Quantitative insights into the cyanobacterial cell economy
Figures
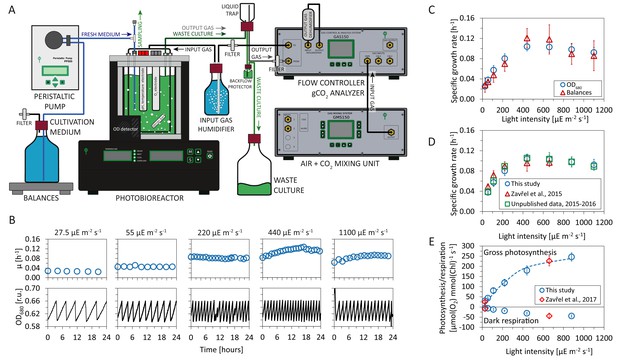
Experimental setup and evaluation of Synechocystis sp. PCC 6803 (substrain GT-L) phenotype stability.
Panel A: Photobioreactor setup. Cultures were cultivated in a flat-panel photobioreactor vessel (400 mL) in a turbidostat regime according to Zavřel et al. (2015b). Dilution of actively growing culture was based on measurements of optical density at 680 nm (OD680). Inflow air and CO2 were mixed in a gas mixing unit, the sparging gas flow rate was controlled by a gas analyzing unit. Sparging gas was moistened in a humidifier and, after bubbling through the photobioreactor vessel, separated from the waste culture via a liquid trap. CO2 concentration in the output gas was measured by an infrared sensor according to Červený et al., 2009. All other parameters were set as described in Nedbal et al. (2008) and Červený et al., 2009. Panel B: Representative measurement of the OD680 signal (black lines) within a turbidostat cultivation under increasing red light intensity (supplemented with low intensity of blue light). Calculation of specific growth rates (blue circles) is detailed in Materials and methods. Calculation of uptake and refilling rates of selected nutrients (including Na, (N, S, Ca, Mg, P and Fe) during the turbidostat cultivation is detailed in Figure 1—source data 1 (the elemental composition of Synechocystis cells is based on data available in the literature). Panel C: Calculation of growth rates from the OD680 signal and from top loading balances that monitored depletion rate of a spare cultivation medium (source data are available in Figure 1—source data 2). Panel D: Comparison of specific growth rates using an identical experimental setup throughout four successive years 2013–2017 (source data are available in Figure 1—source data 3). Panel E: Rates of gross photosynthesis and dark respiration, measured as O2 evolution and consumption rates directly within the photobioreactor vessel throughout 5 min of light and dark periods in 2016–2017 (this study) and in 2015–2017 (Zavřel et al., 2017). The dashed line represents a P-I curve fit of data from this study according to Platt et al. (1980). Source data are available in Figure 1—source data 4. Figure 1C: n = 6–11, Figure 1D: n = 3–11, Figure 1E: n = 4–6. Error bars (Figure 1C–1E) represent standard deviations.
-
Figure 1—source data 1
Uptake and refilling rates of selected nutrients during the quasi-continuous cultivation.
- https://doi.org/10.7554/eLife.42508.003
-
Figure 1—source data 2
Source data for Figure 1C.
- https://doi.org/10.7554/eLife.42508.004
-
Figure 1—source data 3
Source data for Figure 1D.
- https://doi.org/10.7554/eLife.42508.005
-
Figure 1—source data 4
Source data for Figure 1E.
- https://doi.org/10.7554/eLife.42508.006
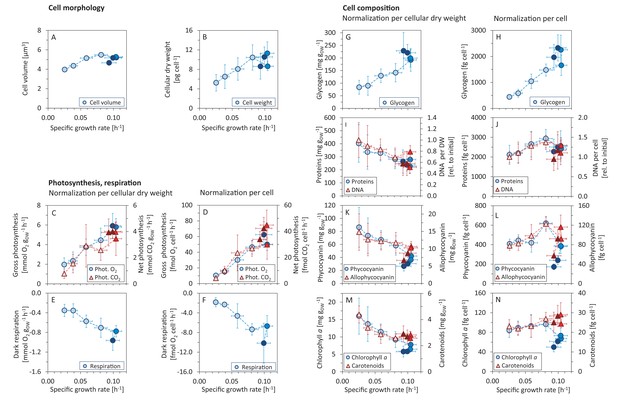
Variations in morphology and composition of Synechocystis cells with changing growth rate.
Under increasing light intensity and changing growth rate, the following parameters were estimated: cellular volume (A) and dry weight (B), gross photosynthesis (C, D) and dark respiration (E, F), and content of glycogen (G, H), proteins, DNA (I, J), phycobiliproteins (K, L), chlorophyll a and carotenoids (M, N). The data are plotted relative to cellular dry weight (C, E, G, I, K, M) as well as per cell (D, F, H, J, L, N). DNA content was normalized to its initial value after standardization per dry weight and per cell, the measurement was only semi-quantitative. All values represent averages from 3 – 11 independent biological replicates, error bars represent standard deviations. If error bars are not visible (panel A), the standard deviation was too small for visualization. Within each figure, data points are displayed in three different color shades to reflect (from bright to dark) light-limited, light-saturated and light-inhibited growth. Data plotted as a function of light intensity are available in Figure 2—figure supplement 1. Comparison with data available in the literature is summarized in Figure 2—source data 2.
-
Figure 2—source data 1
Source data for Figure 2.
- https://doi.org/10.7554/eLife.42508.009
-
Figure 2—source data 2
Comparison of the values measured in this study with data reported in the literature.
- https://doi.org/10.7554/eLife.42508.010
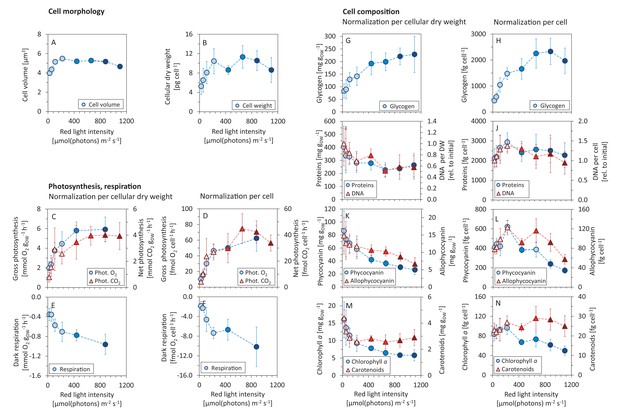
Allocation of key cellular resources as a function of light intensity.
https://doi.org/10.7554/eLife.42508.008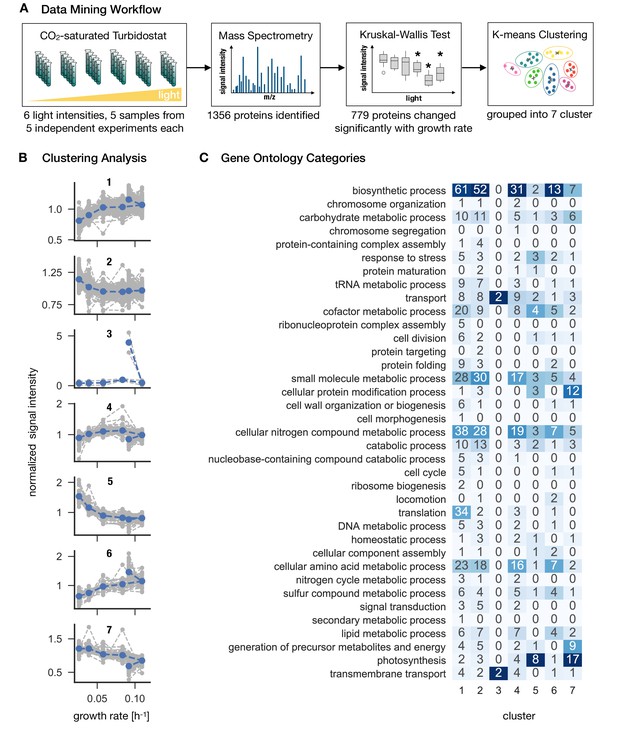
Synechocystis proteome allocation as a function of growth rate.
Panel A: The workflow. Samples were harvested and analyzed by mass spectrometry (the proteomics dataset is available in Figure 3—source data 1). A Kruskal-Wallis test was used to distinguish between growth-dependent and growth-independent proteins. 779 growth-dependent and 577 growth-independent proteins were identified. Panel B: Clustering analysis. Based on k-means clustering analysis (Figure 3—figure supplement 1), the 779 growth-dependent proteins were separated into seven clusters. Gray dashed lines represent protein abundances as medians of 5 biological replicates, normalized by the respective means. Blue dashed lines represent centroids of the respective clusters. Panel C: Proteins were annotated using the GO classes, the matrix represents the annotation mapped to GO slim categories. Proteins can be associated to several GO slim categories. The highest ranking annotation per cluster is highlighted in dark blue.
-
Figure 3—source data 1
Proteomics dataset.
- https://doi.org/10.7554/eLife.42508.013
-
Figure 3—source data 2
List of growth-dependent proteins.
- https://doi.org/10.7554/eLife.42508.014
-
Figure 3—source data 3
List of growth-independent proteins.
- https://doi.org/10.7554/eLife.42508.015
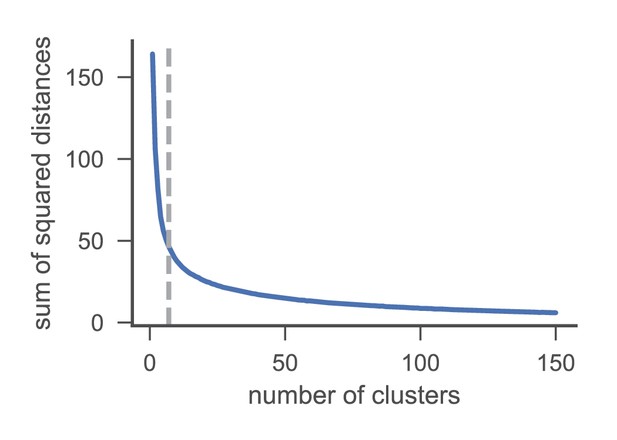
Elbow method for the identification of an appropriate number of clusters (grey dashed line at seven clusters).
https://doi.org/10.7554/eLife.42508.012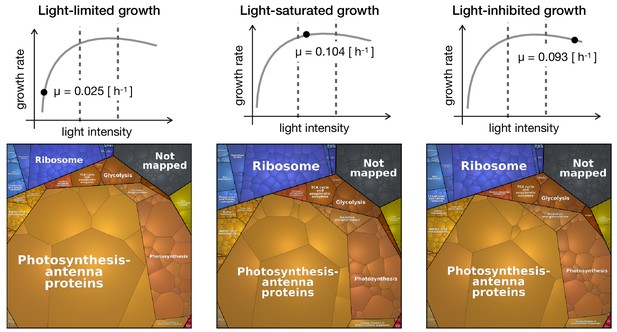
Proteomaps of proteome reallocation in Synechocystis under light-limited (27.5 μmol(photons) m-2s-1), light-saturated (440 μmol(photons) m-2s-1) and photoinhibited growth (1100 μmol(photons) m-2s-1).
https://doi.org/10.7554/eLife.42508.018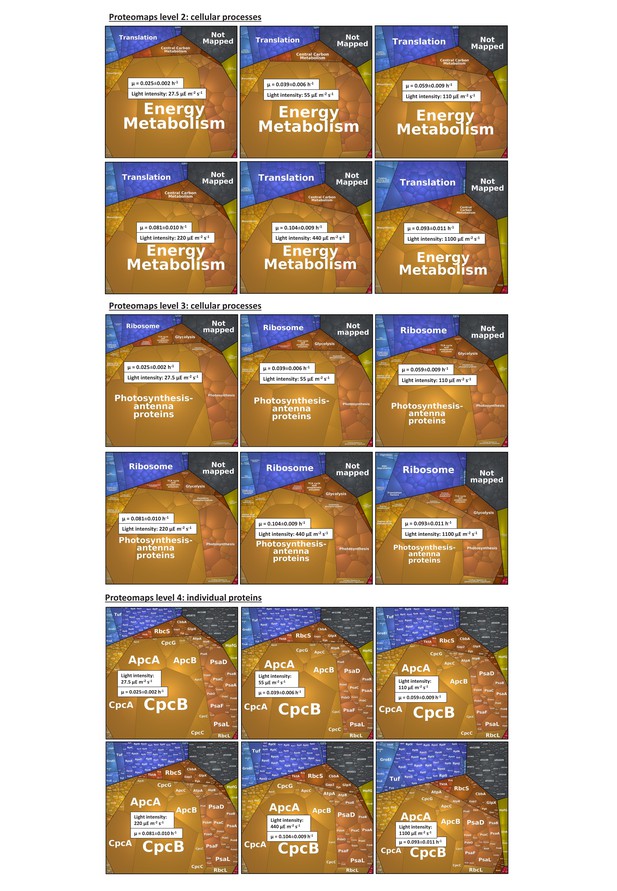
Proteomaps of levels 2, 3 and 4.
https://doi.org/10.7554/eLife.42508.019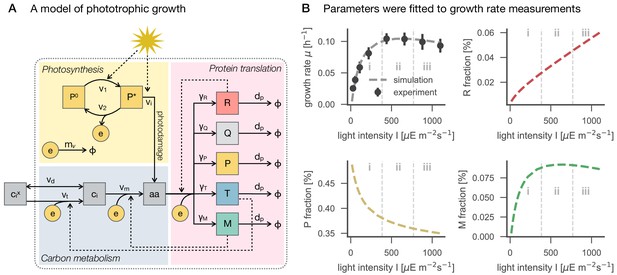
A model of phototrophic growth and reproduction of experimental growth curves.
Panel A: A coarse-grained model of phototrophic growth, adopted from Faizi et al. (2018). The model describes optimal proteome allocation under conditions of (i) light-limited, (ii) light-saturated and (iii) light-inhibited growth. Coarse-grained cellular processes include passive () and active import () of external inorganic carbon , conversion of inorganic carbon into amino acids (), light harvesting and provision of cellular energy by photosynthesis ( and ), as well as maintenance and photodamage ( and ). Amino acids are translated into coarse-grained protein fractions for transport (), metabolism (), ribosomes (), photosynthetic electron transport (), as well as a growth-independent proteome fraction . Translation is limited by the amount of available ribosomes . Panel B: The model reproduces the measured growth curve (Figure 1C–D) as a function of light intensity. Shown are the specific growth rate μ, as well as the main proteome fractions predicted by the model: ribosome () fraction, photosynthetic electron transport () fraction, and metabolism () fraction, as a function of light intensity.
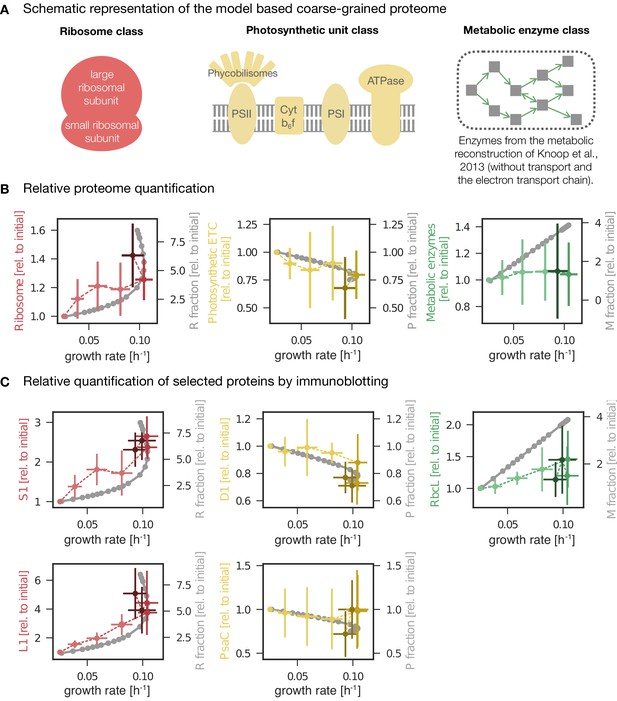
Changes in protein abundance as a function of specific growth rate compared to the predictions obtained from a computational model of proteome allocation.
Panel A: Schematic representation of ribosome, photosynthetic units and metabolic enzyme classes considered in the proteome allocation model. Panel B: Relative proteomics data ( LFQ, label-free quantification intensities, left axes, mean fold change ± SD) of protein classes in comparison with the model predictions (grey lines, right axes). Panel C: Relative protein abundances obtained by immunoblotting analysis for selected proteins (left axes, median fold change ± SD) in comparison with coarse-grained model predictions (grey lines, right axes). Experimental values represent averages from 5 independent experiments, the error bars represent standard deviations. Panels B-C: The experimental data points are displayed in three different color shading to reflect (from bright to dark) light-limited, light-saturated and light-inhibited growth. The full dataset of the immunoblotting analysis is provided in Figure 6—source data 1 and Figure 6—figure supplement 1. The list of proteins considered for ribosome, photosynthetic unit and metabolic enzyme classes is listed in Figure 6—source data 2. The influence of constant ribosomal, photosynthetic unit and metabolic enzyme classes on cellular growth rate is simulated in Figure 6—figure supplement 2.
-
Figure 6—source data 1
Results of the immunoblotting analysis.
- https://doi.org/10.7554/eLife.42508.024
-
Figure 6—source data 2
List of proteins considered for ribosome, photosynthetic unit and metabolic enzyme classes.
- https://doi.org/10.7554/eLife.42508.025
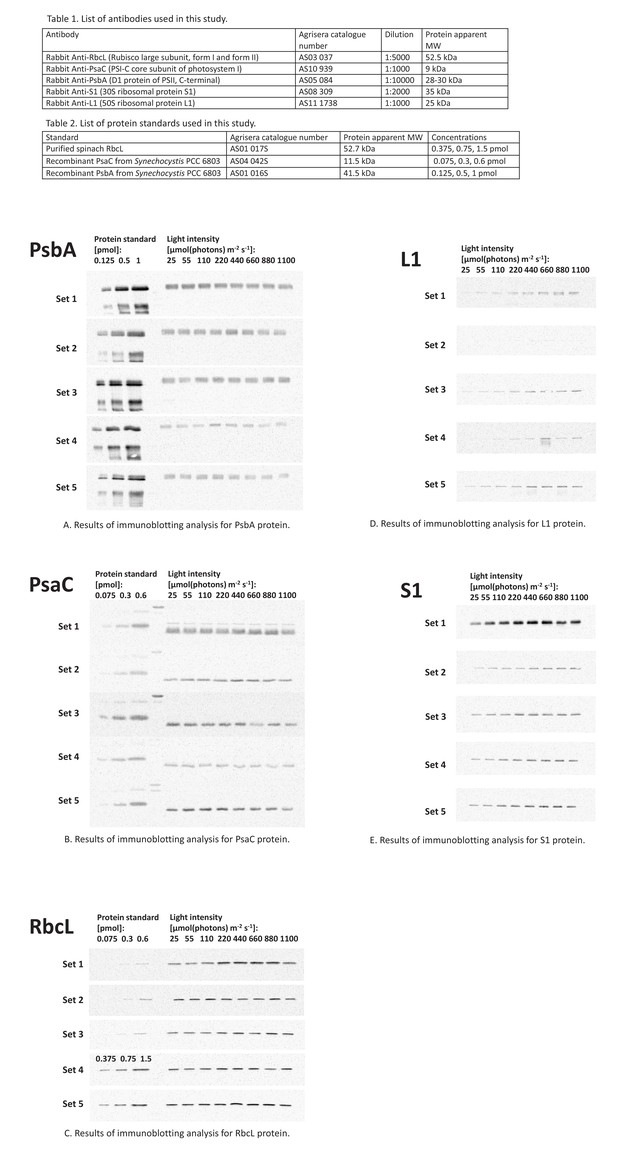
Immunoblots and a list of antibodies used for the immunoblotting analysis.
https://doi.org/10.7554/eLife.42508.022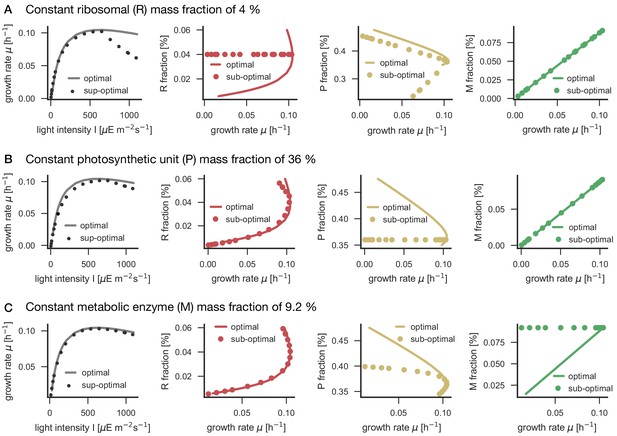
Model simulations for investigating the influence of constant enzyme fractions on the cellular growth rate.
https://doi.org/10.7554/eLife.42508.023Tables
Gene Ontology (GO) slim categories (Klopfenstein et al., 2018) with the amount of associated growth-dependent and independent proteins.
A complete list of the GO slim categories is provided in Table 1—source data 1. Here, only categories that exhibit a significant difference (Fisher's exact test, ) between growth-dependent and independent groups are listed. Shown is the number of annotations per category.
Gene ontology categories | Growth dependent | Growth independent |
---|---|---|
Translation | 40 | 13 |
Transport | 36 | 14 |
Photosynthesis | 36 | 8 |
Catabolic process | 32 | 4 |
Protein folding | 14 | 3 |
Cell division | 12 | 0 |
Cell wall organization or biogenesis | 10 | 1 |
Cell cycle | 9 | 0 |
-
Table 1—source data 1
List of all 40 GO slim categories with the respective amounts of growth-dependent and growth-independent proteins (and their cluster associations).
- https://doi.org/10.7554/eLife.42508.017
Quantification of selected protein complexes in Synechocystis cells.
Protein abundances were estimated as molecules per cell, as inferred from mass spectrometry, immunoblotting and spectrophotometric analysis. The stoichiometries of protein complexes were based on Uniprot (www.uniprot.org, (UniProt Consortium, 2018)) and RCSB (www.rcsb.org, (Berman, 2000)) databases. Protein abundances are not precise estimates but indicate ranges. The range in the second column reflects the minimal and maximal protein amounts estimated across all light intensities studied in this work. Estimation of protein abundances is detailed in Table 2—Source data 1, a list of all proteins is provided in Table 2—Source data 2. The experimental conditions of (Moal and Lagoutte, 2012) are comparable to the conditions used in this study with the exception of high light used here and distinct Synechocystis substrains (Figure 2—source data 2).
Protein complex | Molecules per cell | Method | Stoichiometry | Reference |
---|---|---|---|---|
Elongation factor | 179000–274000 | Proteomics | TufA | This study |
Phosphoglycerate kinase | 45000–73000 | Proteomics | Pgk | This study |
Ribosome small subunit | 36000–66000 | Proteomics | Rps1A,1B,B,C,D,E,F,G,H,I,J,K,L,M,N,O,P,Q,R,S,T,U | This study |
Phycobilisome (phycocyanin) | 12000–23000 | Proteomics | ((CpcA,B),C1,C2,D,G) | This study |
26000–66000 | Spectrophotometry | This study | ||
Photosystem I | 31000–63000 | Proteomics | (PsaA,B,C,D,E,F,I,J,K,L,M,X) | This study |
96000 | Spetroscopy | Keren et al., 2004 | ||
540000 | Spetroscopy | Moal and Lagoutte, 2012 | ||
Ribosome large subunit | 33000–54000 | Proteomics | RplA,B,C,D,E,F,I,J,K,L,M,N,O,P,Q,R,S,T,U,V,W,X,Y, RpmA,B,C,E,F,G,H,I,J | This study |
Transketolase | 31000–50000 | Proteomics | TktA | This study |
PII signal transducing protein | 36000–46000 | Proteomics | GlnB | This study |
Photosystem II | 23000–46000 | Proteomics | (PsbA1,A2,B,C,D,E,F,H,I,J,K,L,M,N,O,T,U,V,X,Y,Z, Ycf12) | This study |
17000–29000 | Immunoblotting | This study | ||
100000 | Spetroscopy | Moal and Lagoutte, 2012 | ||
RuBisCO | 26000–43000 | Proteomics | (RbcL, RbcS) | This study |
39000–63000 | Immunoblotting | This study | ||
Ferredoxin-NADP reductase (FNR) | 33000–42000 | Proteomics | PetH | This study |
140000 | Immunoblotting | Moal and Lagoutte, 2012 | ||
D-fructose 1,6-bisphosphatase class 2 | 29000–36000 | Proteomics | Slr20944 | This study |
Phycobilisome (allophycocyanin) | 19000–38000 | Proteomics | (ApcA,B),C,D,E,F | This study |
9000–19000 | Spectrophotometry | This study | ||
G3P dehydrogenase | 21000–32000 | Proteomics | Gap2 | This study |
Plastocyanin | 15000–29000 | Proteomics | PetE | This study |
Superoxide dismutase [Fe] | 14000–25000 | Proteomics | SodB | This study |
Orange carotenoid protein | 15000–24000 | Proteomics | Slr1963 | This study |
RNA polymerase | 8000–15000 | Proteomics | RpoA,B,C1,C2,D,E,F | This study |
Cytochrome b6/f | 8000–15000 | Proteomics | (PetA,B,C2,D,G,L,M,N) | This study |
Chaperonine GroEL | 7000–13000 | Proteomics | GroL1 | This study |
Ribosome recycling factor | 6000–7000 | Proteomics | Frr | This study |
Phosphoglycerate dehydrogenase | 3000–5000 | Proteomics | SerA | This study |
Pyruvate dehydrogenase | 3000–4000 | Proteomics | (PdhA, PdhB) | This study |
Glutamine synthetase | 2000–4000 | Proteomics | GlnA | This study |
Isocitrate dehydrogenase | 2000–3000 | Proteomics | Icd | This study |
Glycogen synthase | 2000–3000 | Proteomics | GlgA1 | This study |
DNA polymerase III | 1000–2000 | Proteomics | DnaN | This study |
Pyruvate kinase | 1000–2000 | Proteomics | Pyk2 | This study |
Acetyl-coenzyme A carboxylase | 1000 | Proteomics | AccB, AccC, AccA,ACCD | This study |
Carbonic anhydrase | 400–700 | Proteomics | IcfA | This study |
Acetyl-coenzyme A reductase | 300–600 | Proteomics | PhaB | This study |
Circadian clock proteins KaiA/KaiB/KaiC | 200–500 | Proteomics | KaiA/KaiB/KaiC | This study |
-
Table 2—source data 1
Calculations of selected protein complex copies in Synechocystis cells.
- https://doi.org/10.7554/eLife.42508.027
-
Table 2—source data 2
List of all proteins quantified by proteomics measurements in Synechocystis cells.
- https://doi.org/10.7554/eLife.42508.028
Reagent type (species) or resource | Designation | Source or reference | Identifiers | Additional information |
---|---|---|---|---|
Antibody | Rabbit Anti-PsbA | Agrisera | Cat. #: AS05 084RRID:AB_2172617 | WB (1:10000) |
Antibody | Rabbit Anti-PsaC | Agrisera | Cat. #: AS10 939 RRID:AB_10752085 | WB (1:1000) |
Antibody | Rabbit Anti-RbcL | Agrisera | Cat. #: AS03 037 RRID:AB_2175406 | WB (1:5000) |
Antibody | Rabbit Anti-S1 | Agrisera | Cat. #: AS08 309 RRID:AB_1271140 | WB (1:2000) |
Antibody | Rabbit Anti-L1 | Agrisera | Cat. #: AS11 1738 RRID:AB_10754471 | WB (1:1000) |
Peptide, recombinant protein | Recombinant PsbA from Synechocystissp. PCC 6803 | Agrisera | Cat. #: AS01 016S | 41.5 kDa |
Peptide, recombinant protein | Recombinant PsaC from Synechocystissp. PCC 6803 | Agrisera | Cat. #: AS04 042S | 11.5 kDa |
Peptide, recombinant protein | Puri1ed spinach RbcL | Agrisera | Cat. #: AS01 017S | 52.7 kDa |
Additional files
-
Supplementary file 1
Summary of the proteome allocatioin model.
- https://doi.org/10.7554/eLife.42508.029
-
Transparent reporting form
- https://doi.org/10.7554/eLife.42508.030