Dynamic multifactor hubs interact transiently with sites of active transcription in Drosophila embryos
Figures
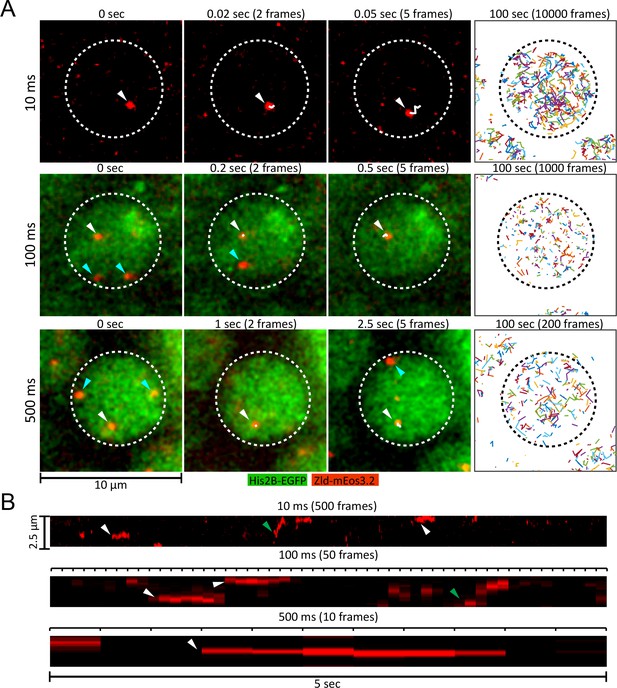
Live embryo single-molecule imaging and tracking of endogenous mEos3.2-Zld.
(A) First three columns are example images showing single molecules of mEos3.2-Zld tracked over at least five frames (white arrows and trajectories) at frame rates of 10, 100 and 500 ms. Cyan arrows indicate molecules that appear for only one frame and are thus detected but not tracked. For the 100 and 500 ms data, enough signal is present in the His2B-eGFP channel from the 405 nm activation laser to enable simultaneous imaging of chromatin. Last column shows all single-molecule trajectories acquired in each nucleus over 100 s, corresponding to 539, 263, and 186 trajectories over 10000, 1000, and 200 frames for the 10, 100 and 500 ms data, respectively. Dotted lines indicate the boundary of a nucleus. Contrast was manually adjusted for visualization. (B) Representative kymographs over 5 s of imaging, corresponding to 500, 50, and 10 frames for the 10, 100 and 500 ms frame rate data, respectively. Green arrows point to molecules that display relatively large motions, and white arrows to immobile molecules.
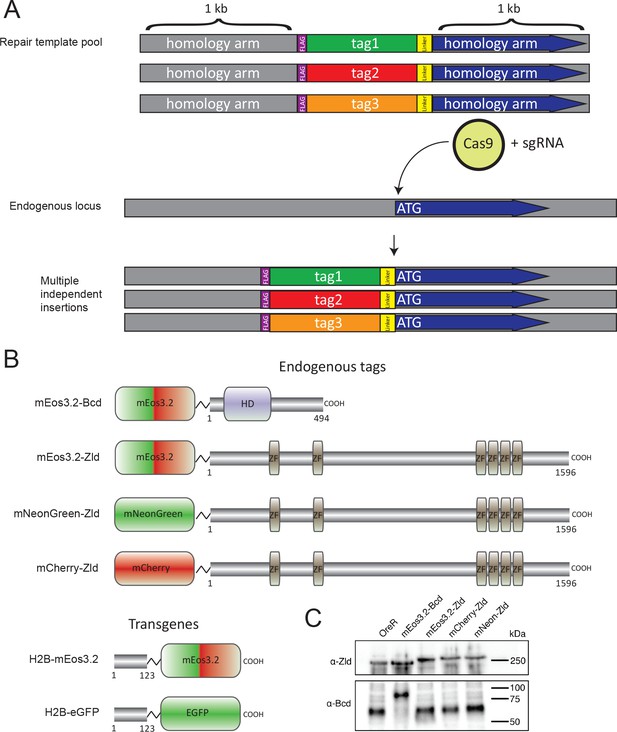
Overview of CRISPR-Cas9 genome editing strategy.
(A) Pools of homology repair template plasmids containing different protein tags were co-injected with a plasmid encoding an sgRNA targeting the N-terminus of the target coding sequence into embryos expressing transgenic Cas9. PCR genotyping and DNA sequencing were used to find chromosomes containing tag insertions and to determine the identity of the inserted protein. (B) Fusion proteins used in this study. Zelda and Bicoid fusion proteins were generated by inserting fluorescent proteins at the endogenous locus. To avoid potential problems associated with endogenous tagging of a multicopy locus, histone fusions were supplied as exogenous transgenes. (C) Western blots for Bcd and Zld in fluorescently tagged lines. OreR embryos are from a wild-type strain with untagged Bcd and Zld. The observed shifts in band size correspond to the size of fluorescently tagged Bcd or Zld protein. Expected sizes: Bcd = 54.4 kDa, mEos3.2-Bcd = 80.1 kDa, Zld = 170.1 kDa, mEos3.2-Zld = 195.8 kDa, mCherry-Zld = 198.9 kDa, mNeonGreen-Zld = 196.7 kDa.
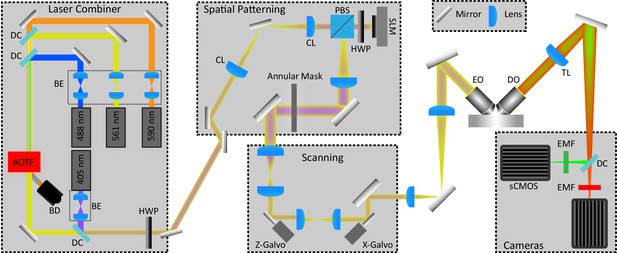
Simplified Schematic of Lattice Light Sheet Microscope.
The schematic is organized to show the major modules of the microscope. The Laser Combiner module contains six lasers (three shown here) for excitation ranging from 405 to 639 nm, each of which are independently expanded and collimated by using a pair of lenses that serve as a beam expander (BE). The paths of each laser are combined and made collinear by using 1 mirror and 1 dichroic mirror (DC) per laser. The combined beams are then input into an Acousto-optic tunable filter (AOTF) for rapid switching between lasers and control of power. A beam dump (BD) is used to safely capture the light from lasers not being used. To achieve constant and controllable photoactivation the laser combiner was modified to include a 405 nm laser that bypasses the AOTF. A half-wave plate (HWP) is used to adjust the polarization of the input light to the Spatial Patterning module. For spatial patterning, a pair of cylindrical lenses (CL) is used to stretch the Gaussian beam output from the Laser Combiner module into a thin stripe, which illuminates the Spatial Light Modulator (SLM) after passing through a polarizing beam splitter (PBS) and a second HWP. A lens projects the Fourier transform of the plane of the SLM onto an annular mask which is used to confine the spatial frequencies of the patterned light to the desired minimum and maximum numerical apertures. In the scanning module, a pair of lenses de-magnifies and projects the annular mask plane onto first the z-galvo scanning mirror for moving the light sheet through the sample, and a second pair-of lenses relays the plane of the z-galvo onto the x-galvo for dithering the sheet for uniform illumination. Another pair of lenses is then used to project a magnified image of the galvo planes to the back focal plane of the excitation objective (EO) which focuses the light to project the lattice pattern through the sample. An orthogonally placed detection objective (DO) collects the emission light, and a tube-lens (TL) then forms an image at each cameras sensor plane. A dichroic mirror first splits the light into red (>560 nm) and green (<560 nm) channels, followed by a narrower bandpass emission filter (EMF) for further filtering before each camera. With the exception of the modifications to the laser combiner module and the use of two Hamamatsu sCMOS ORCA Flash 4.0 cameras for detection the design is identical to what was originally described by Chen et al., 2014a and Chen et al., 2014b.
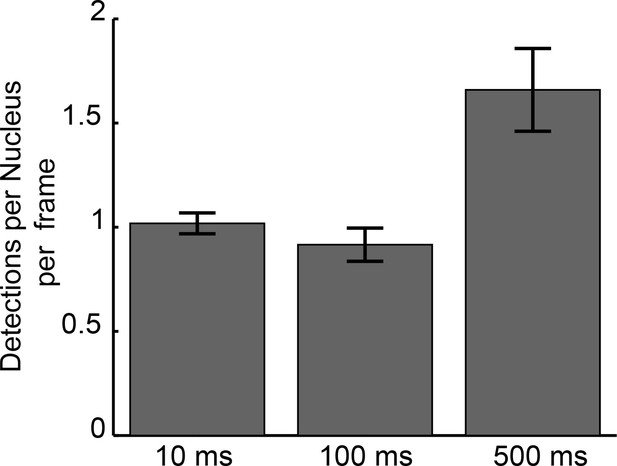
Mean detections per nucleus per frame for each frame rate.
Detections per nucleus/frame characterized over 804245, 78281, 15165 frames of imaging and 169, 434, and 359 nuclei for 10 ms, 100 ms, and 500 ms, datasets respectively. Error bars show standard errors over all nuclei.
Movie illustrating ability to controllably photactiviate mEOS3.2.
Related to Figure 1. Movie illustrating the ability to photo-activate mEos3.2 with the modified LLSM system. The power of the 405 nm laser was set to its highest value at approximately the 4 s mark after which a continuous increase in the signal can be observed reflecting the density of activated molecules.
Example movie of mEos3.2-Zld acquired at 10 ms frame rate.
Related to Figure 1. White scale bar is 5 μm, images were gaussian filtered and inverted for display.
Example movie of mEos3.2-Zld (red) and His2B-EGFP (green) acquired at 100 ms frame rate.
Related to Figure 1. White scale bar is 5 μm, a nucleus undergoing division is shown for illustrative purposes, data from mitotic nuclei were not used for single-molecule analysis in this work. Images were gaussian filtered for display.
Example movie of mEos3.2-Zld (red) and His2B-EGFP (green) acquired at 500 ms frame rate.
Related to Figure 1. White scale bar is 5 μm, data from mitotic nuclei were not used for single-molecule analysis in this work. Images were gaussian filtered for display.
Example of a mobile molecule of mEos3.2-Zld tracked at 10 ms frame rate.
Related to Figure 1. White scale bar is 2 μm. Images were gaussian filtered and inverted for display.
Example of a immobile molecule of mEos3.2-Zld tracked at 10 ms frame rate.
Related to Figure 1. White scale bar is 2 μm. Images were gaussian filtered and inverted for display.
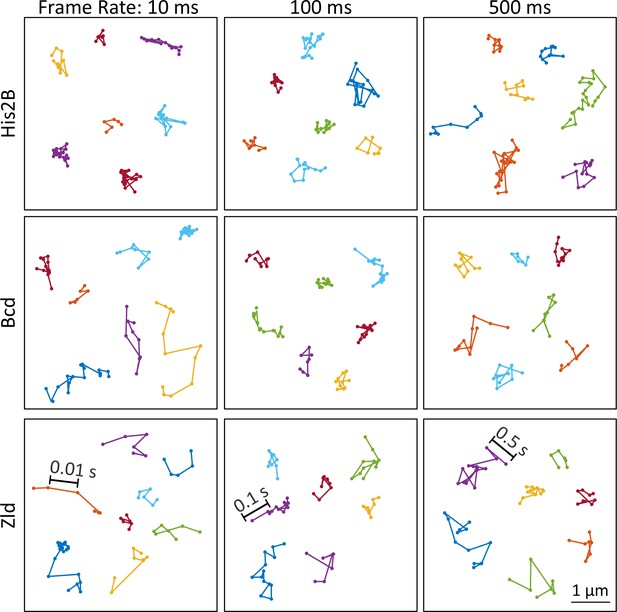
Representative single-molecule trajectories of His2B, Bcd and Zelda.
Representative single-molecule trajectories of His2B, Bcd, and Zld from data acquired at frame rates of 10, 100 and 500 ms.
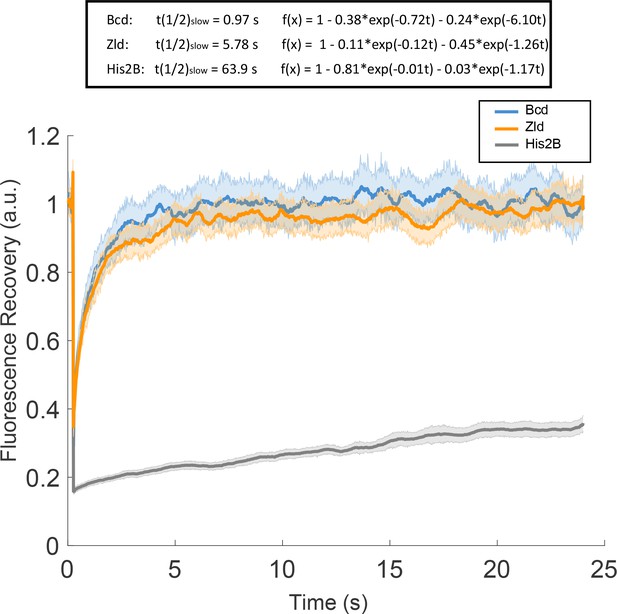
Fluorescence recovery after photobleaching (FRAP).
FRAP curves for Bcd, Zld, and His2B and results of 2 exponential fitting. Solid lines are averages over at least 27 measurements and shaded regions indicate standard error.
Comparison of single-molecule movies for His2B-mEos3.2, mEos3.2-Bcd, and mEos3.2-Zld at 10 ms frame rate.
Related to Figure 2. White scale bar is 2 μm. Panels left to right are His2B-mEos3.2, mEos3.2-Bcd, and mEos3.2-Zld. Images were gaussian filtered, inverted, and contrast was manually adjusted to allow for ease of visual comparison.
Comparison of single-molecule movies for His2B-mEos3.2, mEos3.2-Bcd, and mEos3.2-Zld at 100 ms frame rate.
Related to Figure 2. White scale bar is 2 μm. Panels left to right are His2B-mEos3.2, mEos3.2-Bcd, and mEos3.2-Zld. Images were gaussian filtered, inverted, and contrast was manually adjusted to allow for ease of visual comparison.
Comparison of single-molecule movies for His2B-mEos3.2, mEos3.2-Bcd, and mEos3.2-Zld at 500 ms frame rate.
Related to Figure 2. White scale bar is 2 μm. Panels left to right are His2B-mEos3.2, mEos3.2-Bcd, and mEos3.2-Zld. Images were gaussian filtered, inverted, and contrast was manually adjusted to allow for ease of visual comparison.
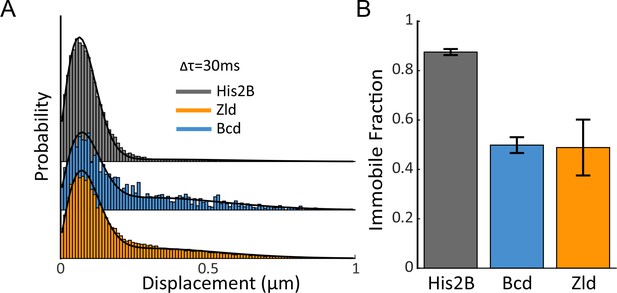
Immobile Fraction of His2B, Zld, and Bcd molecules.
(A) Histograms of displacements for His2B, Zld, and Bcd after three consecutive frames (Δτ = 30 ms) at a frame rate of 10 ms. The Zld and Bcd distributions show a right tail indicative of a large free population that is missing from His2B distribution. Black lines are fits from two-state kinetic modelling, data shown is compiled from 3 embryos totalling 77869, 81660, and 11003 trajectories and 30, 128, 41 nuclei for His2B, Zld, and Bcd, respectively. (B) Fraction of molecules bound or immobile as determined from kinetic modeling of the displacement distributions, a summary of the model parameters is shown in Figure 3—figure supplement 1. Error bars show standard errors over three embryos.
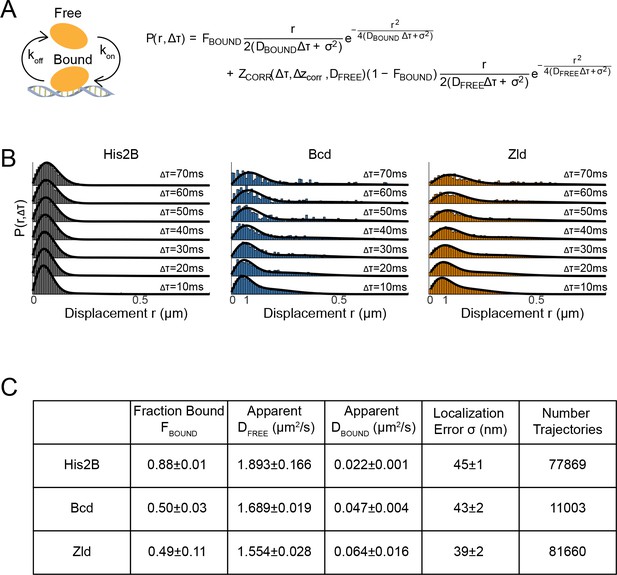
Kinetic modeling of fast SPT data.
(A) Overview of two-state model in which molecules are either in a free or bound state and the kinetic-model used to fit the displacement distributions, P(r,Δτ), where FBOUND is the fraction of molecules bound (or immobile), r is the displacement, Δτ is the time delay, σ is the localization error, and ZCORR is the function used to estimate the fraction of trajectories lost due to moving out of the axial detection range (Δzcorr) as detailed in Hansen et al., 2018. (B) Displacement distributions at multiple time delays and corresponding fits (black lines), data from one embryo is shown for each protein, three embryos were measured for each in total. (C) Summary of results from fits to the kinetic model where errors shown are over three biological replicates.
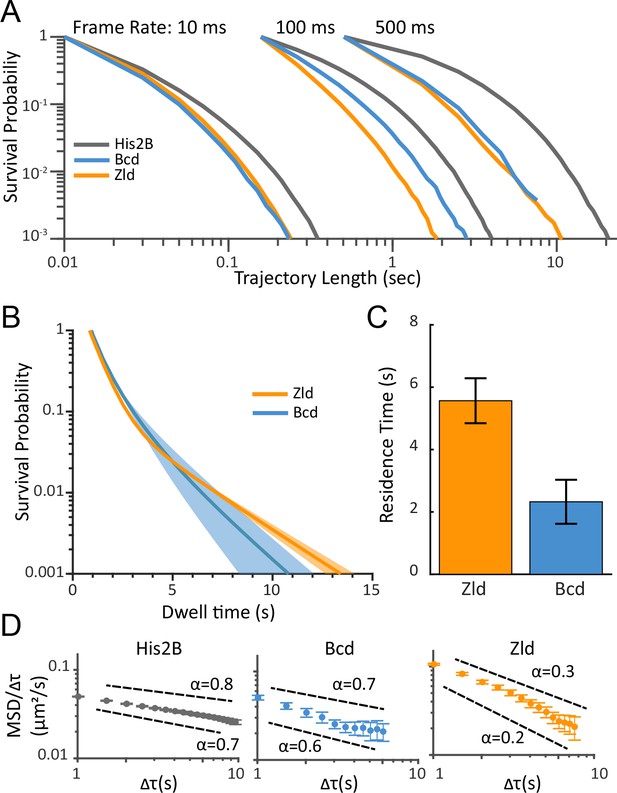
Residence times and dynamics of bound Zld and Bcd molecules.
(A) Raw survival probabilities of trajectories at all frame rates. Calculated over 77869, 81660, 11003, at 10 ms, 107998, 42698, 8990 at 100 ms, and 2420, 14487, 47681 at 500 ms, trajectories for His2B, Zld, and Bcd respectively. (B) Uncorrected two-exponent fits to the survival probability distributions obtained from the 500 ms frame rate data. Dark solid lines are the mean over fits from three embryos and the shaded regions indicate the standard error. (C) Bias corrected quantification of the slow residence times for Zld (5.56 ± 0.72 s) and Bcd (2.33 ± 0.71 s). Error bars indicate standard error over three embryos for a total of 188 and 171 nuclei for Bcd and Zld, respectively. (D) MSD/τ curves for His2B, Bcd, and Zld at 500 ms frame rates plotted on log-log-scale. For anomalous diffusion MSD(τ)=Γτα, where α is the anomalous diffusion coefficient. For MSD/τ, in log-log space, the slope is thus 0 for completely free diffusion that is when α = 1, and sub-diffusive(0<α<1), motions display higher negative slopes, with lower α corresponding to more anomalous motion.
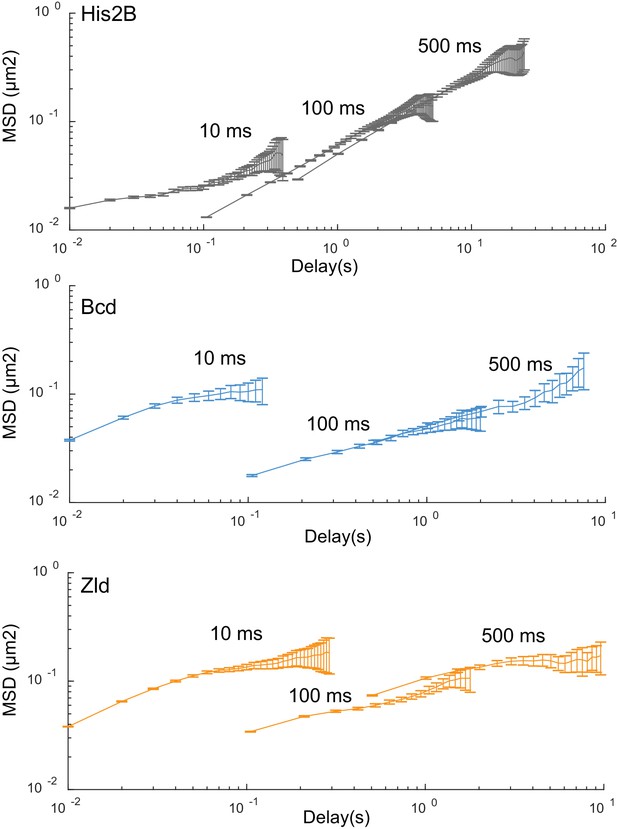
Mean square displacement curves.
MSD curves for His2B, Zld, and BCD, at all frame rates plotted on log-log-scale. Calculated over 77869, 81660, 11003, at 10 ms, 107998, 42698, 8990 at 100 ms, and 2420, 14487, 47681 at 500 ms, trajectories for His2B, Zld, and Bcd respectively. Error bars show standard error over all trajectories.
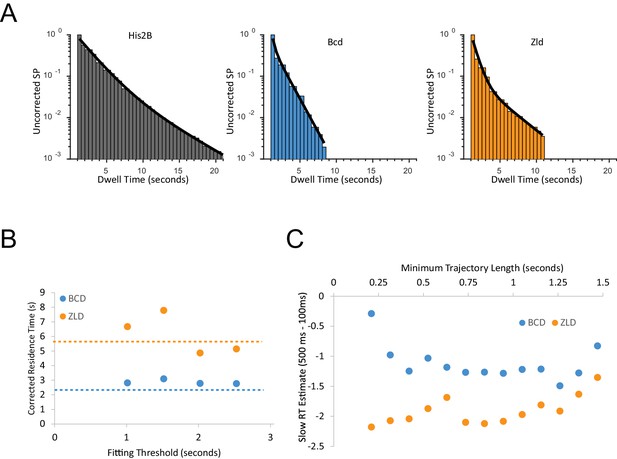
Inference of residence times from single-molecule trajectories.
(A) Example fits of two-exponent model (black line) to the survival probability (SP) distributions data from a single embryo for each protein studied (B) Bias corrected residence time (RT) as a function of the minimum trajectory length threshold used for fitting, the reported inferred residence time is from when the fit parameter plateaus at a threshold of 2.02 s for both proteins (C) Comparison of inferred residence times from 100 ms vs 500 ms frame rate data sets as a function of the minimum trajectory length fitting threshold.
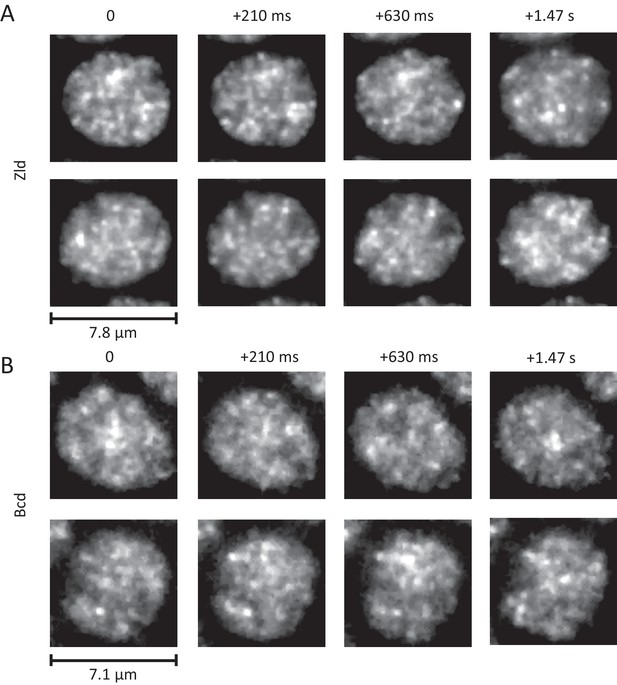
Dynamic interphase hubs of Zld and Bcd.
Example images of the spatial distributions of Zld (A) and Bcd (B) at various time intervals illustrating the dynamic nature and wide range of size distributions and temporal persistences of enriched hubs (see also Figure 5—videos 2–4). mNeonGreen-Zld and EGFP-Bcd were imaged at 15 ms and 210 ms frame rates, respectively. To allow comparison, the sum projection of 14 frames (210 ms total integration) is shown for Zld. Images were processed with a 1-pixel radius median filter to remove salt-and-pepper noise and contrast-adjusted manually for visual presentation.
Cell cycle dynamics of Zelda spatial distribution.
Related to Figure 5. Maximum intensity projection over 81 slices spaced at 250 nm apart. Right is His2B-mCherry and left is sfGFP-Zld imaged through nuclear cycles 13 and 14. His2B is present at high concentrations in both the cytoplasm and nucleoplasm of Drosophila embryos, resulting in the extranuclear signal visible in the right panel. Time interval between each volume is 8.91 s. The field of view is 23.2 × 23.2 μm.
Four-dimensional Interphase dynamics of Zld spatial distributions.
Related to Figure 5. Three-dimensional rendering of Zld spatial distribution in nuclear cycle 13. Progress bar indicates time in minutes. Slice spacing in z is 200 nm, time between volumes is 9.5 s.
Interphase dynamics of Zld spatial distributions at high temporal resolution.
Related to Figure 5. Interphase dynamics of Zld spatial distribution at 10 ms exposure times and 15 ms frame rate.
Four dimensional dynamics of Bcd spatial distribution.
Related to Figure 5. Three-dimensional rendering of Bcd spatial distribution from nuclear cycles 13–14. Progress bar indicates time in minutes. Slice spacing in z is 200 nm, time between volumes is 8 s.
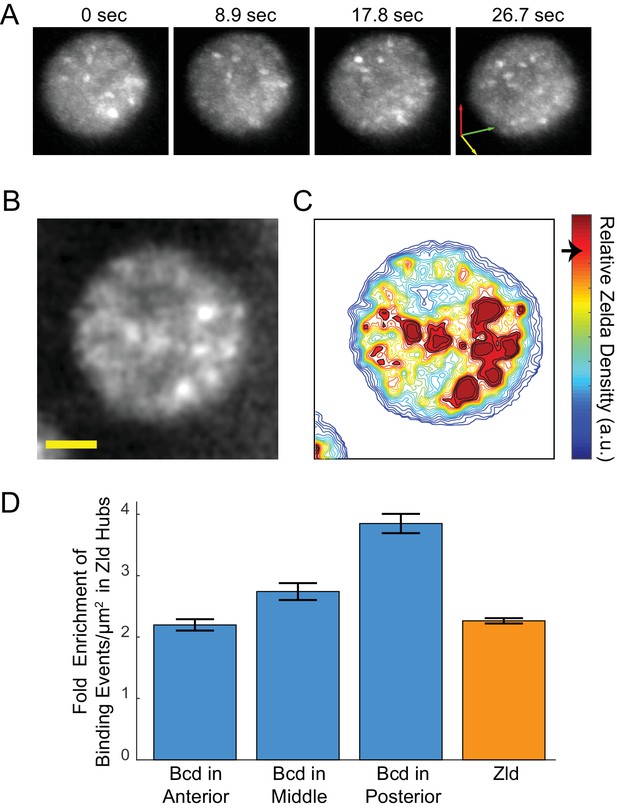
Enrichment of Bcd binding in Zld hubs.
(A) Three-dimensional volume renderings of an interphase nucleus showing the dynamic nature of Zld hubs (see Figure 5—video 2, Figure 5—video 3).The 3D axes indicate the xyz axes and the arrow lengths are 2 μm along each direction. (B) Representative snapshot of the interphase distribution of Zelda, yellow scale bar is 2 μm. (C) Relative Zelda Density map for the nucleus shown in (B), the arrow on the colorbar indicates the threshold for defining a region as high density (corresponding to hubs). (D) Fold enrichment in the density of bound molecules of Bcd in the anterior, middle, and posterior embryo, and of Zld, in Zld hubs vs. the rest of the nucleoplasm. Error bars show standard error over three embryos with a total of 1344, 3921, 481 nuclear images for Bcd Ant, Mid, and Post, respectively, and 4399 for Zld.
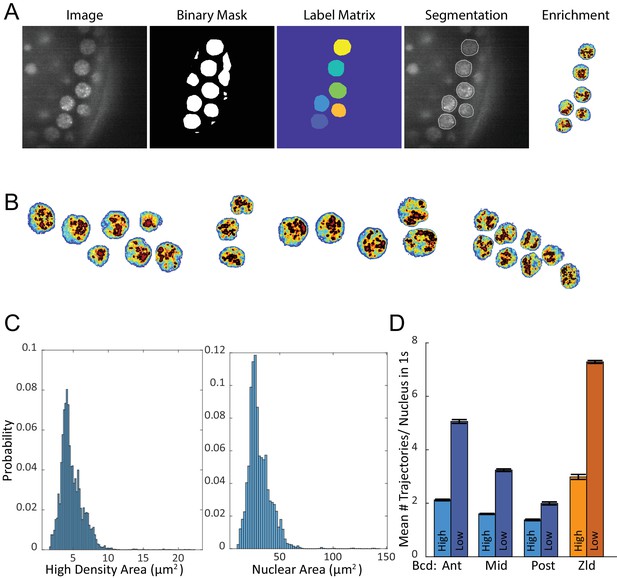
Analysis of Zelda density.
(A) Major steps in nuclear segmentation algorithm, the Zelda image is first low-pass filtered and then converted to a binary mask through adaptive thresholding, a hand drawn mask is applied to remove regions of low contrast and the embryo edge. The binary mask is dilated, a label matrix is calculated and regions not meeting eccentricity and size cutoffs are removed. The relative density map for each nucleus is then calculated using the distribution of intensity values within each nucleoplasm. (B) More examples of calculated density maps. (C) Histograms of high-density areas and nuclear areas. Distributions are from 33,041 images of nuclei. (D) Mean number of single trajectories in high-density vs. low-density regions per nuclear image, for a total of 1344, 3921, 489, nuclear images in the Anterior (Ant), Middle (Mid), and Posterior(Mid) positions for Bcd, respectively, and 4399 for Zld with trajectories (# in High Density/# in low Density) of 4326/12437, 3921/11211, 836/1793 for Bcd Ant, Mid, and Post, respectively, and 25470/66923 for Zld. Error bars are standard errors over all nuclear images.
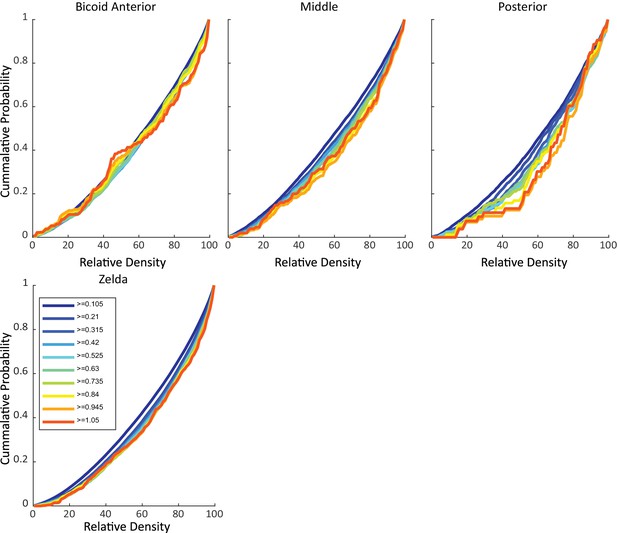
Cumulative probability of trajectories vs. relative Zelda density.
Plots show the cumulative probability of a trajectory greater than or equal to a certain length in time (as indicated by color) vs. the relative density of Zld. The top row shows the probability for single-molecule trajectories of Bicoid across the Anteroposterior axis and the bottom panel is for Zld single-molecule trajectories. For Bcd-binding events, in the anterior there is little cost in terms of Zld density for increased trajectory lengths, but in middle and posterior positions greater Zld enrichment is required for molecules to be immobilized for longer.
Bcd single-molecule localizations in context of the bulk spatial distribution of Zld.
Related to Figure 6. Bicoid detections (red dots) overlaid on Zld spatial distribution. Each frame in the movie corresponds to 1 s of detections at 100 ms exposure times. Field of view is 9 × 14 μm.
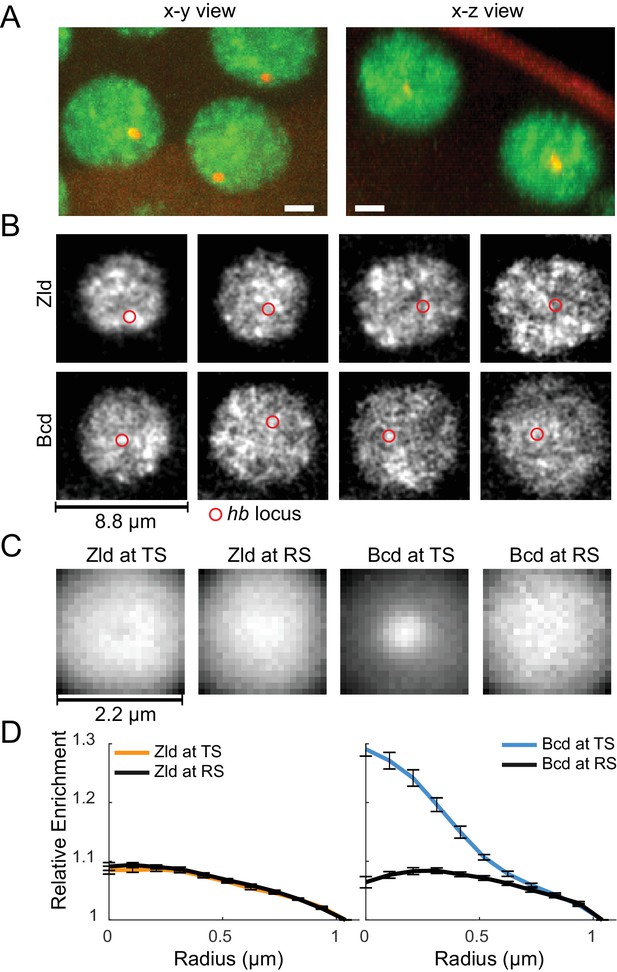
Spatio-temporal distribution of Zld and Bcd hubs in context of active hb loci.
(A) Representative x-y and x-z max projections over a nuclear diameter of mNeonGreen-Zld (green) and an active hb locus tagged with MS2-MCP-mCherry (red) white scale bars are 2 μm. (B) Representative snapshots of the distribution of Zld and Bcd with the hb locus indicated by the red circle. Images suggest that high concentration Bcd hubs frequent the active locus whereas Zld exhibits more transient and peripheral interactions. Contrast of each image was manually adjusted for visualization and comparison. (C) Average Zld and Bcd signals in a 2.2 μm window centered at active hb locus (TS) and at random sites in the nucleus (RS). Averages were calculated over 3943 and 6307 images of active loci from six embryos for Bcd and Zld, respectively (see Figure 7—videos 4 and 5). (D) Radial profiles of the images in C, normalized to one at the largest radius. Error bars show standard error over all images analyzed.
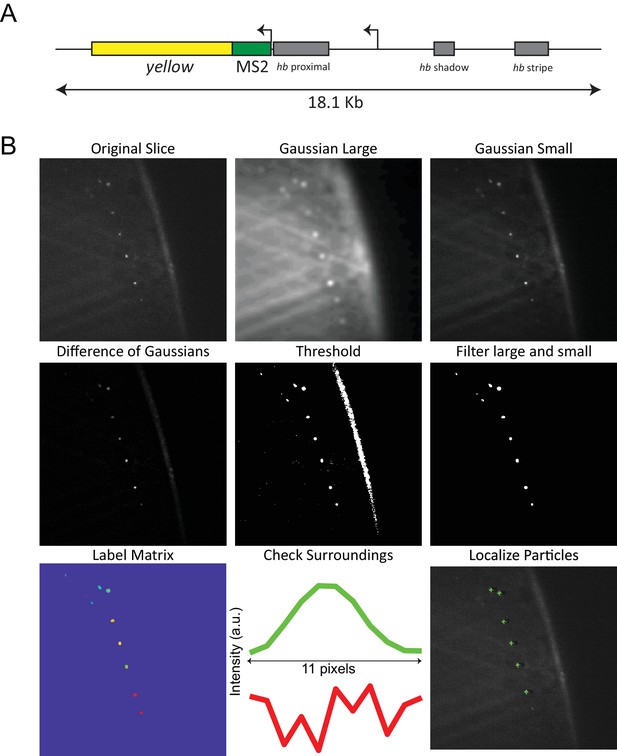
MS2 system and data analysis.
(A) BAC construct used in the experiments to visualize transcription of hb, from Bothma et al. (2015). The coding region is replaced by the yellow gene and 24 MS2 stem loops are inserted downstream of the P2 promoter, allowing the visualization of transcripts from both proximal and distal promoters. The BAC spans an 18.1 kb genomic region containing all known hb regulatory sequences, and its expression closely mirrors that of native hb. (B) Segmentation and localization of active loci. A difference of gaussians is used to enhance the contrast of transcription sites, a binary mask is calculated by applying a threshold and filtered to remove large and small structures. A label matrix for remaining segmented regions is then generated and line profiles through the center of the regions is used to determine if they lie inside of a nucleus. Finally, the center of the active locus is determined using the line profile.
Four dimensional imaging of protein distribution in the context of transcription.
Related to Figure 7. Example of four dimensional imaging of Zld spatial distribution in the context of an active transcription site imaged using the MS2-MCP system (red).
Example of Bcd spatial distribution around an active hb locus.
Related to Figure 7. eGFP-Bcd (green) and MCP-mcherry (red) dynamics from nuclear cycles 13–14. Maximum intensity projection over 61 slices spaced 250 nm apart with ~6 s between volumes.
Example of Zld spatial distribution around an active hb locus.
Related to Figure 7. mNeonGreen-Zld (green) and MCP-mcherry (red) dynamics from nuclear cycles 13–14. Maximum intensity projection over 61 slices spaced 250 nm apart with 5.18 s between frames.
Calculation of average Zld signal around active hb loci.
Related to Figure 7. Top panel shows images of the MS2 signal, corresponding Zld signal in the same window (TS) and at a random control site in the same nucleus (RS). Middle panel shows a running average of the images in the top panel and bottom panel shows the corresponding running average radial profile.
Calculation of average Bcd signal around active hb loci.
Related to Figure 7. Top panel shows images of the MS2 signal, corresponding Bcd signal in the same window (TS) and at a random control site in the same nucleus (RS). Middle panel shows a running average of the images in the top panel and bottom panel shows the corresponding running average radial profile.
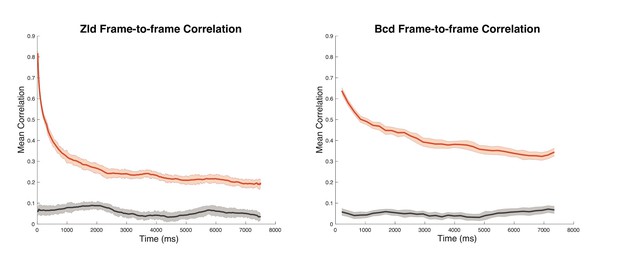
Frame-to-frame correlation of nuclear protein fluorescence generated from movies with a 15 ms frame rate for Zelda and 210 ms frame rate for Bcd.
Nuclei were manually segmented, fluorescent signal was normalized independently for each nucleus and each frame, and the pixel-wise correlation of nuclear signal between frames was calculated. Vectors of correlation coefficients were generated for series of frames beginning every by 420 ms, and coefficients at each time offset (+1 frame, +2, +3…) were averaged to produce the plots. The decay in correlation for the nuclei for each protein is shown in red; gray lines represent controls in which nuclei were permuted and correlations were measured between fluorescent signal in different nuclei. Shading represents standard error of the mean.
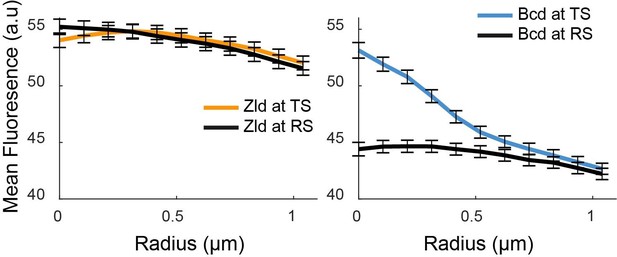
Videos
Tables
Reagent type (species) or resource | Designation | Source or reference | Identifiers | Additional information |
---|---|---|---|---|
Strain, strain background (D. melanogaster) | mNeonGreen-Zld | this paper | Fly line with an N-terminal mNeonGreen fusion tag inserted at the endogenous Zld locus. | |
Strain, strain background (D. melanogaster) | mEos3.2-Zld | this paper | Fly line with an N-terminal mEos3.2 fusion tag inserted at the endogenous Zld locus. | |
Strain, strain background (D. melanogaster) | H2B-EGFP | this paper | Fly line with an H2B-EGFP transgene inserted on chromosome 3. Transgene is expressed ubiquitously under the control of a synthetic tubulin promoter. | |
Strain, strain background (D. melanogaster) | H2B-mEos3.2 | this paper | Fly line with an H2B-mEos3.2 transgene inserted on chromosome 3. Transgene is expressed ubiquitously under the control of a synthetic tubulin promoter. | |
Strain, strain background (D. melanogaster) | MCP-mcherry | H Garcia lab | Fly line with MCP-mCherry inserted as a transgene on chromosome 2. | |
Genetic reagent (D. melanogaster) | sgRNA #1 bicoid | this paper | sgRNA targeting N-terminus of Bcd gene, sequence GCGGAGTGTTTGGGGAAAA | |
Genetic reagent (D. melanogaster) | sgRNA #1 bicoid | this paper | sgRNA targeting N-terminus of Bcd gene, sequence TAAAAGTTTTGATCTGGCGG | |
Genetic reagent (D. melanogaster) | sgRNA #1 bicoid | this paper | sgRNA targeting N-terminus of Bcd gene, sequence TGATGGTAAAAGTTTTGATC | |
Genetic reagent (D. melanogaster) | sgRNA Zelda | M Harrison lab | sgRNA targeting N-terminus of Zld gene, sequence CCTCTGCCGCGTGCAGGGG | |
Software, algorithm | Spot-On | Hansen et al., 2018, eLife |
Additional files
-
Transparent reporting form
- https://doi.org/10.7554/eLife.40497.038