Distinct RanBP1 nuclear export and cargo dissociation mechanisms between fungi and animals
Figures
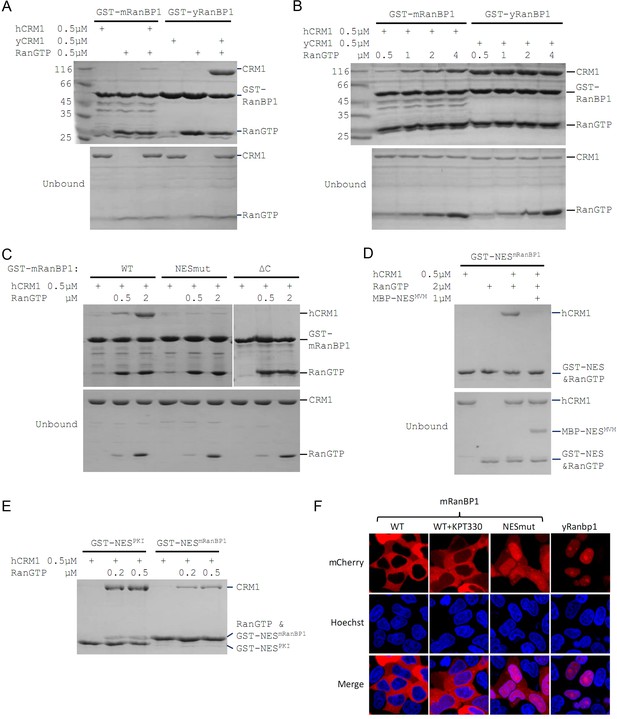
Mouse RanBP1 forms a nuclear export competent complex with hCRM1 only through its NES, and in a RanGTP concentration dependant manner.
(A, B, C) GST tagged mouse/yeast RanBP1 or mutants pull down of CRM1 in the presence of RanGTP. (A) While yeast proteins form a strong RanBP1-RanGTP-CRM1 complex, the animal proteins form a RanBP1-RanGTP complex with very weakly bound CRM1. (B) At increasing concentration of RanGTP, while yeast complex is not affected, animal CRM1 is increasingly bound to GST-mRanBP1-RanGTP. (C) Mutation (NESmut) or deletion of the NES of mRanBP1 (ΔC) abolishes hCRM1 binding at either high or low RanGTP concentration. (D) GST-NESmRanBP1 pull down of CRM1 in the presence of RanGTP and NES inhibitor MBP-NESMVM. NES of mRanBP1 binds to hCRM1 in the presence of RanGTP and is effectively outcompeted by MBP-NESMVM, suggesting that the NES of mRanBP1 binds to NES groove of CRM1. (E) GST-NES pull down of hCRM1 and different concentration of RanGTP to show that the NES of mRanBP1 is weaker than the well-studied NES of PKI cargo. (F) mCherry tagged different RanBP1 constructs were transfected into HeLa cells and treated with or without 5 µM CRM1 inhibitor KPT-330 for 3 hr. While mRanBP1 is exclusively cytoplasmic, mRanBP1 treatment with KPT-330 and NESmut are significantly re-localized to the nucleus. yRanBP1 is exclusively nuclear.
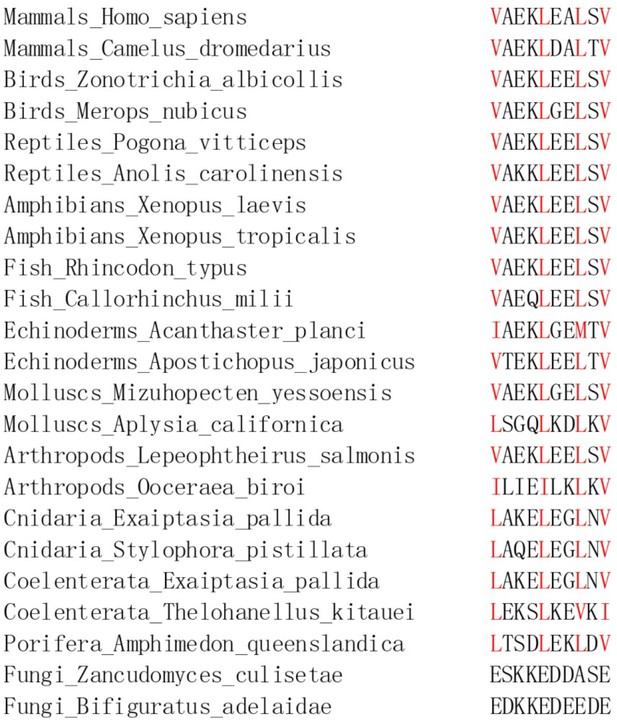
NES of RanBP1 is conserved in animals but does not exist in fungi.
hRanBP1 is blasted against different domain or phylums using BLASTp. The NES in the most and the least conserved RanBP1 from each domain or phylum are shown. Only one RanBP1 sequence is available in PubMed for Porifera phylum and RanBP1 is not found for a couple of phyla. Letters in red highlight hydrophobic residues that constitute the NES.
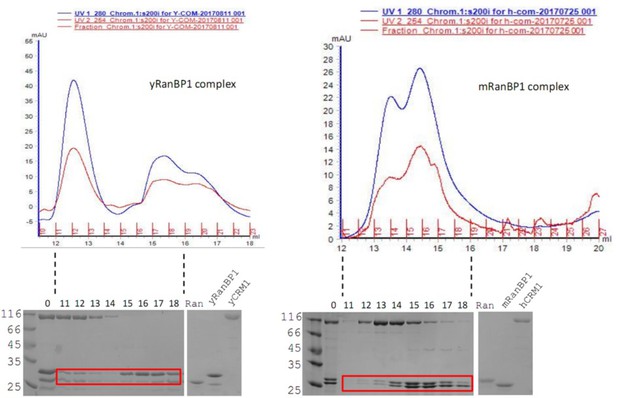
In excess of RanBP1, mRanBP1, hRanGTP and hCRM1 do not form complex like yeast proteins.
While yeast proteins form a stable trimeric complex (left, two Ran-RanBP1 peaks), mRanBP1 and RanGTP do not co-elute with hCRM1 (right, one Ran-RanBP1 peak).

GST-mRanBP1 pull down of hCRM1 in the presence of RanGTP or RanGDP.
The complex is formed only in the presence of RanGTP.
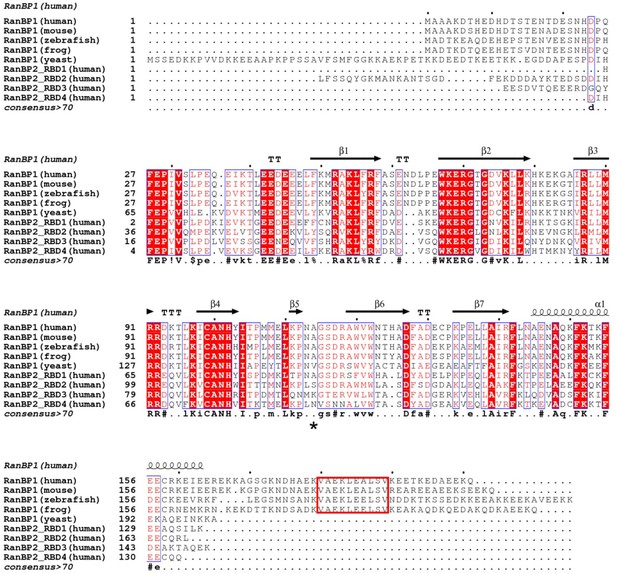
Structure based sequence alignment of human, mouse, zebrafish, frog and yeast RanBP1 and 4 RBDs from human RanBP2.
Secondary structure is shown on top of alignment and the consensus is shown below the alignment. Human A114 is indicated with a * below the alignment. NES is labeled in red box.
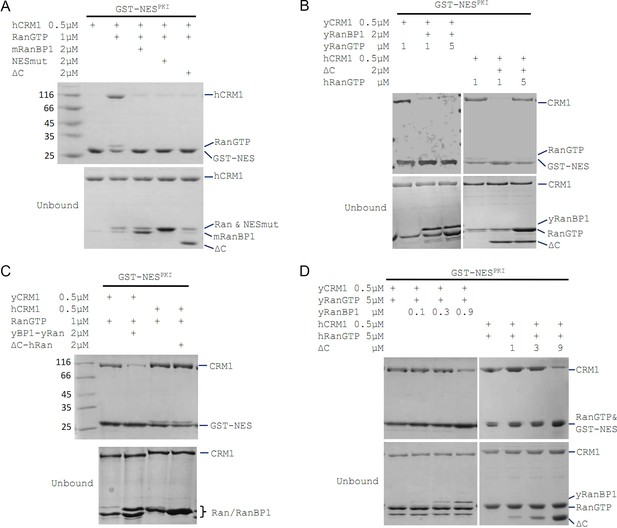
mRanBP1’s RBD dissociates CRM1 cargo through sequestering RanGTP.
(A–D) GST-NESPKI pull down of CRM1 and RanGTP in the presence of RanBP1 or its mutants. (A) NESmut or ΔC dissociates cargo as WT RanBP1. (B) High concentration of RanGTP inhibits cargo dissociation in animals but not yeast. (C) RanGTP-bound ΔC loses its ability in cargo dissociation while yeast RanGTP-RanBP1 remains competent. (D) ΔC dissociates cargo when its concentration is higher than that of RanGTP, and yRanBP1 dissociates cargo when its concentration is higher than that of CRM1.
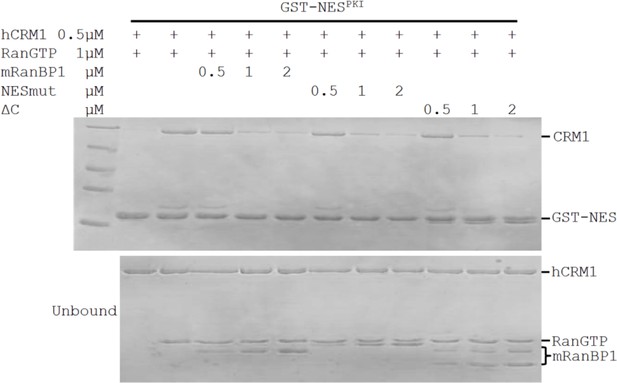
GST-NES pull down shows that NESmut or ΔC is as potent as WT RanBP1 in dissociating CRM1 complex.
There is no obvious cargo dissociation difference among RanBP1 constructs for each concentration tested.
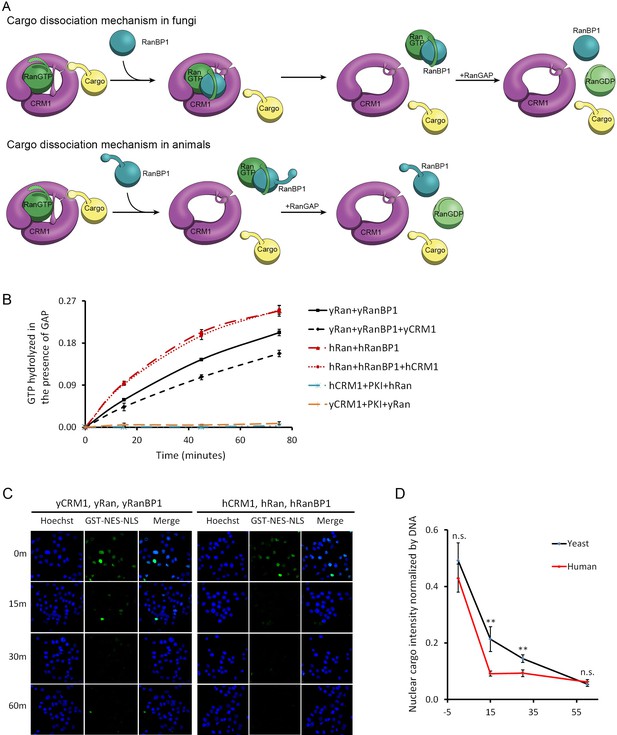
CRM1 cargo dissociation mechanism in fungi and animals.
(A) In fungi, cargoes are actively released from CRM1’s NES groove by formation of RanBP1-RanGTP-CRM1 trimeric complex. RanGTP-RanBP1 transiently released from CRM1 is catalyzed by RanGAP. In animals, RanBP1 strips RanGTP from CRM1, resulting in dissociation of NES cargo from CRM1. RanGAP catalyzes further dissociation of Ran-RanBP1. (B) GAP mediated RanGTP hydrolysis (arbitrary unit) at different time points. CRM1 and NES (PKI) inhibit RanGTP hydrolysis in both human (cyan) and yeast (orange). Addition of RanBP1 increases the rate of GAP hydrolysis. While addition of yCRM1 partially inhibit GAP mediated hydrolysis, addition of hCRM1 does not. Error bars represent standard deviation of quadruple repeats. (C) In vitro nuclear export of GST cargo in the presence of human or yeast CRM1/Ran/RanBP1 using semi-permeabilized HeLa cells. The reactions were stopped at different time points and level of nuclear cargo was stained with anti-GST antibody. (D) Quantification and statistical analysis of nuclear cargo intensity after normalization by DNA intensity. Error bars represent standard error of measurements for each set of data containing measurements from at least 30 cells. ** denotes p<0.01.
-
Figure 3—source data 1
Nuclear export in the presence of human or yeast proteins using semi-permeablized cells.
- https://doi.org/10.7554/eLife.41331.012
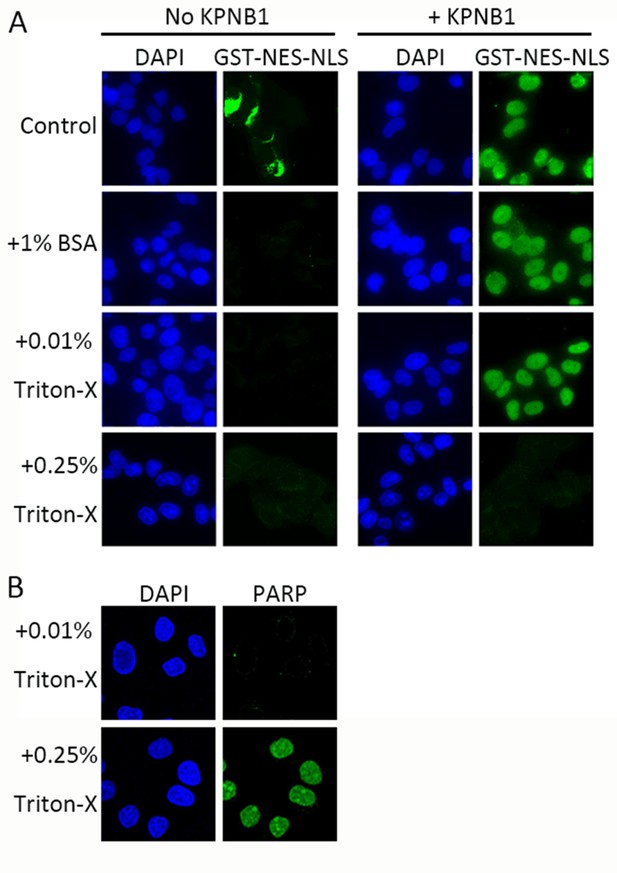
Low concentration of Triton-X prevents non-specific cytoplasmic binding and does not permeate the nuclear envelope.
(A) GST-NES-NLS was stained with anti-GST antibody. Control samples do not contain any reagents to prevent non-specific contamination. Images were collected at the same level of excitation and processed equally. 0.01% Triton-X works slightly better than 1% BSA in our hands. (B) PARP (poly [ADP-ribose] polymerase) could be stained when the cells were incubated in the presence of 0.25% Triton-X, but not 0.01% Triton-X.
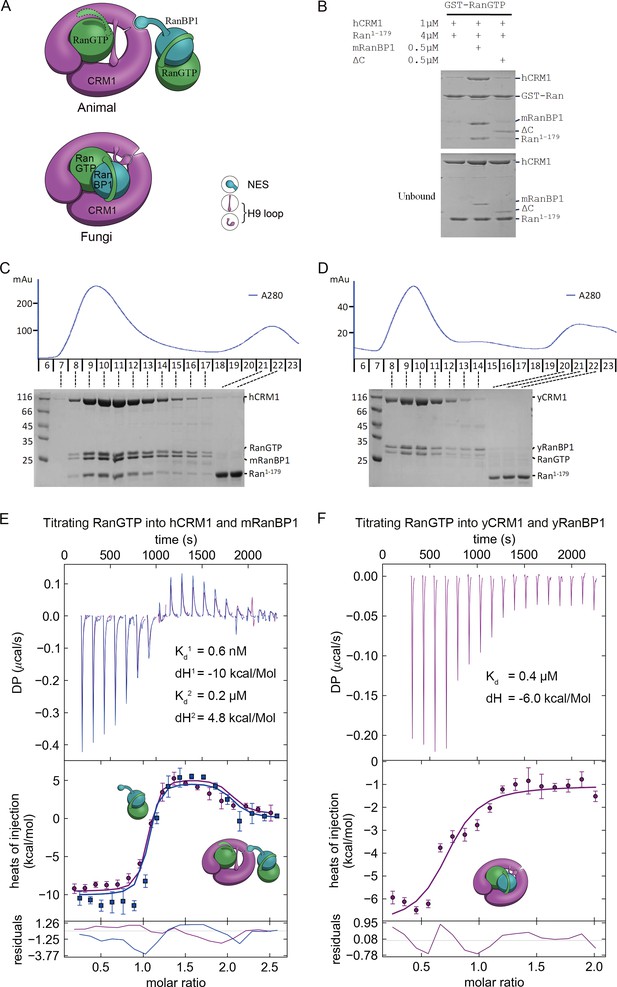
Animal RanBP1, RanGTP and CRM1 form a tetrameric complex containing two RanGTP proteins.
(A) Model of animal and fungi RanBP1 nuclear export complexes. Yeast complex contains one RanGTP and animal complex contains two. Unlike yeast, animal RBD does not contact CRM1 and animal CRM1 has different conformation of NES groove and H9 loop. (B) GST-RanGTP pull down of Ran1-179 and CRM1 in the presence of mRanBP1, ΔC or buffer. Ran1-179 is bound when WT mRanBP1 is added, but not when ΔC is added. Size exclusion profiles and SDS-PAGE analysis of the peaks by animal (C) and yeast (D) RanBP1, RanGTP, RanGTP1-179 and CRM1 proteins mixed at 1:1:3:1 molar ratio. The first peak contains four bands for animal sample and three bands for yeast samples. ITC titration of RanGTP into animal (E) and yeast (F) CRM1-RanBP1 respectively. Figure E and F include two and one independent titration experiments, respectively. Global or single fit of Kd and ΔH is displayed in the figure respectively. Error bars represents 95% confidence interval of measurements. While animal proteins display an exothermic phase and an endothermic phase, yeast proteins only produce an exothermic phase.
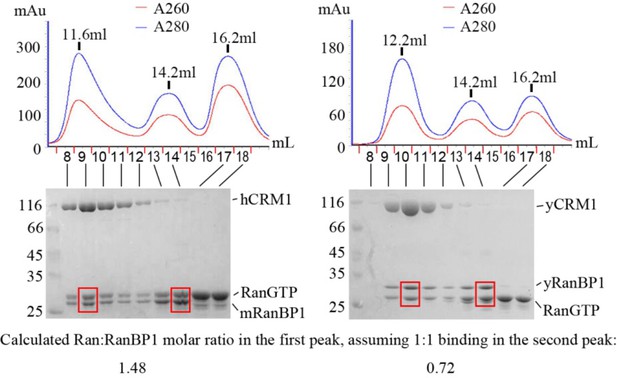
Size exclusion peaks of RanBP1, RanGTP and CRM1 from yeast or animals mixed at 2:5:1 molar ratio and SDS-PAGE analysis of different peaks.
The higher ratio of A260/A280 in the third peak indicates nucleotide bound Ran, which is in excess. Molar ratio of co-eluted Ran:RanBP1 in the first peak (fraction 9 or 10, boxed in red) is normalized by the corresponding second peak (fraction 14, boxed in red), assuming Ran:RanBP1 ratio in the second peak is 1:1. However, Ran-RanBP1 peaks (second peak) may be contaminated with excess of Ran, and it is known that for yeast proteins, the molar ratio of Ran to RanBP1 in first peak should be 1. After further normalizing animal RanGTP:RanBP1 molar ratio with that of yeast, a value of 2.05 (1.48/0.72) was obtained for animal Ran:RanBP1 ratio in the first peak, suggesting a tetrameric complex with two RanGTP proteins.
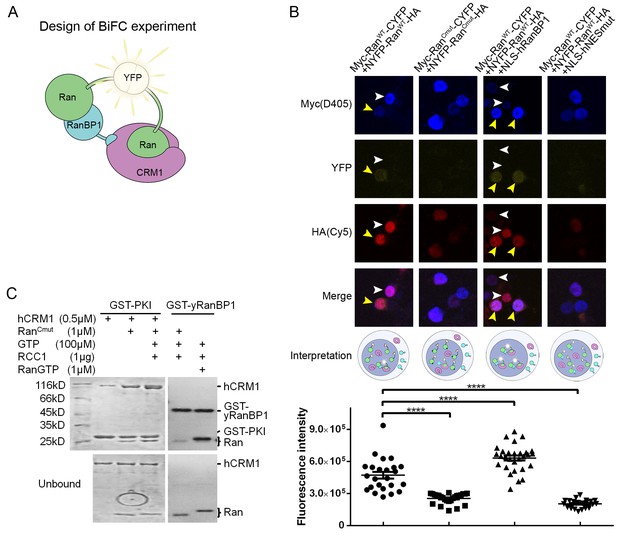
RanBP1 tetrameric complex exists in the cell nucleus.
(A) Design of BiFC experiment. When the RanBP1 tetramer is formed, a YFP signal could be detected. This assay may not discriminate which Ran binds to RanBP1 (or CRM1) in the tetramer. (B) Representative immunofluorescence (HA and Myc) and fluorescence (YFP) images on cells co-transfected with fusion plasmids (labelled on top). NLS-hRanBP1 or NLS-hNESmut (hNESmut: human RanBP1 with NES mutation) was used to increase nuclear level of RanBP1 or its mutant. Nucleus was not stained so that the two transfected proteins could be stained without contaminating the YFP channel. The boundary of nucleus could be estimated by Ran fusions, which is mainly localized in the nucleus (see Figure 5—figure supplement 3). Results interpretation (middle panel) explains why the yellow fluorescence is observed or not. Bottom panel shows the nuclear YFP intensities of at least 20 cells (only florescent cells transfected with two Ran fusions) in the corresponding samples under the same level of illumination. **** denotes p<0.0001. (C) RanCmut is able to bind to CRM1 and NES (left panel) but not able to bind yRanBP1 (right panel).

Pull down of 293 T cells expressed Ran fusion proteins using GST-mRanBP1 or GST, in the presence or absence of Ran knock down.
Input lanes were used to ensure similar amount of Ran fusions present in each pull down sample. In the presence of Ran knock down, Ran fusions bound more to RanBP1, functionally rescues the reduction of bound RanWT.
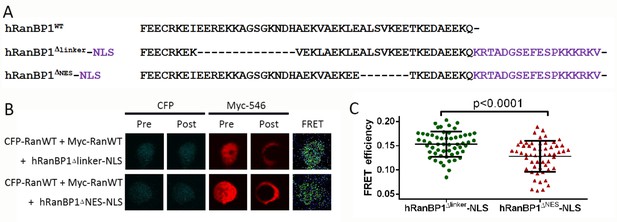
Acceptor photobleach-FRET and statistical analysis of FRET efficiencies.
293 T cells were seeded in 24-well plates containing circular coverslips slides. After adhesion overnight, cells were transfected with Myc-Ran and CFP-Ran plasmids in addition to HA-tagged hRanBP1Δlinker-NLS or hRanBP1ΔNES-NLS (panel A, showing only the C terminus). Pull down experiments verified that RanBP1 tetramer formation is possible for hRanBP1Δlinker-NLS but not hRanBP1ΔNES-NLS. 24 hr after transformation, cells were fixed and stained with primary antibodies against Myc (ProteinTech, Mouse) and HA (CST, Rabbit) separately. The slides were then incubated with the secondary antibody anti-Mouse-Alexa Fluor 546 (ThermoFisher) and anti-Rabbit-Cy5 (ThermoFisher). FRET measurements were performed by acquiring pre- and post- acceptor bleach images of donor and acceptor using the Olympus FV-1000 acceptor photobleach FRET, followed by calculation of FRET efficiency for acquired images (B). Only cells transfected with two Ran plasmids and one RanBP1 plasmid were analyzed. Statistical significance (C) was calculated using two-tailed unpaired Student’s t test. Each group contains more than 40 cells.
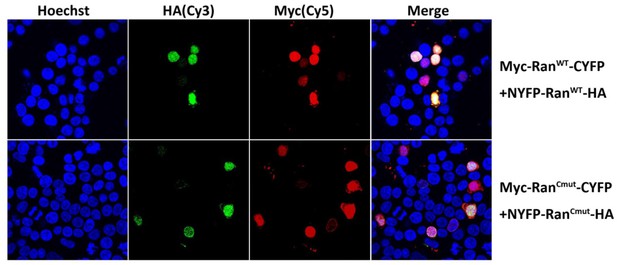
Immunofluorescence (HA and Myc) on cells co-transfected with two fusion plasmids.
All the four Ran fusion constructs are mainly localized in the cell nucleus, similar as RanWT.
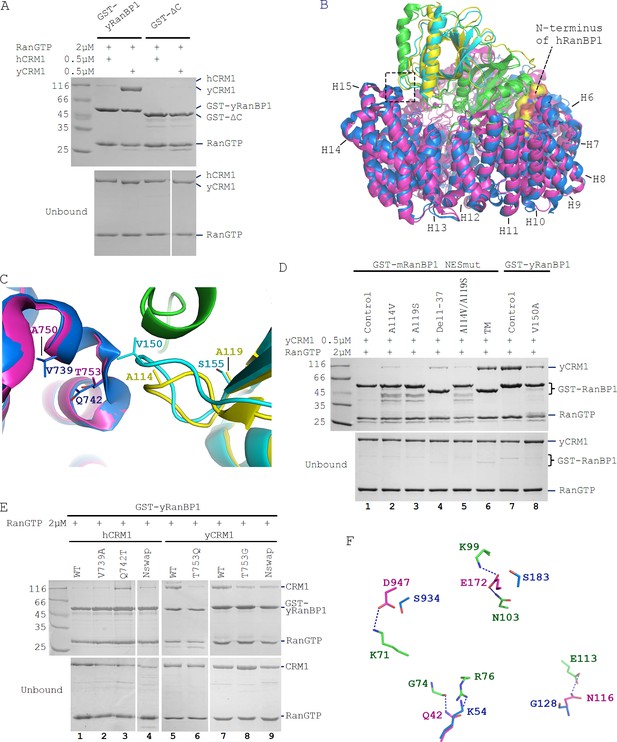
Sequence deviation of RanBP1 and CRM1 from their yeast orthologs prevents binding between RanBP1-RanGTP and CRM1 in animals.
(A) Cross-species pull down of CRM1 using immobilized GST-yRanBP1 or GST-ΔC in the presence of RanGTP. (B) Superimposition of hRanBP1-hRan (1K5G, yellow and green) and hCRM1 (3GB8, blue) onto yRanBP1(cyan)-hRan(green)-yCRM1(magenta) crystal structure (4HAT). N-terminus of hRanBP1 (surface representation) is located between intimately packed RanGTP and CRM1 and thus possibly hinders their binding in animals. Heat repeats 6–15 (H6–H15) of CRM1 are labelled. (C) Zoom in of boxed region in 6B shows RanBP1-CRM1 interface and adjacent residues (shown as sticks) that are different between species. (D) GST-RanBP1 and its mutants pull down of yCRM1 and RanGTP. TM contains mutations A114V, A119S and Δ1–37, in addition to NES mutation. A114V and N-terminal deletion collectively rescue binding to yCRM1-RanGTP. (E) GST-yRanBP1 pull down of human and yeast CRM1 mutants in the presence of RanGTP. Both RanBP1 and Ran interacting regions of CRM1 are important for trimeric complex formation. (F) In the yRanBP1-hRan-yCRM1 crystal structure, yCRM1 and hRanGTP residues that form hydrogen bonds (dash lines) are displayed as magenta sticks. The structurally non-conserved hCRM1 residues are displayed as blue sticks. To improve clarity, main chain atoms (except glycine) and conserved residues are omitted.
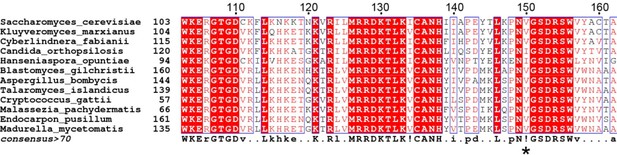
Multiple sequence alignment of fungi RanBP1 proteins to show that S.
cerevisiae V150 (marked with *) is well conserved to be V or I.
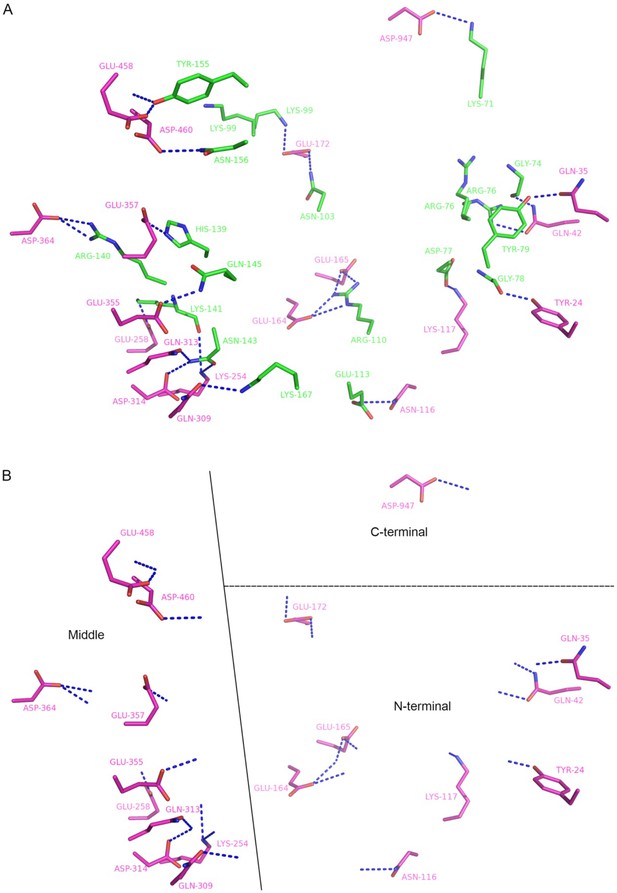
Residues that form hydrogen bonds (blue dash) between hRanGTP (green) and yCRM1 (magenta) in 4HAT structure.
(A) Interacting residues are displayed as sticks with main chain atoms removed except those form hydrogen bonds. (B) yCRM1 interacts with hRan using three regions: N terminal, middle and C terminal regions. Only yCRM1 residues and hydrogen bonds in S7A are shown.
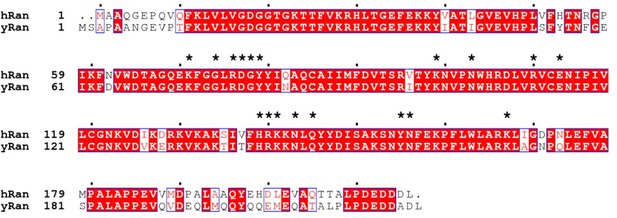
Sequence alignment of human and yeast Ran.
The hRan residues that electrostatically interact with yCRM1 are labelled with a * above the alignment.
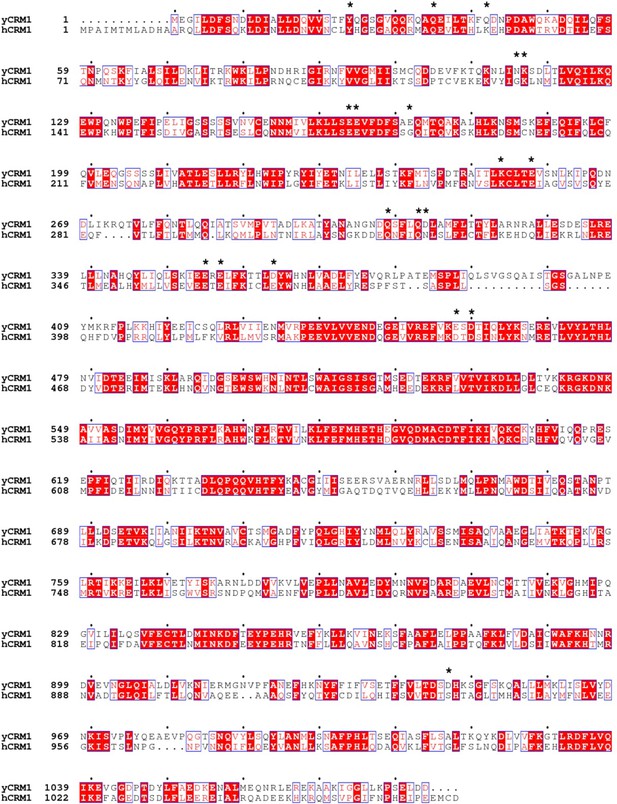
Sequence alignment of human and yeast CRM1.
The yCRM1 residues that electrostatically interact with hRan are labelled with a * above the alignment.
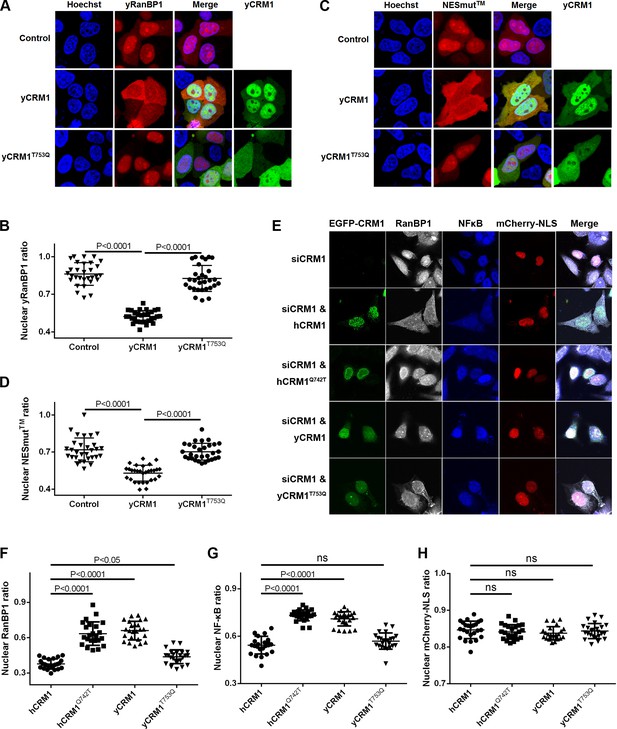
Residues that play important roles in RanBP1 localization and nuclear export of cargo.
(A) mCherry tagged yRanBP1 was transfected alone or co-transfected with EGFP-yCRM1 or EGFP-yCRM1T753Q mutant, and visualized under microscope. (B) Quantification and statistical analysis of yRanBP1 localization in 7A. Ratio of nuclear yRanBP1 for each cell is calculated as its nuclear intensity divided by total cellular intensity. Error bars represent standard deviation of each set of data containing measurements from at least 30 cells. (C) mCherry tagged NESmut was transfected alone or co-transfected with EGFP-yCRM1 or EGFP-yCRM1T753Q mutant, and visualized under microscope. (D) Quantification and statistical analysis of NESmut localization in 7C. (E) Subcellular localization of endogenous RanBP1, NFκB and transfected mCherry-NLS under the treatment of siCRM1 and transfection of different EGFP-CRM1 constructs. mCherry-NLS is constructed as mCherry-NESPKI-MBP-NLSSV40 in pmCherry-C1 plasmid. (F–H) Quantification and statistical analysis of nuclear ratio of RanBP1, NFκB and mCherry-NLS in 7E.
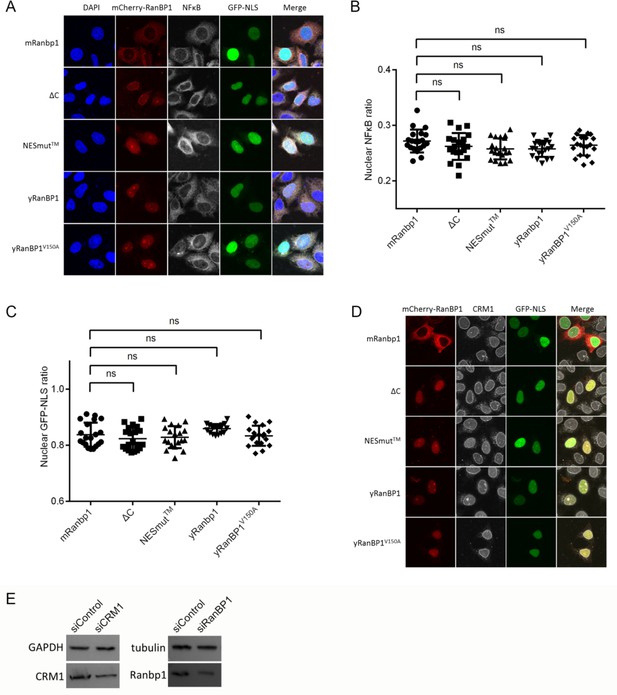
siRanBP1 does not change the subcellular localization of endogenous CRM1, NFκB and transfected GFP-NLS.
(A) Subcellular localization of endogenous NFκB and the transfected GFP-NLS (constructed as GFP-NESPKI-MBP-NLSBPSV40) in the presence of siRanBP1 and transfection of different mCherry-RanBP1 constructs. (B–C) Quantification and statistical analysis of nuclear ratio of NFκB and GFP-NLS in A. (D) Subcellular localization of endogenous CRM1 and transfected GFP-NLS in the presence of siRanBP1 and transfection of different mCherry-RanBP1 constructs. (E) Western analysis shows that siCRM1 and siRanBP1 are effective.
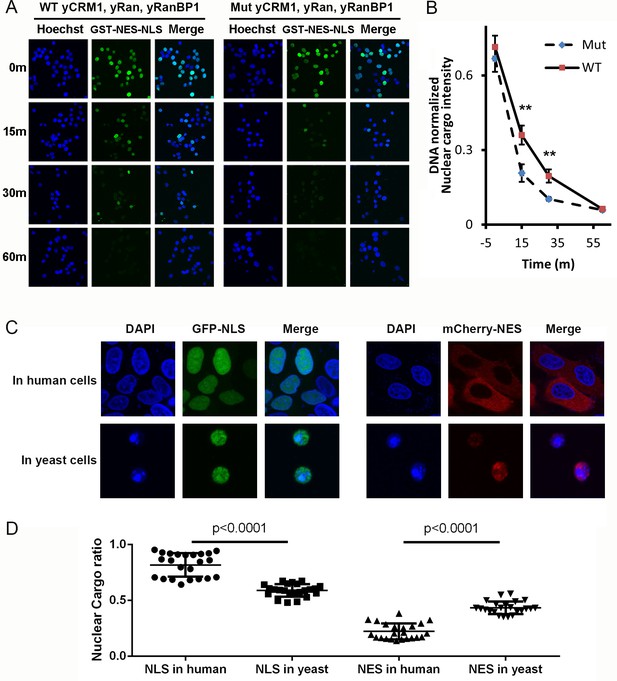
Mammalian nuclear transport system has higher efficiency.
(A) Nuclear export of GST-NES-NLS in the presence of yCRM1/yRan/yRanBP1 or their mutants (yCRM1T753Q, yRan, yRanBP1V150A and fused with mRanBP1’s NES). Protocol used in this experiment is similar as in Figure 3C. (B) Quantification of nuclear cargo intensity normalized by DNA in Figure 7E. Shown also includes unpaired student t-test between WT and mutant samples at each time point. Error bars represent standard error of measurements for each set of data containing measurements from at least 23 cells. Only 15 m and 30 m samples display statistical significances (** denotes p<0.01). (C) Representative images of GFP-NLS and mCherry-NES localization in human (HeLa) and yeast cells (W303.1a). pRS416 (for yeast transfection), pEGFP-C1 and pmCherry-C1 (for human transfection) plasmids were used to express fluorescent tagged NES or NLS proteins. mCherry-NES is constructed as mCherry-NESPKI-MBP-NLSSV40. GFP-NLS is constructed as GFP-NESPKI-MBP-NLSBPSV40. (D) Quantification and statistical analysis of nuclear cargo ratio in 8C.
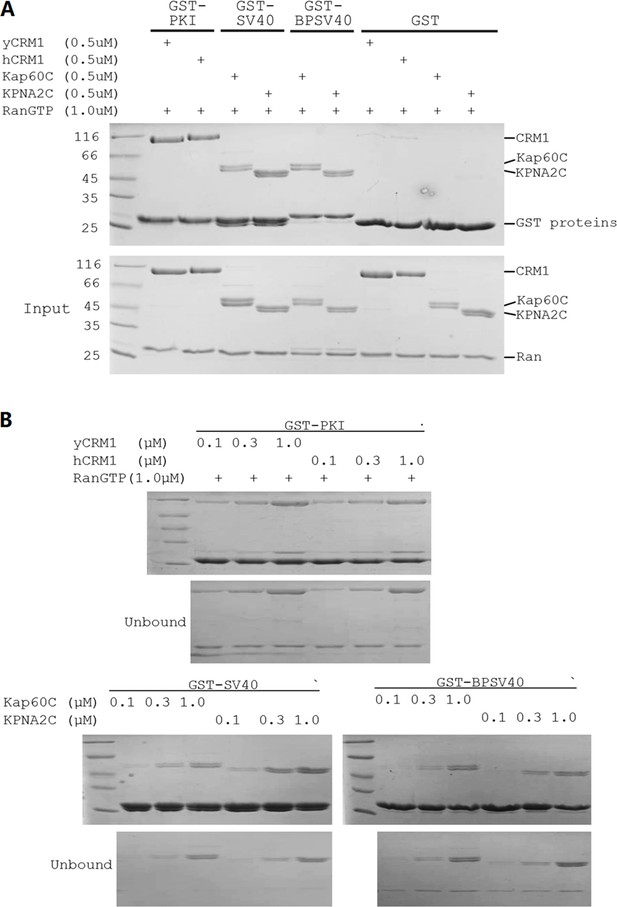
The NLS/NES used displayed similar affinities to yeast or human transport receptors.
(A) Pull down shows that NES, NLS SV40 and NLSBPSV40 (that were used for Figure 8C) bind the yeast and human transport receptor with comparable efficiency. Kap60C and KPNA2C are the yeast and human importin α proteins with their N-terminal importin β binding (IBB) domains deleted. BPSV40 is same as reported in Hodel et al. (2001). (B) The different pull downs were performed at more concentrations to compare binding strength. More cytoplasmic localization of mCherry-NLS in human in theory could be due to tighter binding to CRM1 or weaker binding to human importin α (KPNA2). However, we showed that neither is true. In fact, SV40NLS binds to human importin α slightly stronger. Similarly, GFP-NLS localization difference was also not due to differences in binding to the transport receptors.
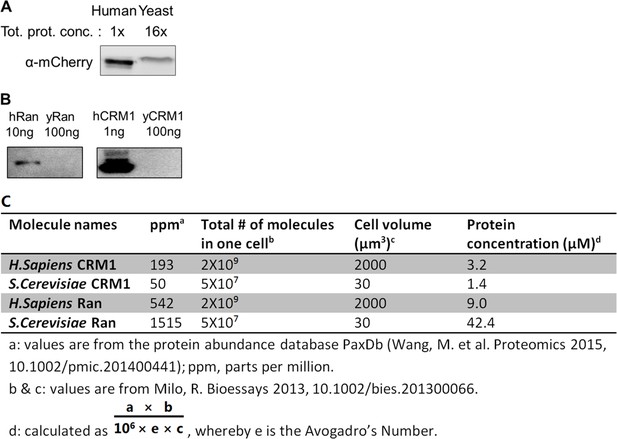
Yeast mCherry-NES-NLS were expressed at very low level compared to human cells.
(A) Yeast sample was loaded 16x more than human sample but the yeast protein band intensity is still less than half of human protein band. Tot. proti. conc.: total protein concentration quantified by bicinchoninic acid assay (BCA). (B) antibodies against human Ran and CRM1 were not reactive against the yeast proteins. Proteins were purified from E. coli. (C) Estimated CRM1 and Ran concentrations in yeast and human cells.
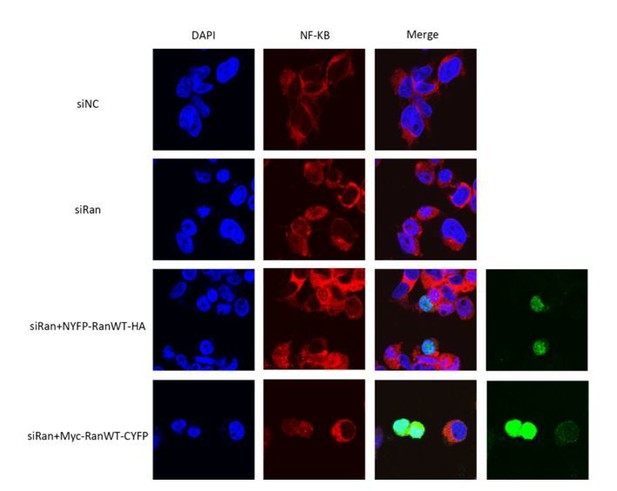
Nuclear export of NFκB in the presence of Ran knock down and rescues with Ran fusions.
Tables
Reagent type (species) or resource | Designation | Source or reference | Catalogue no. | Additional information |
---|---|---|---|---|
Genetic reagent (H. sapiens) | pcDNA 3.1(+) myc-RanWT-CYFP | this study | Used in BiFC | |
Genetic reagent (H. sapiens) | pcDNA 3.1(+) NYFP-RanWT-HA | this study | Used in BiFC | |
Genetic reagent (H. sapiens) | pcDNA 3.1(+) myc-RanCmut-CYFP | this study | Used in BiFC | |
Genetic reagent (H. sapiens) | pcDNA 3.1(+) NYFP-RanCmut-HA | this study | Used in BiFC | |
Genetic reagent (H. sapiens) | pcDNA 3.1(+) CFP-RanWT | this study | Used in FRET | |
Genetic reagent (H. sapiens) | pcDNA 3.1(+) myc-RanWT | this study | Used in FRET | |
Genetic reagent (H. sapiens) | pcDNA 3.1(+) hRanBP1Δlinker-NLS | this study | Used in FRET | |
Genetic reagent (H. sapiens) | pcDNA 3.1(+) hRanBP1ΔNES-NLS | this study | Used in FRET | |
Genetic reagent (M. musculus) | pcDNA 3.1(+) NLS-mRanBP1 | this study | Used in BiFC | |
Genetic reagent (M. musculus) | pcDNA 3.1(+) NLS-NESmut | this study | Used in BiFC | |
Antibody | Rabbit anti-HA | Cell Signalling | 3724 | |
Antibody | Mouse anti-Myc | ProteinTech | 60003–2-Ig | |
Antibody | Alexa 647 labelled Anti-Rabbit IgG | ThermoFisher | A-21244 | |
Antibody | Dylight 405 labelled Anti-mouse IgG | Beyontime | A0609 | |
Antibody | Anti-GST | Santa Cruz | sc-138 | |
Antibody | Anti-RanBP1 | ProteinTech | 27804–1-AP | |
Antibody | Anti-CRM1 | absin | 115104 | |
Antibody | Anti-NFκB | SAB | 48676–1 | |
Chemical compound | Digitonin | Abcam | ab141501 |
Additional files
-
Transparent reporting form
- https://doi.org/10.7554/eLife.41331.029