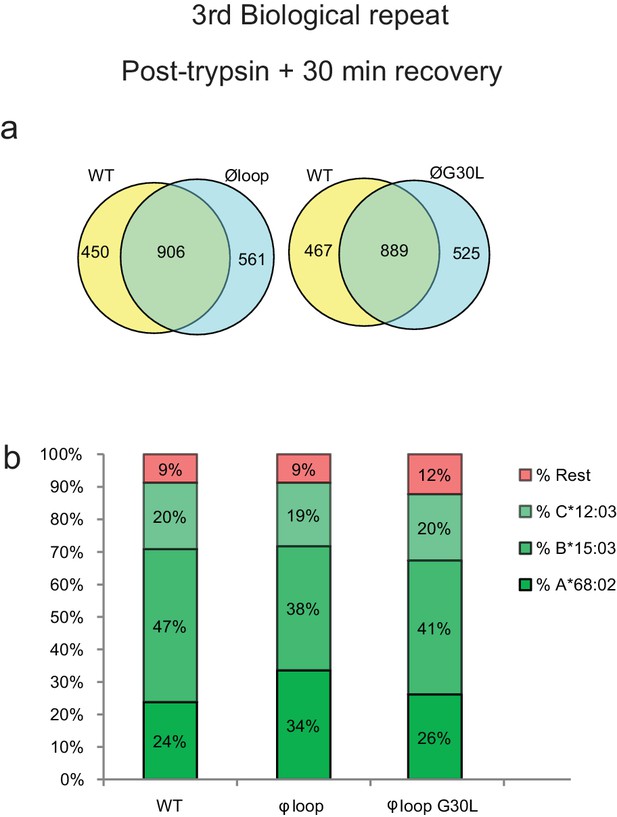
3rd biological repeat.
Peptides were eluted from W6/32-reactive MHC I complexes isolated from a third set of IFNγ treated HeLaM-TAPBPRKO expressing either TAPBPRWT, TAPBPRØloop or TAPBPRØG30L and analysed using LC-MS/MS. The treatment of these cells is comparable to dataset two in the main Figure 5 (cells were allowed to recover in media for 30 min after trypsination, before freezing. The sequences of identified peptides are listed in Figure 5—source datas 11–13. The dataset is based on three technical repeats. (a) Venn diagrams compare all the identified peptides using a presence/absence approach. (b) Bar graphs summarise the MHC I molecules (HLA-A*68:02, -B*15:03 or –C*12:03) that the identified peptides in (a) were matched to using the NetMHCpan-4.0. In (b), peptides not successfully assigned are indicated in orange (rest). As shown in Figure 5e and g, this additional dataset suggests that TAPBPR molecules with a function loop strips peptides from HLA-A*68:02 molecules.
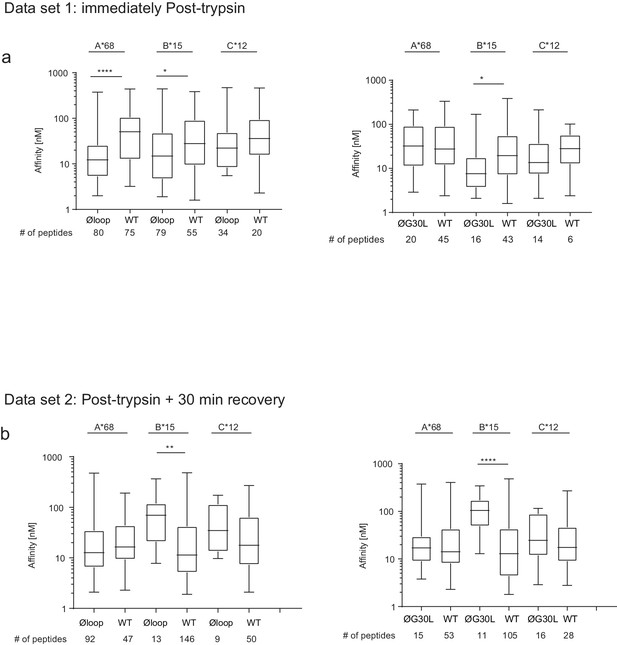
Peptide affinity predictions using NetMHC.
The affinity of peptides identified as being differentially modulated in the Volcano plots in
Figure 5b and f) were predicted using netMHCpan3.0. For each comparisons the peptides were sorted into the A-/B-/C-alleles using best_NetMHC_Allele. Peptides with an affinity above 500 nM were excluded. Box and whiskers plots show the peptide affinities for HLA-A*68:02, -B*15:03 and C*12:03 for peptides differentially modulated in (a) dataset one and (b) dataset 2. As the data-points were not normally distributed, the Mann-Whitney test was used to find significant differences between the comparisons. The number of peptides used in the analysis is show below each graph. We feel it is very difficult to draw any definitive conclusions regarding the effect of mutating the loop on the affinity of the peptides bound to MHC I from this particular analysis. Firstly, the peptide numbers corresponding to each HLA is low, especially for the HLA-B15 assigned peptides from cells expressing the TAPBPR loop mutant. This questions whether the significance observed is reliable, particularly for HLA-B15. Secondly, our experimental design was set up to explore whether there was a global change in peptide repertoire upon mutation of the TAPBPR loop. Thus, technical limitations regarding the need to assign peptides to particular MHC I (by applying thresholds or cut-offs to determine which peptide was bound to which MHC I) greatly reduce the ability to detect peptides exhibiting low affinity for a particular MHC I. Other studies (Garstka et al., 2015; Kanaseki et al., 2013; Nagarajan et al., 2016), exploring the affinity of peptides by immunopeptidomics use cells expressing only one MHC I. Therefore, the identified peptides do not need to be assigned to MHC I, thus improving the ability to see reliable changes (including subtle changes) in anchors and affinity. A third consideration is that trypsination of cells may be cause the loss of low-affinity peptide from MHC I molecules. One interesting observation from this analysis was the affinity of HLA-A*68:02 assigned peptides between dataset 1 and dataset two for cells expressing TAPBPRWT appeared to increase. Thus hinting that surface expressed TAPBPRWT may dissociate low-affinity peptide from HLA-A*68:02. However, to conclude, from these particular datasets, we are unable to address whether there is any change in affinity of MHC I bound peptides upon mutation of the TAPBPR loop. Improvement in experimental design will address this in the future.
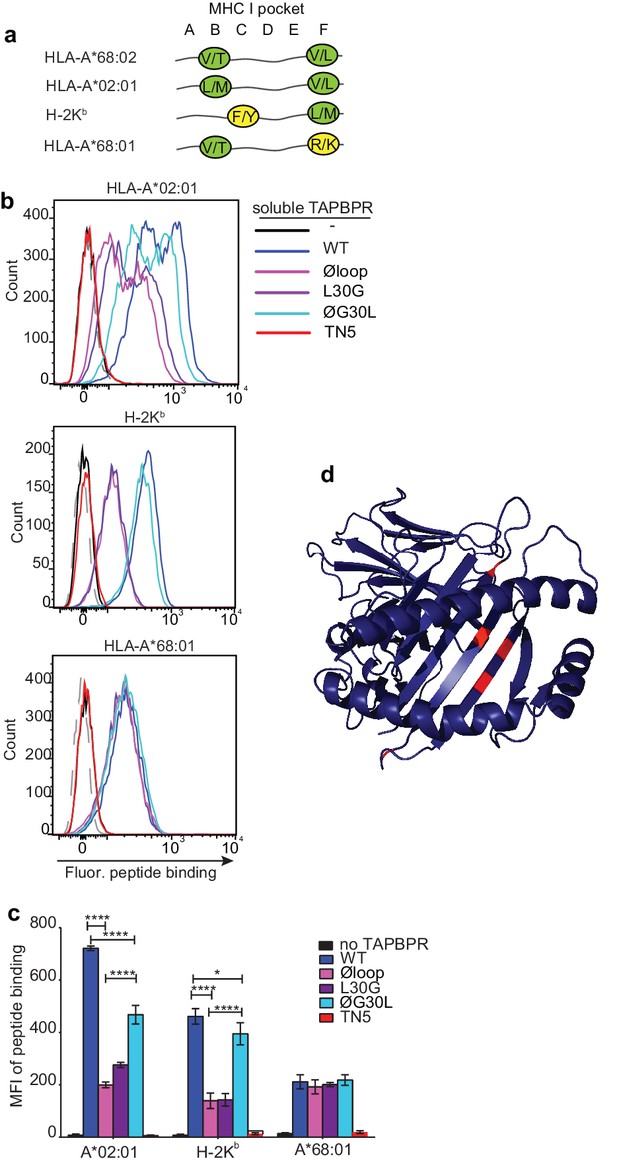
F pocket specificity for hydrophobic residues influences the ability of TAPBPR to edit peptides in a loop-dependent manner.
(a) Comparison of the A-F pocket specificities of the HLA-A*68:02, HLA-A*02:01, H-2Kb and HLA-A*68:01 peptide binding grooves. (b) Binding of fluorescent peptide to IFNγ-treated HeLaM-HLA-ABCKO transduced with HLA-A*02:01, mouse EL4 cells (which express H-2Kb) or HeLaM-HLA-ABCKO transduced with HLA-A*68:01 -/+1 µM soluble TAPBPR variant for 15 min at 37°C, followed by incubation with 10 nM NLVPK*VATV (HLA-A2 binding peptide) for 60 min, 1 nM SIINFEK*L (H-2Kb binding peptide) for 30 min or 100 nM KTGGPIYK*R (HLA-A*68:01) for 60 min at 37°C. (c) Bar graph summarising the peptide exchange by soluble TAPBPR variants as performed in b). Error bars represent MFI -/+ SD from four independent experiments. ****p ≤ 0.0001, ***p ≤ 0.001, *p ≤ 0.05, using unpaired two-tailed t-tests. (d) Structure of MHC I from above the binding groove and the different amino acids between HLA-A*68:02 and –A*68:01 highlighted in red.
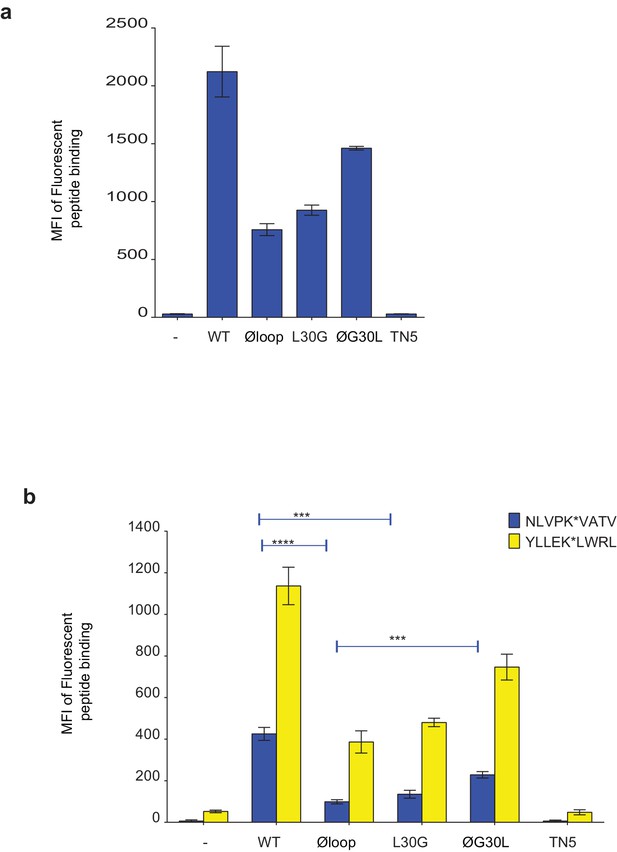
Peptide loading by soluble TAPBPR onto HLA-A2 molecules.
(a) Fluorescent peptide binding to IFNγ treated HeLaM-HLA-ABCKO reconstituted with HLA-A2 treated -/+1 µM soluble TAPBPR variant for 15 min at 37°C, followed by incubation with 10 nM YLLEK*LWRL (HLA-A2 binding peptide) for 60 min at 37°C. (b) Fluorescent peptide binding to IFNγ-treated MCF-7 treated -/+1 µM soluble TAPBPR variant for 15 min at 37°C, followed by incubation with 10 nM NLVPK*VATV or YLLEK*LWRL (HLA-A2 binding peptides) for 60 min at 37°C. Error bars represent MFI -/+SD from four independent experiments. ****p≤0.0001, ***p≤0.001 using unpaired two-tailed t-tests.
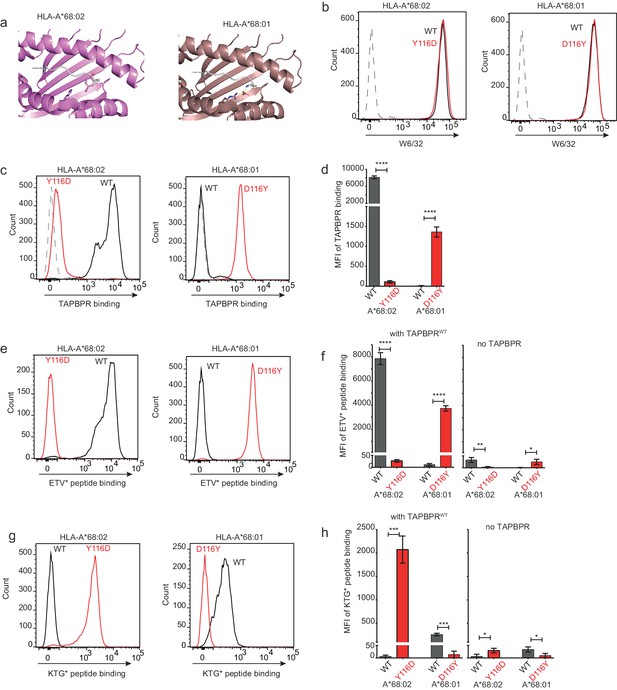
Mutation of the MHC I F pocket alters TAPBPR-mediated peptide editing.
(a) PyMOL images of the binding grooves of HLA-A*68:02 (PDB ID 4HX1) and -A*68:01 (PDB ID 4HWZ), with residues found at position 116 and 114 highlighted. (b) Histograms show the surface expression of HLA-A*68:02WT, -A*68:02Y116D, -A*68:01WT and -A*68:01D116Y, detected using W6/32, when expressed in HeLa-M-HLA-ABCKO cells. (c, e, g) HeLaM-HLA-ABCKO cells expressing the panel of HLA-A*68 molecules were incubated with 1 μM of soluble TAPBPR for 15 min at 37°C, followed by either (c) detection of surface bound TAPBPR using PeTe4, or incubation with (e) 10 nM ETVSK*QSNV (ETV*) or (g) 100 nM KTGGPIYK*R (KTG*) peptide for 15 min and 60 min, respectively. In (c), staining with an isotype control antibody is included as a control (grey dotted line). While histograms in c, e, g are representative images, the bar graphs in d, f, h summarise the MFI of (d) TAPBPR binding and (f and h) fluorescent peptide binding in the presence and absence of TAPBPR, from three independent experiment ± SD. ****p ≤ 0.0001, ***p ≤ 0.001, P** ≤ 0.01, *p ≤ 0.05.
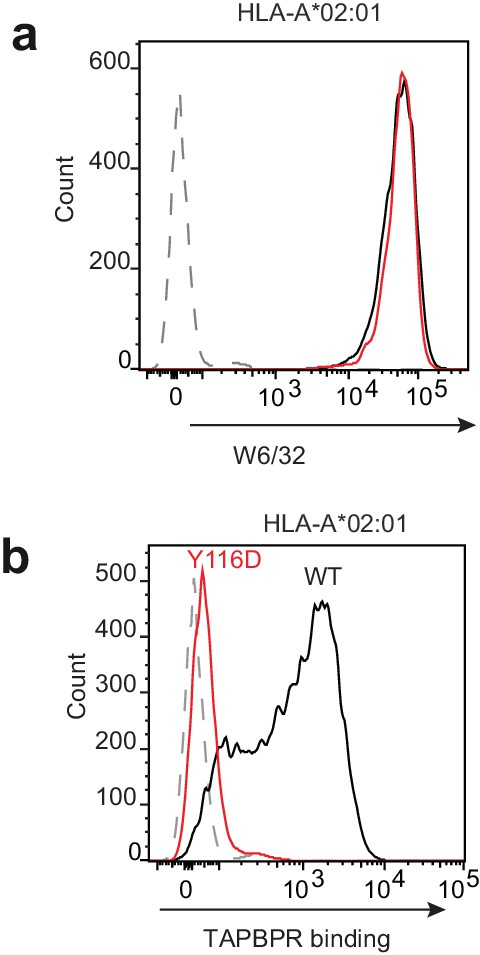
Mutation of the HLA-A2 F pocket alters TAPBPR binding.
https://doi.org/10.7554/eLife.40126.030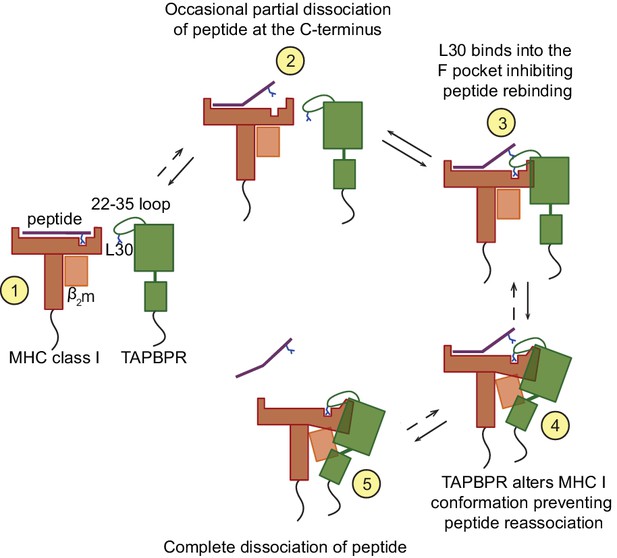
Proposed model of the peptide exchange mechanism of TAPBPR on MHC class I.
https://doi.org/10.7554/eLife.40126.031Tables
Panel of TAPBPR loop mutants.
Residues altered are highlighted in red.
TAPBPR variant | Loop sequence |
---|---|
WT | KDGAHRGALASSED |
Øloop | |
L30G | KDGAHRGA | ASSED
ØG30L | L |
Primers used for cloning and generation of TAPBPR loop mutants.
https://doi.org/10.7554/eLife.40126.032Primer name | Primer sequence 5ʹ−3ʹ |
---|---|
TAPBPRWT- BamHI-for | GCGCGGATCCAGCAGCCTCCATGGGCACACAGGAGGGC |
TAPBPRWT- NotI-rev | GCGCGCGGCCGCTCAGCTGGGCTGGCTTACA |
M22-for | GTCCTAGACTGTTTCCTGGTGGCGGCCGGTGGGAGCGGTGGAGCTCTCGCCAGCAGTG |
M22-rev | CACTGCTGGCGAGAGCTCCACCGCTCCCACCGGCCGCCACCAGGAAACAGTCTAGGAC |
M29-for | GCGGCCGGTGGGAGCGGTGGAGGTGGCAGCGGCGGTG |
M29-rev | TCCACCGCTCCCACCGGCCGCCACCAGGAAACAGTCTAGGAC |
L30G-for | GTGGAGCTGGCGCCAGCAGT |
L30G-rev | ACTGCTGGCGCCAGCTCCAC |
ØG30L-for | GGTGGAGGTCTGGGCGGCGGTGC |
ØG30L-rev | GCACCGCCGCCCAGACCTCCACC |
Primers used for generating HLA mutations.
https://doi.org/10.7554/eLife.40126.033Primer name | Primer sequence 5'−3' |
---|---|
A6801_V12M_Fwd | CTACACTTCCATGTCCCGGC |
A6801_V12M_Rev | GCCGGGACATGGAAGTGTAG |
A6801_M97R_Fwd | CACCATCCAGAGGATGTATGGC |
A6801_M97R_Rev | GCCATACATCCTCTGGATGGTG |
A6801_S105P_Fwd | CGTGGGGCCGGACGGGC |
A6801_S105P_Rev | GCCCGTCCGGCCCCACG |
A6801_R114H_Fwd | GCGGGTACCACCAGGACGCC |
A6801_R114H_Rev | GGCGTCCTGGTGGTACCCGC |
A6801_D116Y_Fwd | GTACCACCAGTACGCCTACG |
A6801_D116Y_Rev | CGTAGGCGTACTGGTGGTAC |
A6801_D116Yonly_Fwd | GTACCGGCAGTACGCCTAC |
A6801_D116Yonly_Rev | GTAGGCGTACTGCCGGTAC |
A2_Y116D_Fwd | GTACCACCAGGACGCCTACG |
A2_Y116D_Rev | CGTAGGCGTCCTGGTGGTAC |
Additional files
-
Transparent reporting form
- https://doi.org/10.7554/eLife.40126.034