Synergy between RecBCD subunits is essential for efficient DNA unwinding
Figures
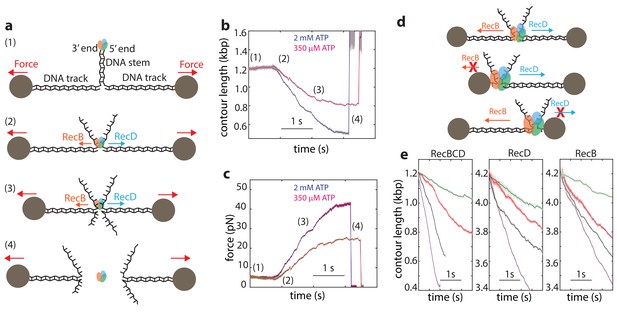
Monitoring RecBCD and its individual subunits ‘in complex’.
(a) Schematic representation of the experimental optical tweezers setup. From top to bottom: RecBCD binds to, and translocates on a DNA stem connected to optically trapped beads through DNA ‘tracks’. Upon reaching the fork, the helicase subunits translocate in different directions due to their opposing polarities, shortening the tether length and increasing the tension on it. The force increases up to a point where RecBCD dissociates from the construct. (b) Two representative contour-time traces, for [ATP] = 2 mM (blue) and [ATP] = 350 uM (purple). Raw data is shown in grey, filtered data (fc = 250 Hz) is shown in blue and purple. (c) The corresponding force-time trace. Raw data is shown in grey; filtered data is shown in blue. (d) Symmetric molecular construct (top, 600 bp and 600 bp) to probe RecBCD and asymmetric constructs (middle, 35 nt and 4200 bp, and bottom, 4200 bp and 35 nt) to probe RecB and RecD, respectively. (e) Representative traces probing both translocases (left), RecD (middle) and RecD (right), at different ATP concentrations (purple, 2 mM; gray, 1 mM; red, 100 μM; green, 20 μM). Raw data is shown in light colors, filtered data (fc = 250 Hz) is shown in dark colors.
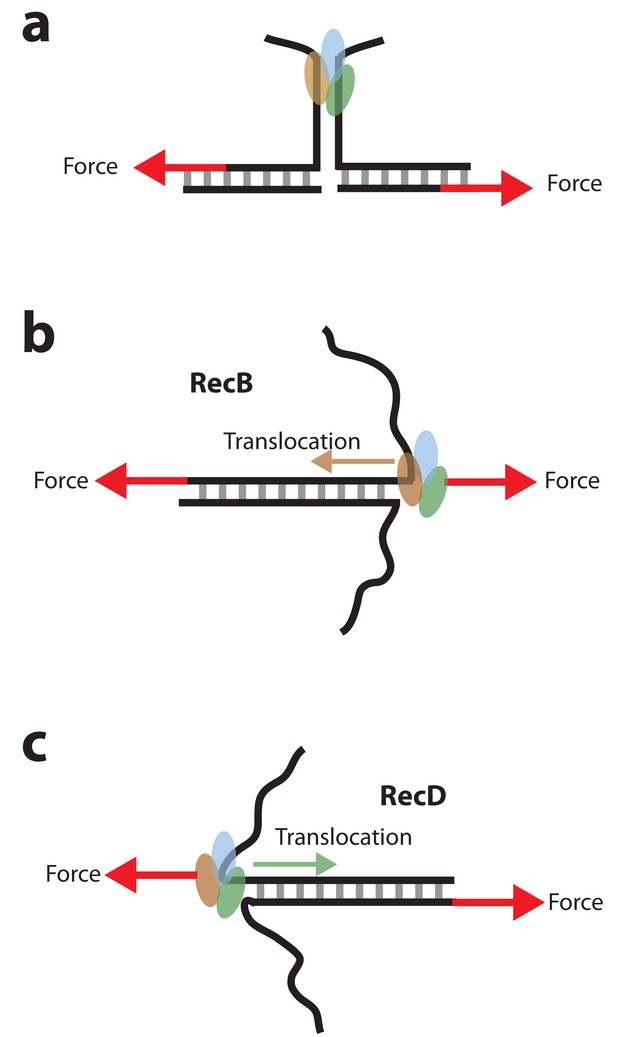
Schematic description of the force effect.
(a) During unwinding of the ‘stem’, the force acts on the DNA fork, not on RecBCD, and is below the force required to ‘unzip’ the DNA. (b–c) As RecBCD finishes unwinding the stem and each subunit engages its track, an effective force acts pulling the DNA on one side and each subunit on the other, in the direction opposite to its translocation. This opposing force functions as an inhibitor of the translocation step.
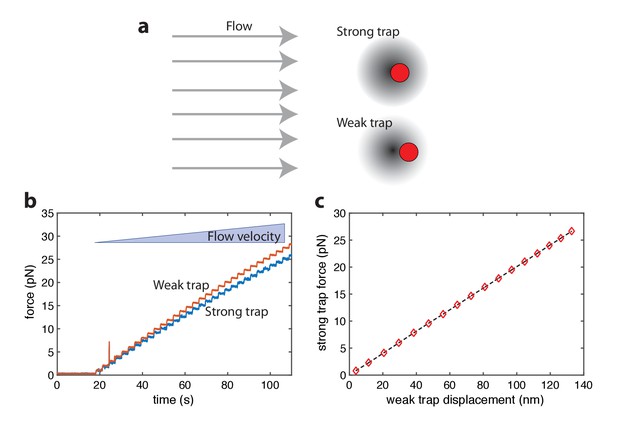
Linearity of the optical traps at large forces.
(a) To verify that all force measurements are in the traps’ linear regime, two similar beads are simultaneously trapped in a weak (k ~ 0.2 pN/nm) and strong (k ~ 0.75 pN/nm) trap, respectively. A flowing buffer creates a Stoke’s flow on the trapped beads, and thus a displacement that depends on the traps’ stiffness. Since the displacement of the bead in the stronger trap is small (<35 nm) the force measurement is assumed to be in the linear regime, and enables to check the linearity of the weak trap, where the bead’s displacement is larger. (b) Calculated forces, based on the beads’ displacement and the traps’ calibrated stiffness. The difference in slope stems from the variability (~10%) in the size of the beads. (c) The force, measured using the strongly trap bead, remains linear (within 1%) with the displacement of the weakly trapped bead up to the measured displacement of ~ 140 nm.
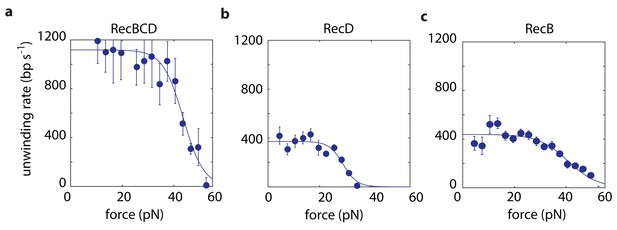
Force-velocity curves for RecBCD and its subunits in complex at 2 mM ATP.
Force velocity curves at [ATP] = 2 mM for (a) RecBCD, (b) RecD and (c) RecB. Data shown as mean ± s.e.m.; the number of experiments is listed in Supplementary file 4. Lines through the data are best fits to Equation 1.
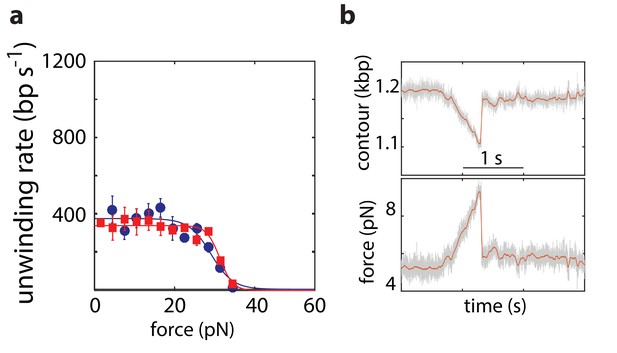
The secondary translocase of RecB does not affect the force-velocity measurements of RecD.
(a) Force-velocity curves on the asymmetric construct probing RecD, for RecBCD (blue, data similar to Figure 2d) and RecBK29QCD (red), at [ATP] = 2 mM. Data shown as mean ± s.e.m., lines through the data are best fits to Equation 1. The number of experiments is listed in Supplementary file 4. (b) Representative trace for RecBC activity on the symmetric construct. RecBC’s secondary translocase can sustain forces up to 8.3 ± 1.2 pN (mean ± s.e.m.). Raw data is shown in grey, filtered data (fc = 250 Hz) is shown in red.
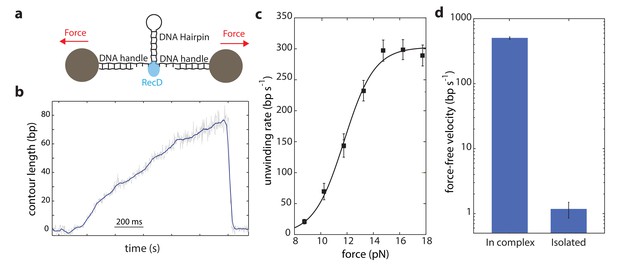
Unwinding of a hairpin under tension by the isolated RecD.
(a) Schematic representation of RecD unwinding in optical tweezers. (b) Representative unwinding trace. Raw data is shown in grey, filtered data (fc = 250 Hz) is shown in blue. [ATP] = 2 mM. (c) Velocity-force curve for the isolated RecD at 2 mM ATP follows a sigmoidal-like behavior saturating at high forces. Data shown as mean ± s.e.m., number of traces in Supplementary file 4. The line through the data is a best fit to Equation S29, Supplementary file 3. (d) Force-free velocities for RecD, obtained from (c) and Figure 2b by extrapolating to F = 0, show ~ 2–3 orders of magnitude difference between the enzyme in-complex and the isolated one (Extrapolated result ± s.e.).
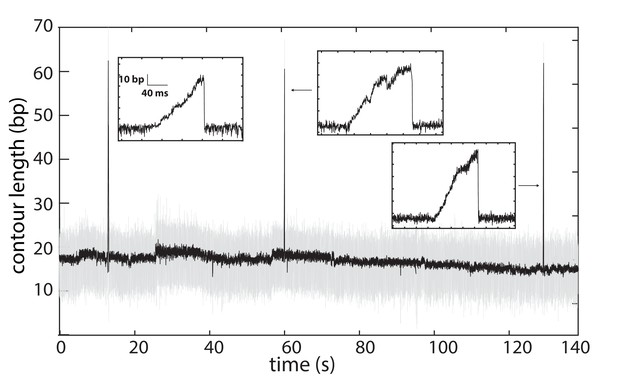
Multiple unwinding events by RecD occur on the same DNA molecular construct.
Contour-time trace for long timescales shows peaks of RecD activity. Zoomed-in windows indicate individual activity events of RecD followed by dissociation. [ATP] = 2 mM. Raw data is shown in grey, filtered data (fc = 250 Hz) is shown in black.
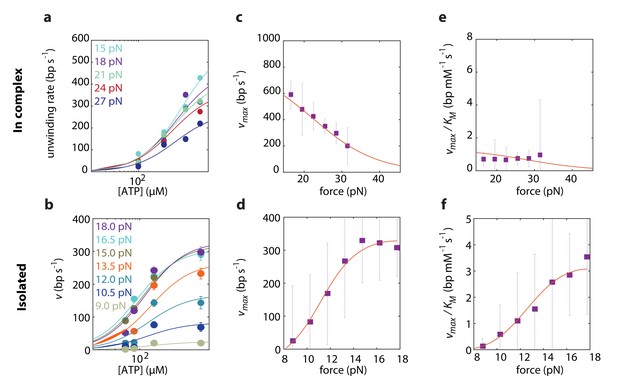
Force dependent Michaelis-Menten curves for RecD in complex
(a) and isolated (b). Data shown as mean ± s.e.m. Lines through the data are the result of a global fit to a kinetic model of a Brownian ratchet before ATP binding (Equations S7 and S8 in Supplementary file 2 and Equations S19 and S20 in Supplementary file 3). Different colors indicate different forces. (c–d). Force-dependent for RecD in complex (c) and isolated RecD (d). Discrete data points indicate as a result of fitting Michaelis-Menten curves to the data in a and b for each force separately (shown as fit result ± s.e.). Lines through the data indicate the results of the global fitting. (e–f) Force dependent for RecD in complex (e) and isolated (f). Discrete data points indicate as a result of fitting Michaelis-Menten curves to the data in a and b for each force separately (shown as fit result ± s.e.). Lines through the data indicate the results of the global fitting.
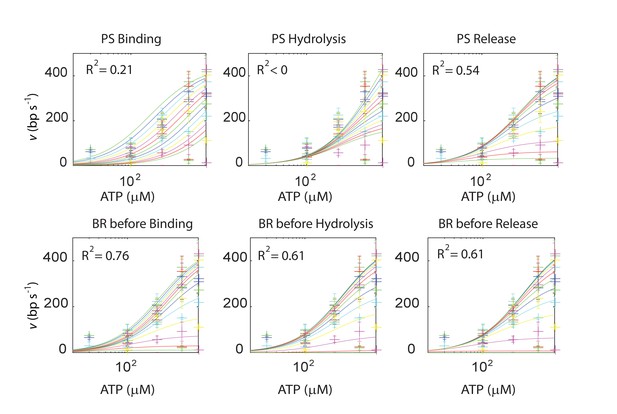
Global fits for different translocation mechanisms of in-complex RecD under force.
Different colors indicate different forces (Materials and methods). Data shown as mean ± s.e.m. Lines show the results of fitting the data with the kinetic models described by Equations S1-S12, Supplementary file 2.
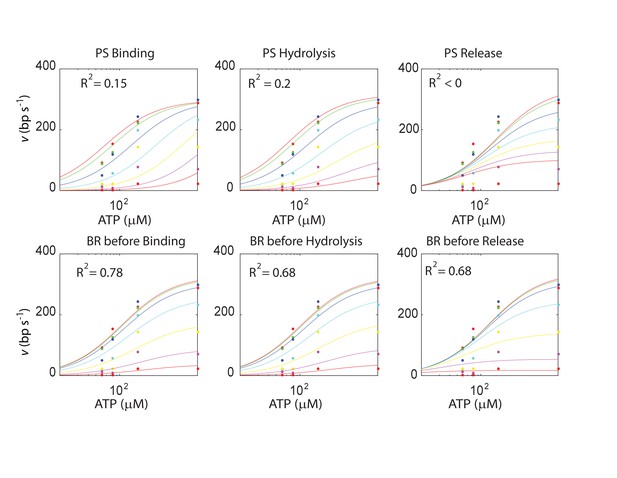
Global fits for different translocation mechanisms of isolated RecD under force.
Different colors indicate different forces (Materials and methods). Data shown as mean ± s.e.m. Lines show the results of fitting the data with the kinetic models described by Equations S13-S24, Supplementary file 3.
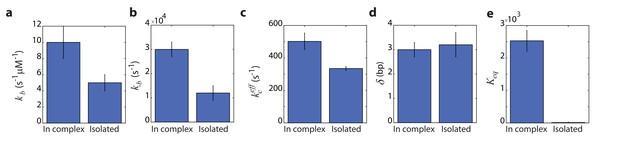
The global fitting parameters reveal a shift towards the post translocation state of RecD when in complex.
(a) Forward ATP binding rate, . (b) Backward binding rate, . (c) effective catalytic rate, . (d) Step size, . (f) Equilibrium constant of translocation, shifted by the complex toward the post-translocation state.
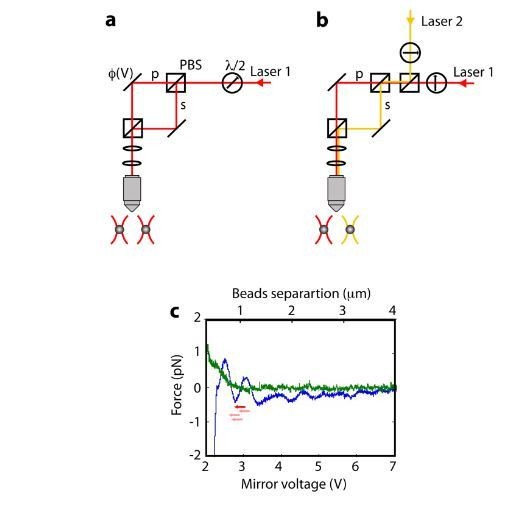
Effect of the trap-trap interference at short distances.
A. In our single-laser, dual-trap optical tweezers setup the two traps are created by splitting a single laser beam into two orthogonally polarized beams, using a polarizing beam splitter (PBS). After being reflected by steerable and fixed mirrors, respectively, the two beams are combined by a second PBS and directed to the focusing objective. Combined with differential detection, this setup offers a high degree of stability, since laser fluctuations are common to both traps and therefore “rejected”. However, the use of the orthogonal polarizations of a single laser with a high numerical objective is known to result in interference between the beams, and thus spurious position-dependent force signals. B. To assess the magnitude of this effect, we introduced a second laser, thus producing the traps by two mutually incoherent beams. C. Measurements with a single laser (blue) display a characteristic wavelength-scale modulation as the traps are brought into proximity by reducing the voltage on the steerable mirror, while two-lasers measurements are devoid of this modulation. The error in the force signal in the relevant position range (red arrow) is estimated as ± 0.5 pN for an individual trace. Due to the variability in bead size, there is some variability in the absolute range probed by different experiments (examples are shown in pink arrows), and thus the errors in average forces, as reported in the manuscript, are even smaller and have no significant effect in our measurements.
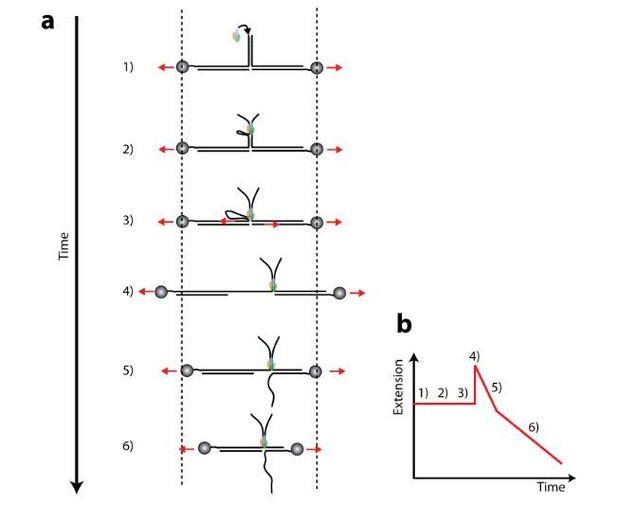
Additional files
-
Supplementary file 1
All the possible mechano-chemical models.
- https://doi.org/10.7554/eLife.40836.013
-
Supplementary file 2
Force-dependent Michaelis-Menten parameters for the different models of unwinding, for experiments measuring activity against an opposing force.
- https://doi.org/10.7554/eLife.40836.014
-
Supplementary file 3
Force-dependent Michaelis-Menten parameters for the different models of unwinding, for experiments measuring unwinding of a hairpin under tension.
- https://doi.org/10.7554/eLife.40836.015
-
Supplementary file 4
Number of traces measured
- https://doi.org/10.7554/eLife.40836.016
-
Supplementary file 5
Oligonucleotides used for synthesizing DNA substrates for optical tweezers experiments.
- https://doi.org/10.7554/eLife.40836.017
-
Supplementary file 6
Results of fitting RecD force velocity curves to a Brownian ratchet before hydrolysis model, in complex and isolated.
- https://doi.org/10.7554/eLife.40836.018
-
Transparent reporting form
- https://doi.org/10.7554/eLife.40836.019