High-throughput synapse-resolving two-photon fluorescence microendoscopy for deep-brain volumetric imaging in vivo
Figures
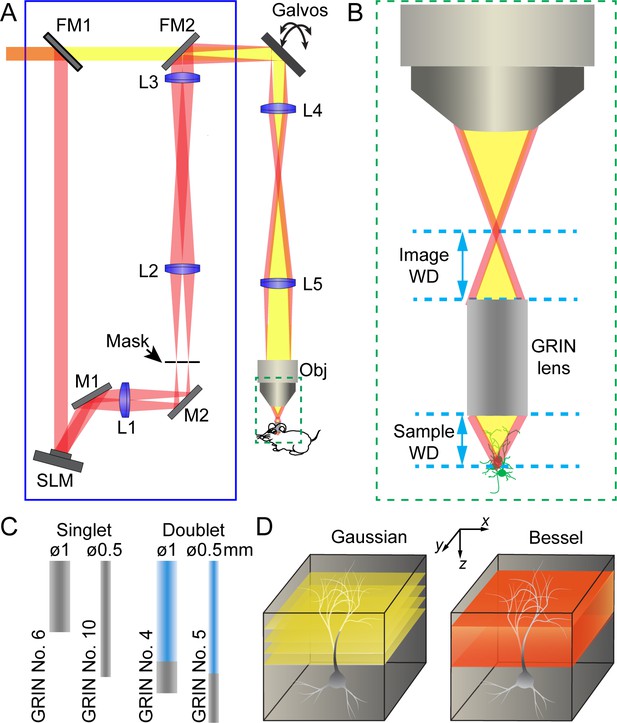
Schematics of two-photon excitation fluorescence microendoscopy with a Bessel focus scanning module.
(A) A Bessel focus scanning module (blue rectangle) made of a spatial light modulator (SLM), a lens (L1), and an annular mask is incorporated into a two-photon fluorescence microendoscopy system. L2-L5: lenses for optical conjugation; M1, M2: turning mirrors; Galvos: x and y galvanometers; Obj: microscope objective; FM1, FM2: flip mirrors to switch between Gaussian (yellow) and Bessel (red) beam paths. (B) Enlarged view of a GRIN lens relaying the focus of the objective into a focus inside the brain. Image WD: image working distance, distance between the objective focus and the top surface of GRIN lens; Sample WD: sample working distance, distance between the bottom of the GRIN lens and the sample structure in focus. (C) Two singlet and two doublet GRIN lenses selected for further characterization. (D) To image a volume requires multiple 2D scans of a Gaussian focus but a single 2D scan with a Bessel focus.
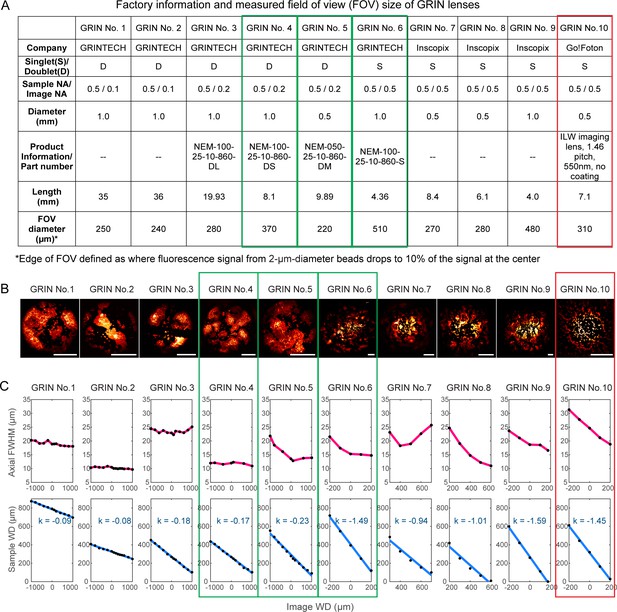
Characterization of ten GRIN lenses.
(A) Factory information and field of view (FOV) sizes of ten GRIN lenses. (B) Maximal intensity projections of 3D image stacks of 2-µm-diameter fluorescent beads obtained under conventional Gaussian focus scanning mode (3D stack was required due to field curvature). Scale bar: 100 µm. (C) Top panel: the axial full width at half maximum (FWHM) of 2-µm-diameter beads at different image working distance (WD). Bottom panel: measured (black dot) relationship between image WD and sample WD, with k being the slope of a linear fit (blue line).
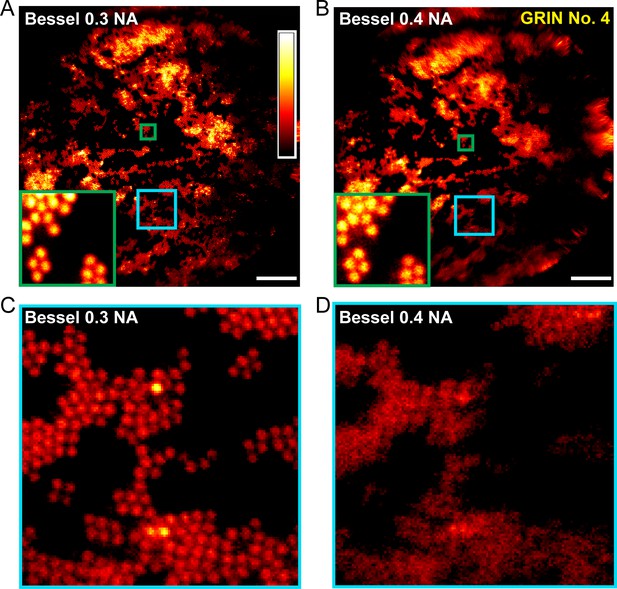
0.3-NA Bessel foci achieves better imaging performance than 0.4-NA Bessel foci.
(A,B) Images of 2-μm-diameter fluorescent beads obtained with Bessel foci of 0.3 NA, 51 µm axial FWHM and 0.4 NA, 45 µm axial FWHM, respectively. Insets show enlarged views of beads at FOV center (green squares). (C,D) Enlarged views of beads away from the FOV center (cyan squares in A and B). Scale bar: 20 µm.
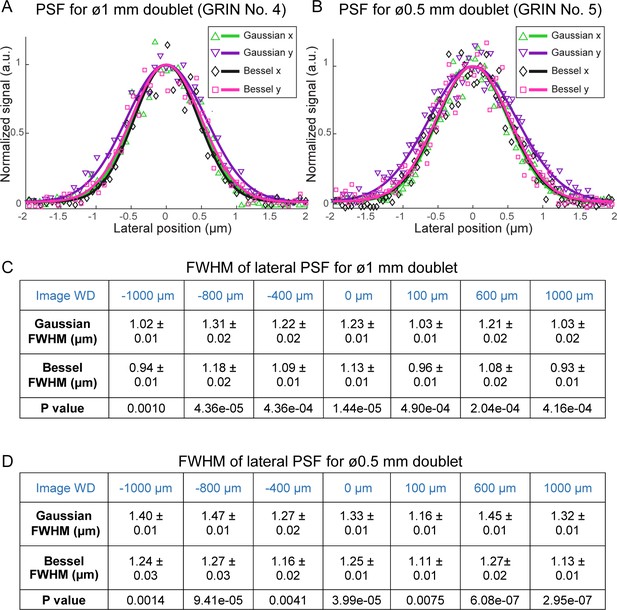
0.3-NA Bessel foci have higher lateral resolution than 0.5-NA Gaussian foci through GRIN lenses.
(A,B) Lateral point spread functions (PSFs) of 0.3-NA Bessel foci (axial FWHM: 57 µm) and 0.5-NA Gaussian foci measured with 0.2-µm-diameter fluorescent beads through 1 mm and 0.5 mm doublets, respectively, at 0 µm image WD. PSFs along x and y directions were fitted with a normalized Gaussian function. (C,D) Bessel foci have narrower lateral PSFs than Gaussian foci across the full range of image WDs tested (−1000 µm to 1000 µm) for 1 mm and 0.5 mm doublets. FWHMs: mean ±standard errors of 6–11 measurements. Sample size depends on the number of isolated beads in the acquired images. P values: non-paired t-tests.
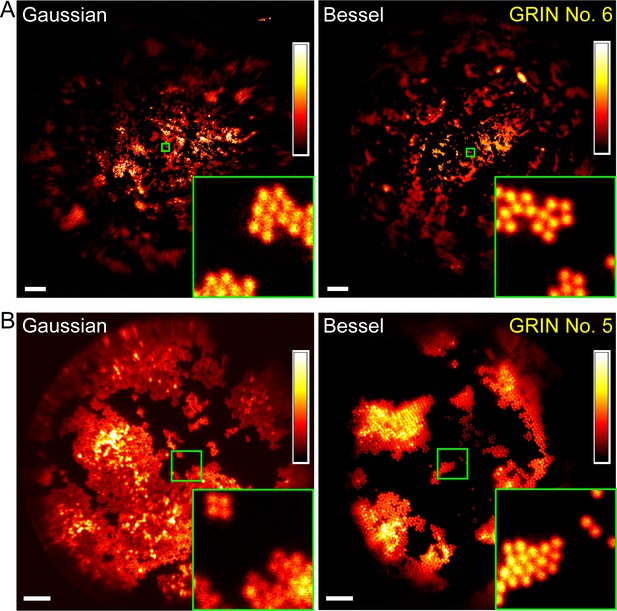
Bessel focus scanning produces superior imaging performance to Gaussian focus scanning.
(A) Through a 1-mm-diameter singlet GRIN lens, Bessel focus scanning has a larger FOV and resolves 2-µm-diameter beads at the central FOV. (B) Through a 0.5-mm-diameter doublet GRIN lens, Bessel focus scanning has similar FOV to Gaussian focus scanning and resolves 2-µm-diameter beads at the central FOV. Insets: zoomed-in views of beads at FOV centers (green squares) indicate that Bessel foci have higher lateral resolution than Gaussian foci. Scale bar: 60 µm in (A); 20 µm in (B).
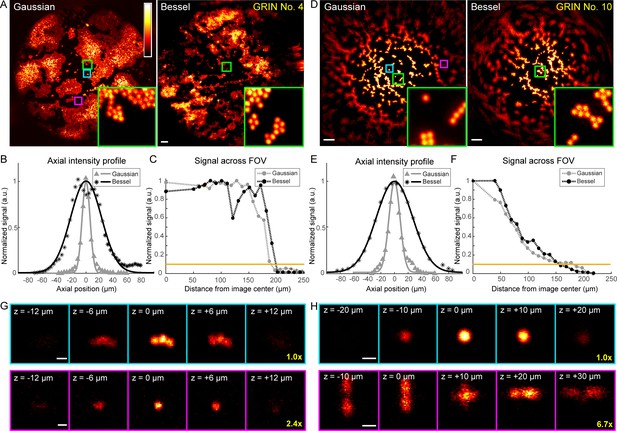
Higher quality GRIN lenses produce superior images with both Gaussian and Bessel foci.
(A) Images of 2-µm-diameter fluorescent beads obtained with a 0.5-NA Gaussian and a 0.3-NA Bessel focus, respectively, through a 1-mm-diameter doublet GRIN lens. Insets: zoomed-in views of beads at the FOV center (green square). (B) Axial intensity profiles of the Gaussian and Bessel foci used in (A). (C) Signal variation across FOVs in (A); horizontal orange line indicates 10% of signal at the center, thus the border of the FOV. (D–F) Same measurements as (A–C), but for a 0.5-mm-diameter singlet GRIN lens of inferior imaging quality. (G, H) Gaussian image stacks of beads (upper panel) at FOV center (cyan square in A and D) and (lower panel) away from FOV center (purple square in A and D, 82 µm and 89 µm from center), respectively. Scale bars: 20 µm in (A, D), 2 µm in (G, H).
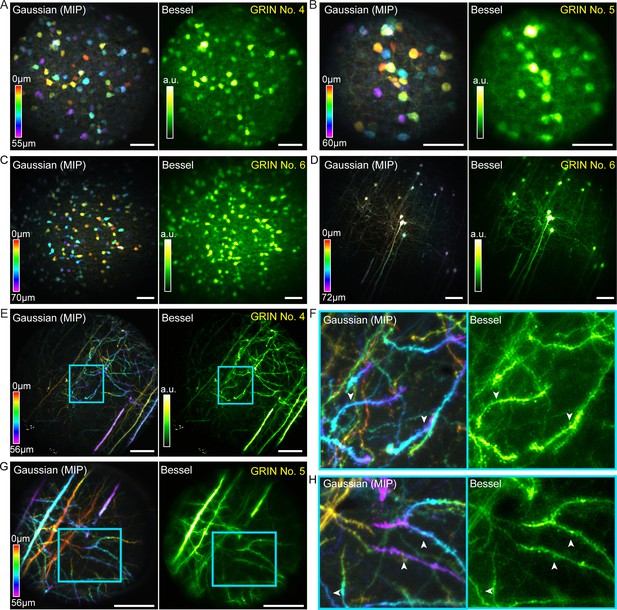
Bessel focus scanning improves imaging throughput and lateral resolution of two-photon fluorescence microendoscopy in fixed brain tissue.
(A) Left: GABAergic neurons in a 370 × 370 × 55 µm3 volume color-coded by depth, imaged via 12 2D scans of a Gaussian focus through GRIN No. 4. Right: A single 2D scan of a Bessel focus imaged all the neurons. Post-GRIN lens power: 20 mW for Gaussian, 41 mW for Bessel. (B) GABAergic neurons in a 220 × 220 × 60 µm3 volume imaged through GRIN No. 5: 13 2D scans of a Gaussian focus (21 mW) and a single 2D scan of Bessel focus (55 mW). (C) GABAergic neurons in a 510 × 510 × 70 µm3 volume imaged through GRIN No. 6: 15 2D scans of a Gaussian focus (13 mW) and a single 2D scan of Bessel focus (50 mW). (D) Pyramidal neurons and their neurites across a 510 × 510 × 72 µm3 volume imaged through GRIN No. 6: 19 2D scans of a Gaussian focus (39 mW) and a single 2D scan of a Bessel focus (100 mW). (E) Pyramidal neuron neurites in a 370 × 370 × 56 µm3 volume imaged through GRIN No. 4: 15 2D scans of a Gaussian focus (45 mW) and a single 2D scan of Bessel focus (94 mW). (G): Pyramidal neuron dendrites in a 220 × 220 × 56 µm3 volume imaged through GRIN lens No. 5: 15 2D scans of a Gaussian focus (34 mW) and a single 2D scan of a Bessel focus (95 mW). (F, H) Zoomed-in views of dendrites from the cyan boxes in (E, G). White arrowheads indicate dendritic spines that were more easily visualized by Bessel focus. Scale bar: 60 µm.
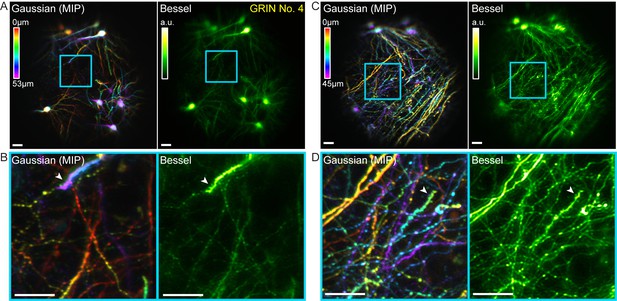
Bessel focus scanning allows volumetric microendoscopic imaging of dendritic spines and axonal boutons in mouse hippocampus in vivo.
(A) Left panel: Hippocampal neurons and neurites within a 370 μm × 370 μm × 53 μm volume color-coded by depth, imaged via 14 2D scans of a Gaussian focus through a 1-mm-diameter doublet GRIN lens. Right panel: Image obtained by a single 2D scan of a Bessel focus (NA: 0.3, axial FHWM: 53 μm). Post-GRIN lens power: 46 mW for Gaussian, 84 mW for Bessel. Image WD: 0 μm. (B) Zoomed-in views of dendritic spines and axonal boutons within the cyan box in (A). (C) Left panel: Hippocampal axons and dendrites within a 370 μm × 370 μm × 45 μm volume color-coded by depth, imaged via 11 2D scans of a Gaussian focus. Right panel: Image obtained by a single 2D scan of the Bessel focus. Post-GRIN lens power: 60 mW for Gaussian, 110 mW for Bessel. Image WD: 400 μm. (D) Zoomed-in views of dendritic spines and axonal boutons within the cyan box in (C). White arrowheads point to dendritic spines. Scale bar: 20 μm.
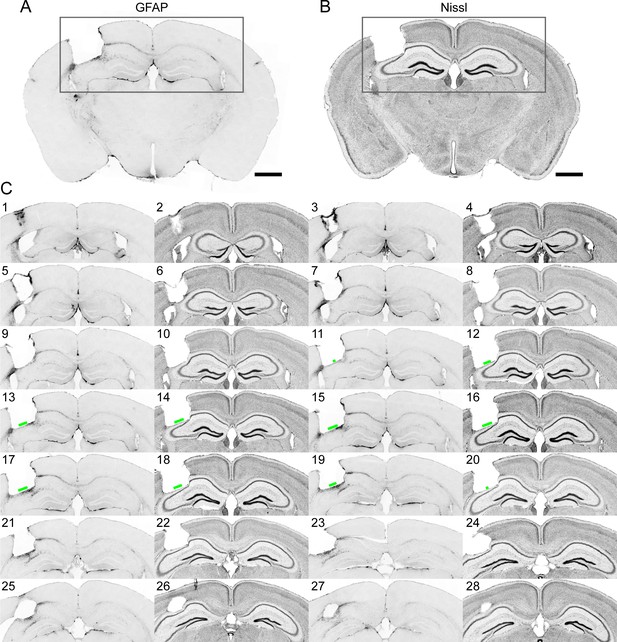
Immunohistochemistry on brain sections surrounding the GRIN lens implantation site.
(A) and (B) Representative brain slices labeled with GFAP antibody and Nissl staining, respectively. (C) Consecutive sections of a brain alternately stained against GFAP (odd number labels) and Nissl staining (even number labels). The green bars indicate the size of the field of view for this GRIN lens (GRIN lens No. 4). Scale bar: 1 mm.
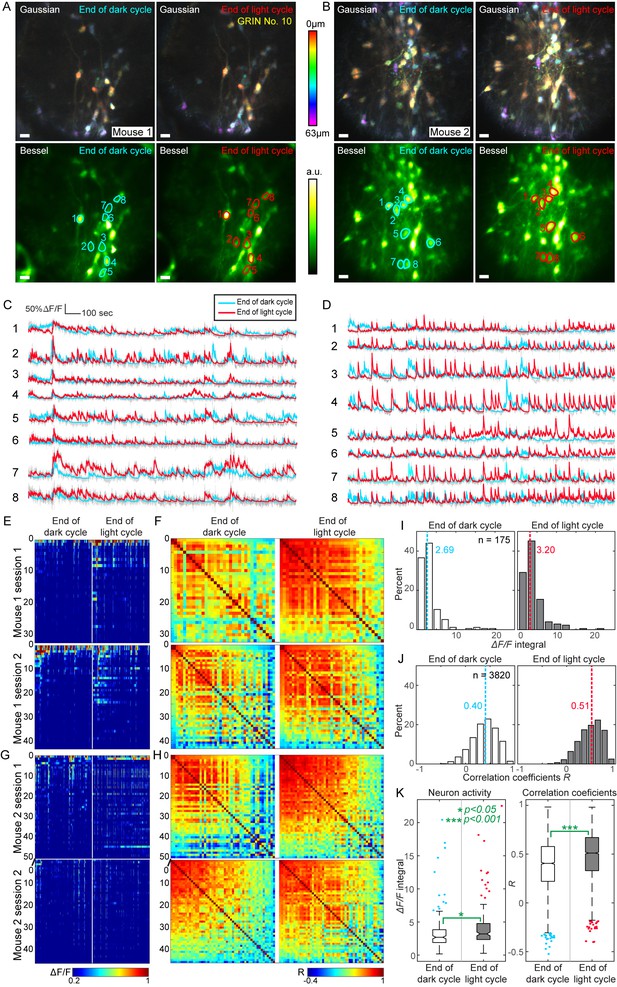
High throughput functional imaging via Bessel focus scanning enables monitoring of 3D population dynamics of GABAergic neurons in lateral hypothalamus during different metabolic states.
(A, B) In two mice, Gaussian stacks were taken through a 0.5-mm-diameter singlet GRIN lens of neurons in a 310 μm × 310 μm × 63 μm volume (top panels, color-coded by depth), followed by functional volumetric imaging using Bessel focus scanning for 30 min to record spontaneous activity from these neurons at the ends of dark and light cycles (bottom panels show the time-averaged images). Post-GRIN lens power: 70 mW for Gaussian; 115 mW for Bessel; Axial FWHM: 19 μm for Gaussian; 63 μm for Bessel. Scale bar: 20 μm. (C,D) ∆F/F calcium transient traces of neurons outlined in A and B, respectively. (E) Raster plots of ∆F/F from all identified neurons in Mouse 1, sorted by their activity at the end of dark cycle. (F) Correlation coefficient (R) matrices between ∆F/F traces from all neuron pairs in E. (G–H) Raster plots and R matrices for Mouse 2. (I,J) Histograms of activity (integral of ∆F/F traces) and R. Dashed lines: medians. (K) Notched box whisker plots of neuronal activity and R, showing statistically significant differences in neuronal activity and their correlation at the ends of dark and light cycles (non-paired t-test). Outliers highlighted in the box-whisker plots were included in the statistical analysis.
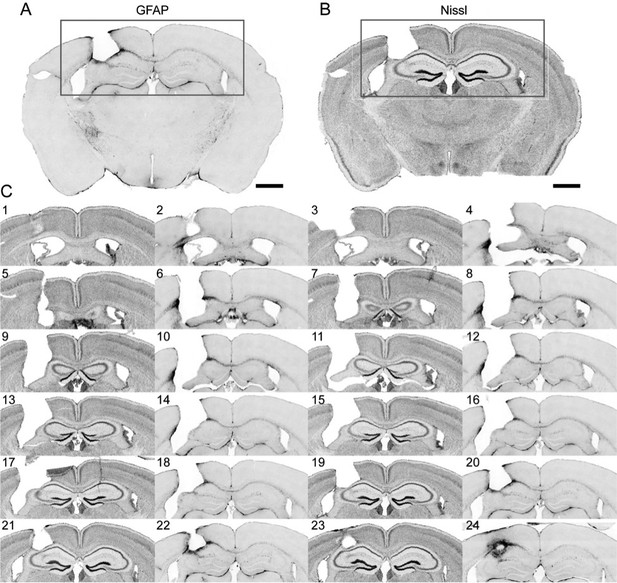
Immunohistochemistry on brain sections surround the GRIN lens implantation site.
(A) and (B) Representative brain slices labeled with GFAP antibody and Nissl staining, respectively. (C) Consecutive sections of a brain alternately stained against GFAP (even number labels) and Nissl staining (odd number labels). Scale bar: 1 mm.
Videos
Example volumetric microendoscopy recordings of GCaMP6s+ lateral hypothalamic GABAergic neurons in Figure 10A (Mouse 1).
All the neurons within the 310 µm × 310 µm × 63 µm volume were in focus throughout the recording, indicating the absence of axial motion artifact via Bessel focus scanning. Left panel: unregistered stack; Right panel: image stack after correcting lateral motion with TurboReg plugin (Thévenaz et al., 1998) followed by a non-rigid lateral motion correction method (Pnevmatikakis and Giovannucci, 2017).
Example volumetric microendoscopy recordings of GCaMP6s+ lateral hypothalamic GABAergic neurons in Figure 10B (Mouse 2).
All the neurons within the 310 µm × 310 µm × 63 µm volume were in focus throughout the recording, indicating the absence of axial motion artifact via Bessel focus scanning. Left panel: unregistered stack; Right panel: image stack after correcting lateral motion with TurboReg plugin (Thévenaz et al., 1998) followed by a non-rigid lateral motion correction method (Pnevmatikakis and Giovannucci, 2017).
Additional files
-
Transparent reporting form
- https://doi.org/10.7554/eLife.40805.014