A chemical probe of CARM1 alters epigenetic plasticity against breast cancer cell invasion
Figures
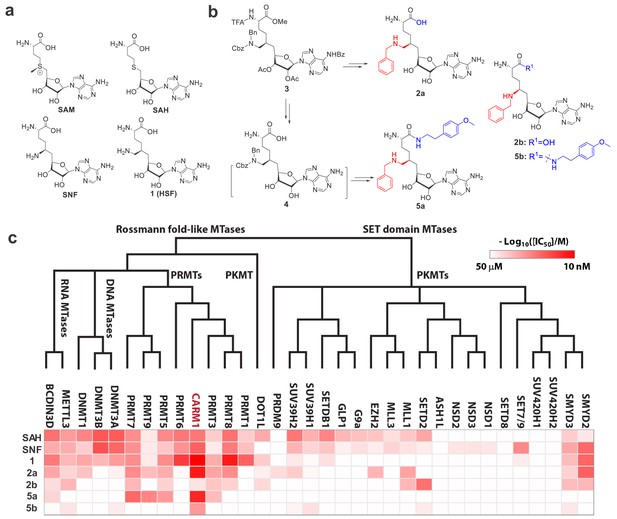
Structures, synthesis and target inhibition of SAM analogs.
(a) Structures of SAM, SAH, sinefungin (SNF) and 6′-homosinefungin (HSF, 1). (b) Structures and synthetic outline of HSF derivatives 2a and 5a, and their structurally related control compounds 2b and 5b. (c) IC50 heat-map of SAM analogs against 34 methyltransferases. HSF derivatives 2a and 5a were identified as potent and selective inhibitors of CARM1; 2b and 5b as their respective control compounds.
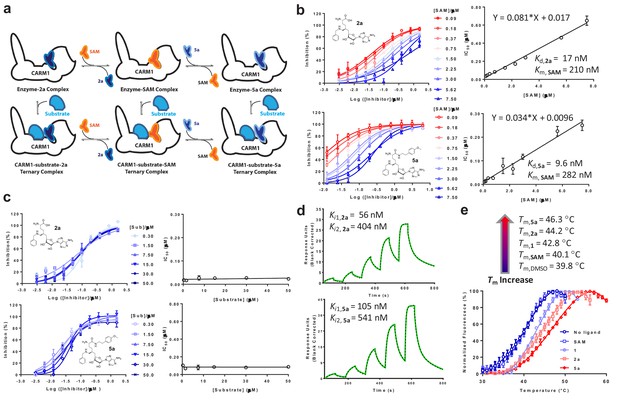
In vitro characterization of CARM1 inhibitors 2a and 5a.
(a) Schematic description of CARM1 in complex with SAM, 2a and 5a in the absence or presence of a substrate peptide. (b, c) IC50 of 2a and 5a in the presence of varied concentrations of SAM and H3 peptide substrate. IC50 data were obtained and presented as the mean of replicates ± standard errors. The IC50 values of 2a and 5a show a linear increase relative to the SAM concentration but remain near constant as the the substrate concentration increases. Given the SAM competitive character, the Kd values of 2a and 5a as well as Kd,SAM can be obtained according to IC50 = [SAM]×Kd/Kd,SAM+Kd. (d) SPR assay for the binding of CARM1 by 2a and 5a. Processed sensorgrams upon ligand binding (black dots) were fitted with a biphasic binding model (green line) with Ki1,2a = 56 nM (0.06 ± 0.02 µM) and Ki2,2a = 404 nM (0.4 ± 0.1 µM); Ki1,5a = 105 nM (0.10 ± 0.01 µM) and Ki2,5a = 541 nM (0.54 ± 0.07). (e) Thermal shift assay of CARM1 in the absence or presence of SAM, 1, 2a, and 5a. Tm values of 39.8 ± 0.2°C, 40.1 ± 0.5°C, 42.8 ± 0.3°C, 44.2 ± 0.6°C and 46.3 ± 0.3°C (means of triplicates ± standard derivatives) were obtained for apo-CARM1 and CARM1 complexes with 5 μM SAM, 1, 2a, and 5a, respectively.
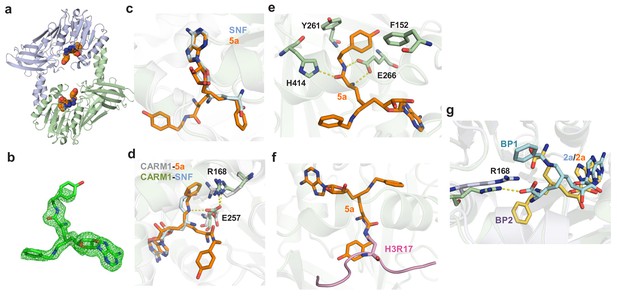
Crystal structure or molecular modeling of CARM1 in complex with 5a and 2a.
(a) Overview of the Rossmann fold in the X-ray structure of CARM1 with 5a. (b) Total omission electron density map of 5a in the CARM1–5a complex. The total omission electron density map was calculated using SFCHECK, as described in the Materials and methods. The electron density contoured at 1.0 σ is shown for the ligands. (c) Comparison of the binding modes between 5a (noncanonical) and SNF (canonical). The structure of SNF)was extracted from a CARM1–SNF–H3R17 complex (PDB 5D × 0). (d) Key interactions between CARM1 and ligands in canonical and noncanonical binding modes. The differentiated interactions are highlighted in gray (CARM1) and blue (SNF) for the canonical mode; and in green (CARM1) and orange (5a) for the noncanonical mode. (e) Additional interactions in which the α-amino amide moiety of 5a forms hydrogen bonds with Glu266 and His414 and hydrophobic interactions with Phe152 and Tyr261. (f) Steric clash between the α-amino amide moiety of 5a and an Arg substrate. The structure of the Arg substrate was extracted from a CARM1–SNF–H3R17 complex (PDB 5D × 0). (g) Two modeled binding poses (BP1 and BP2) of 2a upon binding CARM1 with the Cβ-Cγ-Cδ-Nε dihedral angle χ3 = 180°; the C4'-C5'-C6'-C7' dihedral angle of −50o for BP1 versus χ3 = −65° C4'-C5'-C6'-C7' dihedral −170o for BP2.
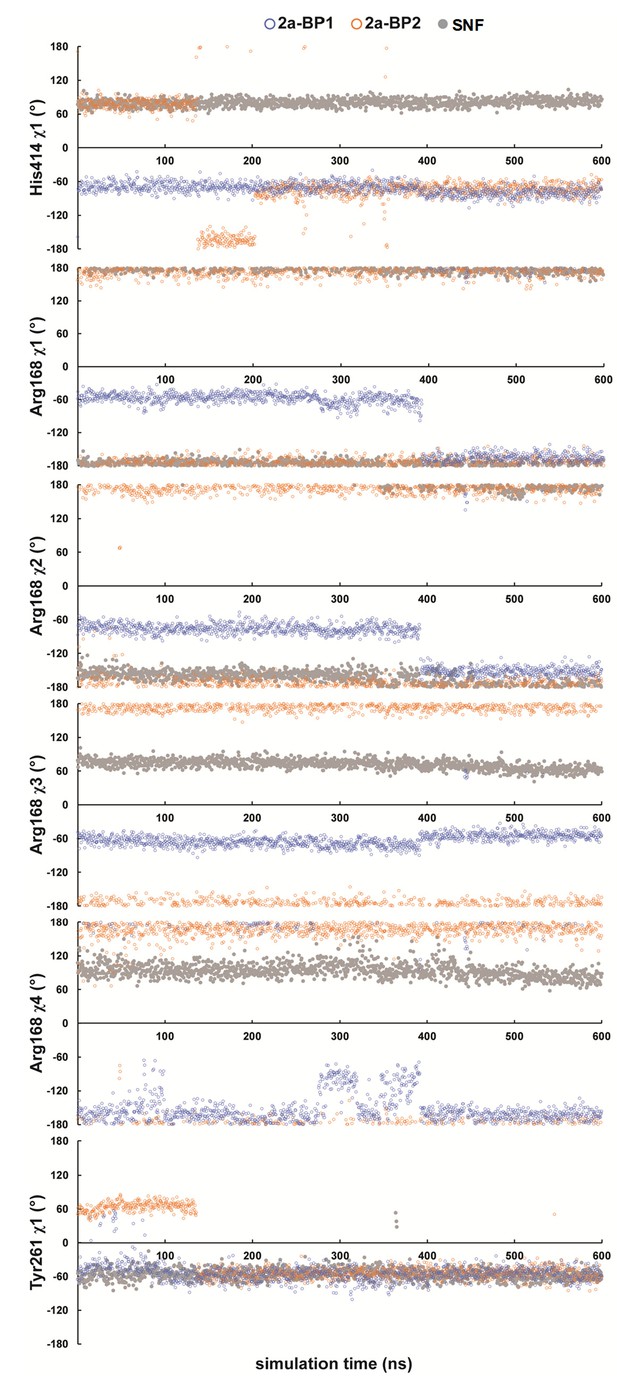
Conformational dynamics of CARM1–2a and CARM1–SNF complexes.
Multiple configurations of Arg168, Tyr261 and His414 and their kinetics were revealed through 600 ns MD simulation for CARM1–2a and CARM1–SNF complexes.
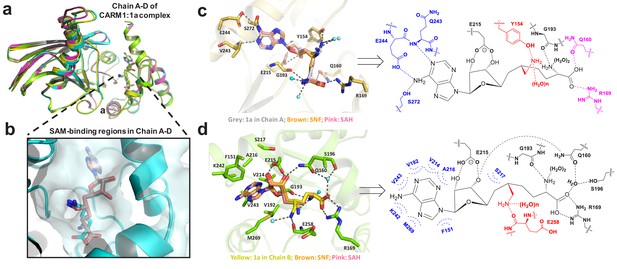
Structure of CARM1 in complex with 1 (HSF) in multiple configurations (PDB: 4IKP).
(a) Overall structures of the CARM1–1 complex featuring V-shape subunits in a tetramer (Chain A–D). (b) Multiple configurations of 1 upon occupying the SAM-cofactor-binding site of CARM1. (c) Representative interaction network of 1 (Configuration III, IV in Chain A) upon binding human CARM1, and its comparison with SNF and SAH. Here, we highlight the conserved hydrogen bonds with adenine ring (blue), the 2′,3′-ribosyl hydroxyl/α-amino groups (black), and the distinct interaction network in Chain A for carboxylic moieties/6′-methyleneamine (pink and red). (d) Representative interaction network of 1 (Configuration I in Chain B) upon binding human CARM1, and its comparison with SNF and SAH. Here, we highlight the conserved hydrophobic interactions with adenine ring (blue), the conserved hydrogen bond interactions with 2′,3′-ribosyl hydroxyl (black), the α-amino, carboxylic moieties and the distinct interaction network of 6′-methyleneamine (red). The images of SNF and SAH were generated on the basis of PDB files 2Y1W and 2Y1X.
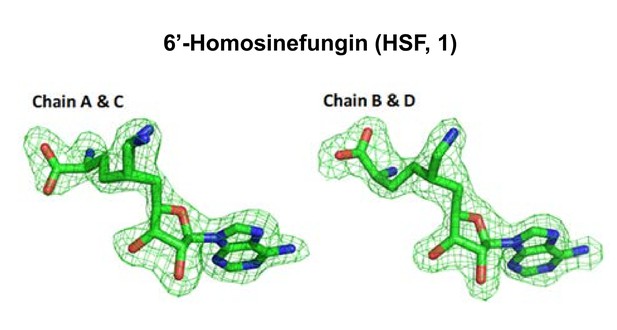
Total omission electron density map of 6′-homosinefungin (HSF, 1) in the CARM1–1 complex.
The total omission electron density map was calculated using SFCHECK, as described in the Materials and methods. The electron density contoured at 1.0 σ is shown for the ligands.
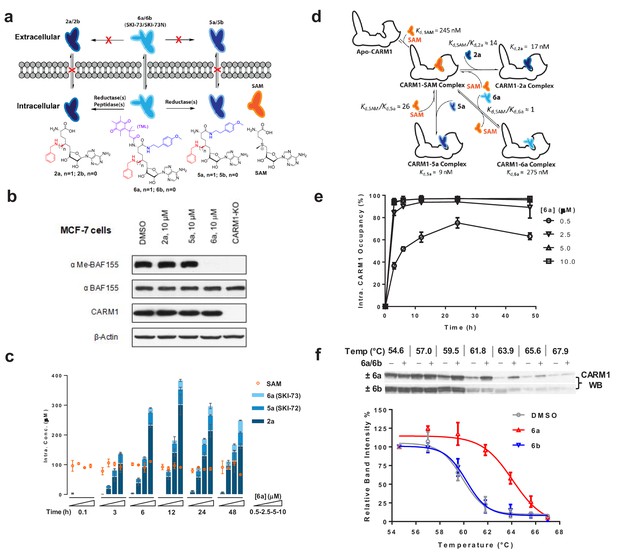
Characterization of cellular activity of 6a as a chemical probe.
(a) Schematic description of the extracellular and intracellular fates of 2a, 5a and 6a. Extracellularly, 2a, 5a and 6a are stable; only 6a can readily penetrate cell membrane. Intracellularly, 6a can be processed into 5a and 2a. Given the poor membrane permeability of 2a and 5a, they are accumulated within cells at high concentrations. (b) CARM1 inhibition of 2a, 5a and 6a in MCF-7 cells with BAF155 methylation as a mark. MCF-7 cells were treated with 10 μM of 2a, 5a and 6a for 48 hr. The ratios between me-BAF155 and BAF155 were quantified as a cellular reporter of CARM1 inhibition. DMSO-treated MCF-7 cells and MCF-7 CARM1-KO cells were used as negative and positive controls, respectively. (c) MS-based quantification of intracellular concentrations of 2a, 5a, 6a and SAM. These compounds were accumulated within MDA-MB-231 cells in a dose- and time-dependent manner. In comparison, the intracellular concentration of SAM remains a constant at 89 ± 16 µM. (d) Schematic description of intracellular engagement of CARM1 by 6a, 5a and 2a in the presence of the SAM cofactor (Equations 5–7). (e) Modeled ligand occupancy of CARM1 with 2a, 5a and 6a as ligands in competition with the SAM cofactor. Percentage of competitive CARM1 occupancy was calculated on the basis of the concentrations of ligands (SAM, 2a, 5a and 6a, Figure 5c) and their Kd values (Figure 2c). (f) Cellular thermal shift assay (CETSA) of CARM1. Representative western blots of CARM1 in MDA-MB-231 cells upon the treatment of 15 μM 6a or its negative control 6b for 48 hr with DMSO treatment as reference. The relative intensity of CARM1 was quantified. The Tm values were determined at the 50% loss of the relative intensity signals with Tm,6a = 63.9 ± 0.3 °C, Tm,6b = 60.2 ± 0.6 °C and Tm,DMSO = 59.6 ± 0.2 °C.
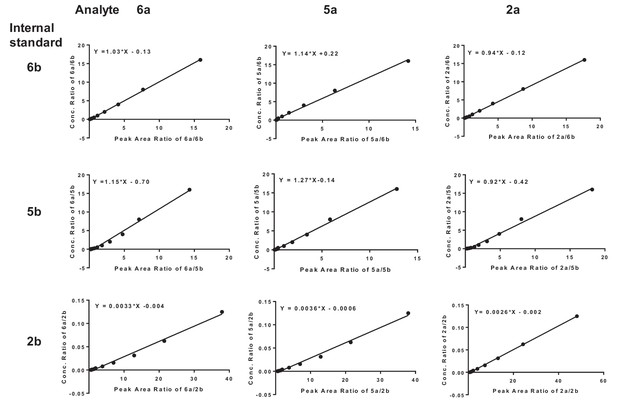
LC-MS/MS working curves for quantification of the analytes 6a (SKI-73), 5a and 2a, with 6b (SKI-73N), 5b, and 2b as internal standards.
The concentration ratios show a linear correlation with the mass peak area ratios between the analyte and the internal standards.
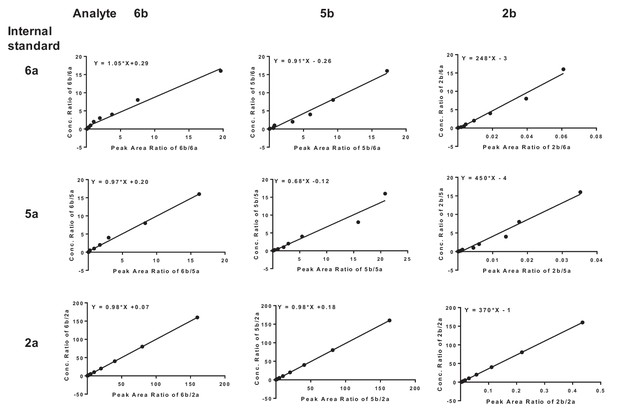
LC-MS/MS working curves for quantification of the analytes 6b (SKI-73N), 5b and 2b, with 6a (SKI-73), 5a, and 2a as internal standards.
The concentration ratios show a linear correlation with the mass peak area ratios between the analyte and the internal standards.
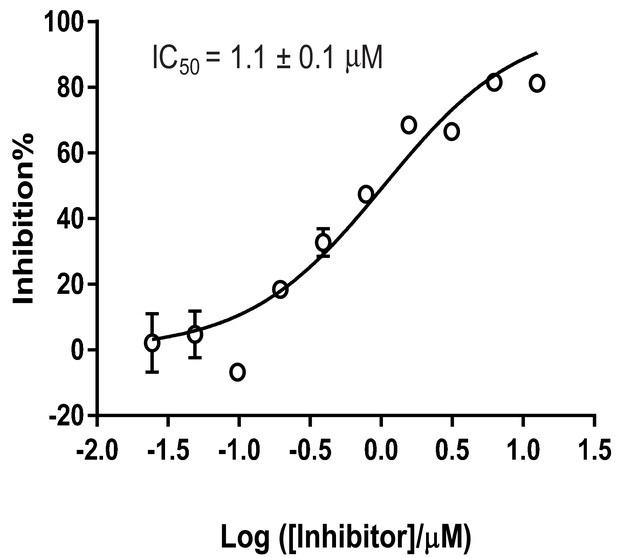
IC50 of 6a (SKI-73) against CARM1.
The IC50 value was obtained by fitting inhibition% against the concentration of the inhibitor to a sigmoid curve with GraphPad Prism. Each data point represents the mean of two replicates and their s.d.
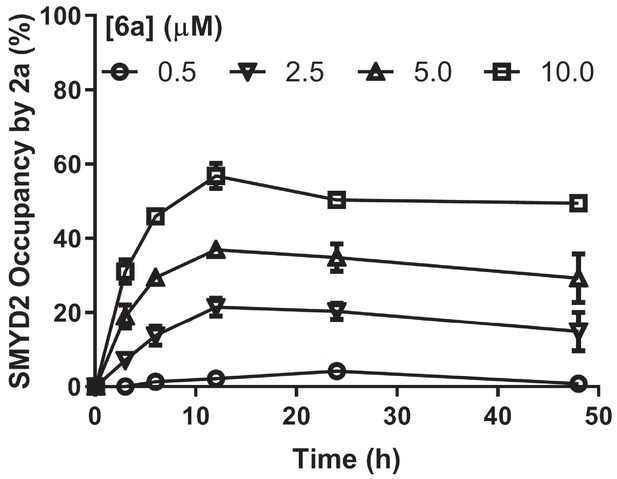
Modeled ligand occupancy of SMYD2 by 2a.
Percentage of competitive SMYD2 occupancy was calculated on the basis of the concentrations of SAM and 2a (Figure 5c) and their Kd values (Kd,2a=150 nM and Kd,SAM=60 nM), as described in Materials and methods.
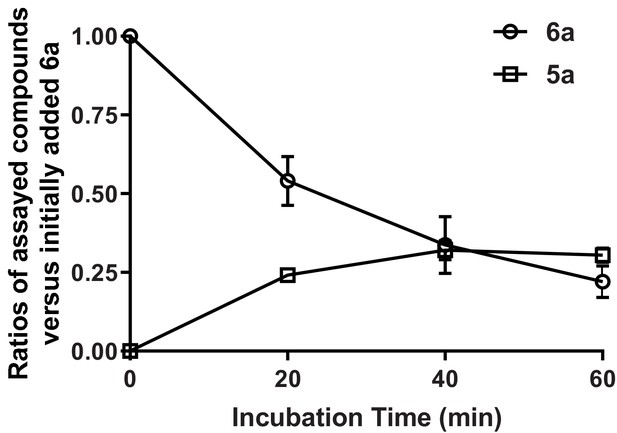
Microsome stability of 6a (SKI-73).
SKI-73 (6a) was incubated with microsomes at the indicated time intervals. The assay mixture was analyzed by LC-MS/MS for quantification of the residual 6a (SKI-73) and the production of 5a and 2a. Molar ratios of the assayed compounds versus initially added 6a (SKI-73) were plotted against the incubation time. No production of 2a was observed under the current assay conditions.
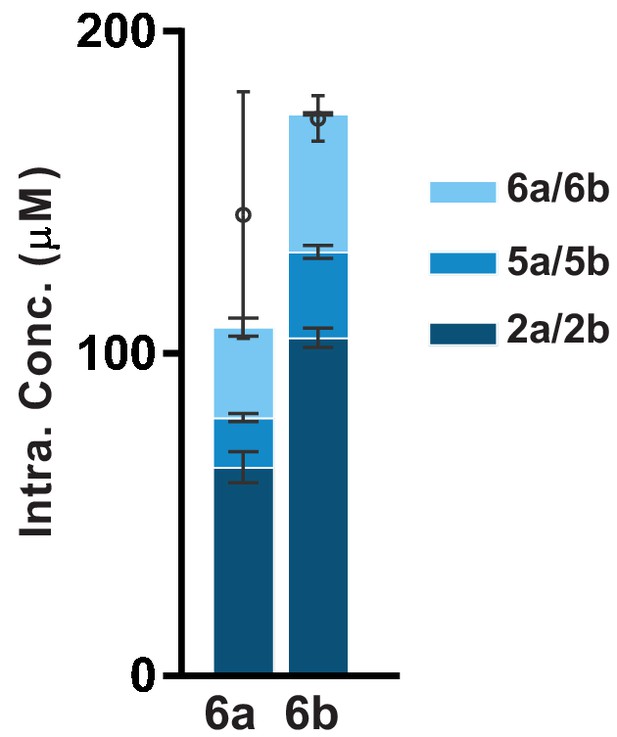
Cellular uptake and intracellular fate of 6a (SKI-73) and 6b (SKI-73N).
MDA-MB-231 cells were incubated with 10 μM 6a (SKI-73) and 6b (SKI-73N) for 24 hr. LC-MS/MS was used to quantify the intracellular concentrations of (6b (SKI-73N), 5b, 2b) and (6a (SKI-73), 5a, 2a), respectively (n = 3, mean values ± s.d.). ([6b [SKI-73N]), 5b, 2b) were accumulated inside MDA-MB-231 cells with the efficiency comparable to that of [6a (SKI-73), 5a, 2a]).
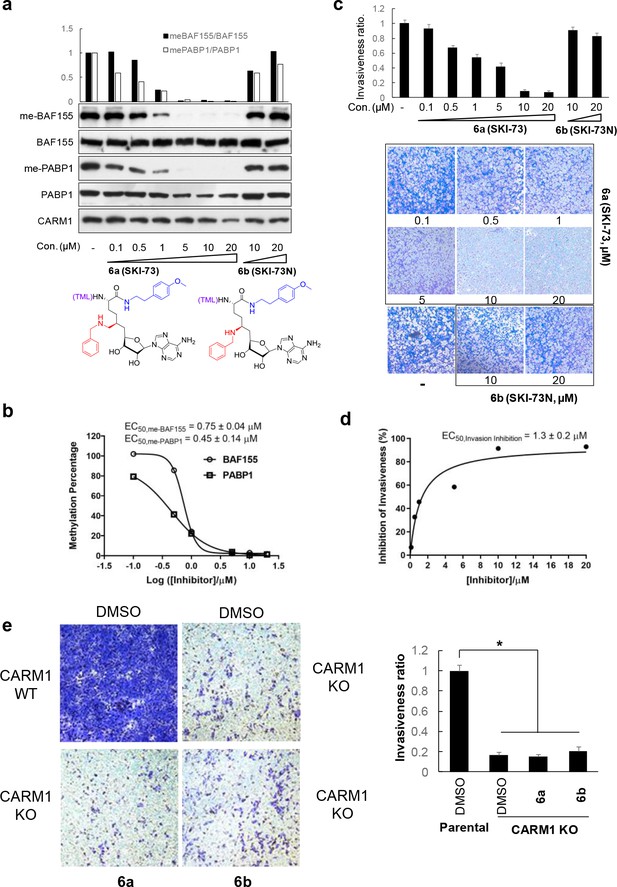
Biological outcomes of CARM1 inhibition by 6a in MDA-MB-231 cells.
(a) Dose-dependent depletion of BAF155 methylation and PABP1 methylation by 6a. BAF155 methylation and PABP1 methylation, two marks of the CARM1-specific methyltransferase activity, were examined upon the treatment of 6a and its structural analog 6b (negative control compound) for 48 hr. Western Blot analysis was then conducted to quantify the relative intensities of the methylated versus total proteins (BAF155 and PABP1, two replicates with a representative one shown). (b) EC50 of the methylation depletion of BAF155 and PABP1. The relative intensity of the methylated versus total BAF155 or PABP1 was plotted against log[6a], with the resultant EC50obtained upon fitting a standard sigmoid curve using GraphPad Prism. (c) Inhibition of cell invasion by 6a. Representative images of the trans-well migration of MDA-MB-231 cells a shown upon treatment with various concentrations of 6a (SKI-73) or its control compound 6b (SKI-73N) for 16 hr. Invasive cells were fixed and stained with crystal violet. The invasiveness ratios were determined using the relative cell invasion of the treatment of 6a or 6b versus DMSO treatment. (d) EC50 of invasion inhibition by 6a. The invasiveness ratios were plotted as a function of the concentration of 6a. EC50 of 1.3 ± 0.2 µM was obtained upon fitting a standard sigmoid curve using GraphPad Prism. (e) Effect of 6a on cell invasion in combination with CARM1-KO. Representative images of the trans-well migration of parent and CARM1-KO MDA-MB-231 cells are shown upon treatment with DMSO, 6a or 6b for 16 hr. The results were analyzed in a similar manner to that described for Figure 5c,d. Statistical analysis was carried out to calculate mean ± standard derivation (N = 5) and to perform two-tailed paired t-tests *, p=0.05.
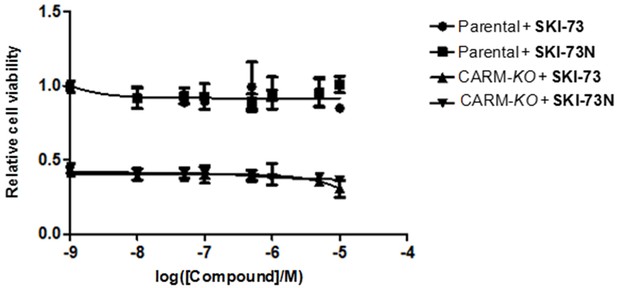
Viability of parental and CARM1-KO MDA-MB-231 cells upon treatment with SKI-73 (6a) and its control compound SKI-73N (6b).
The cells were treated with 0.0001 ~ 10 μM of SKI-73 (6a) or SKI-73N (6b) for 72 hr. MTT assay was then performed to examine their relative viability with DMSO-treated parental cells as the reference.
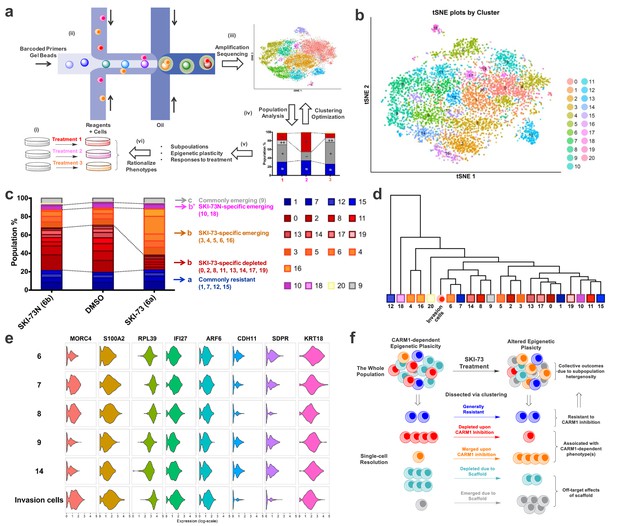
scRNA-seq analysis of MDA-MB-231 cells upon CARM1 perturbation.
(a) Schematic description of scRNA-seq analysis algorithms. (b) tSNE plots of the 21 clustered subpopulations of the G0/G1-phase cells treated with SKI-73N (6b), DMSO and SKI-73 (6a). (c) Population analysis of the 21 clustered subpopulations of the G0/G1-phase cells upon treatment with SKI-73N (6b) or SKI-73 (6a). (d) Phylogenic tree of the 21 clustered subpopulations of the G0/G1-phase cells and invasive cells. (e) Violin plots of the representative transcripts of G0/G1-phase invasion cells that distinguish Subpopulation-8 from the closely related Subpopulations 6, 7, 9, and 14 of G0/G1-phase cells. (f) Working modeling of CARM1-dependent epigenetic plasticity perturbed by SKI-73 (6a).
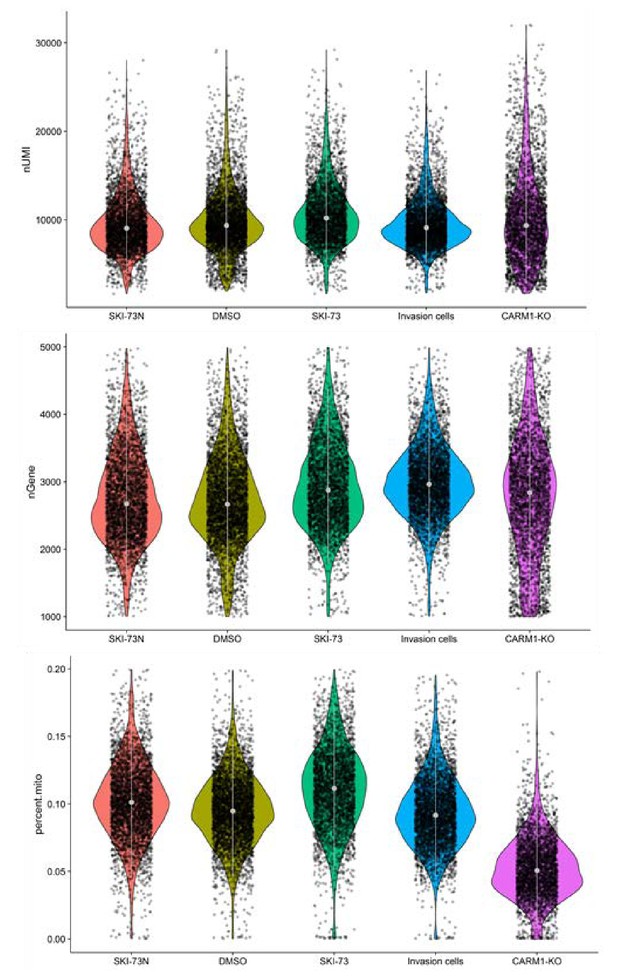
Quality control of the cells that were subjected to scRNA-seq analysis.
MDA-MB-231 cells treated with SKI-73N (6b), DMSO or SKI-73 (6a), invasive cells (MDA-MB-231 cells that freshly invaded through Matrigel), and CARM1-KO MDA-MB-231 cells were collected for 10 × Genomics scRNA-seq. Thresholds of 1000~5000 genes and <20% mitochondrial RNA transcripts were used to select the cells for further analysis. Their unique molecular identifier (UMI) counts (top), the number of genes per cell (middle) and the fraction of mitochondria genes (bottom) are shown.
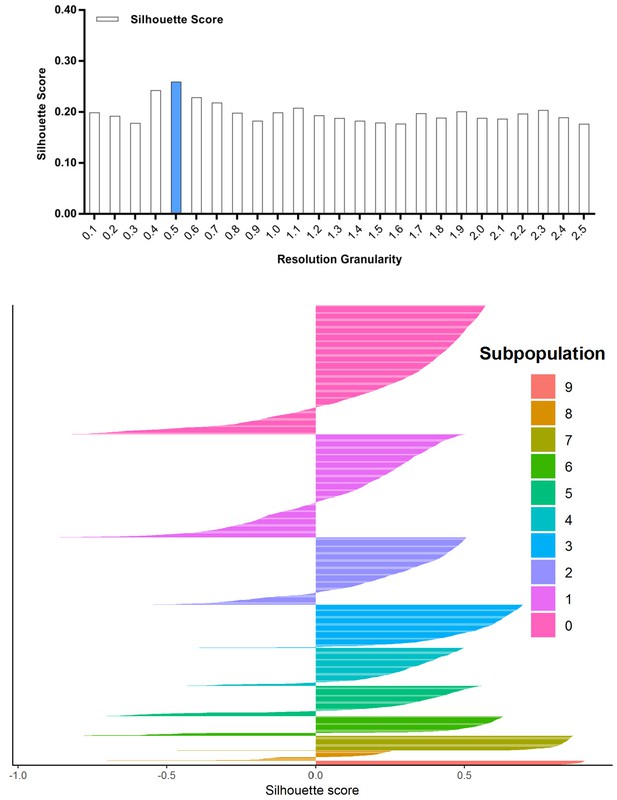
Silhouette analysis of the combined cell population treated with DMSO, SKI-73 (6a) or SKI-73N (6b) guided by the resolution granularity.
The resolution granularity of 0.5 for the highest Silhouette score was used for clustering, which identified ten subpopulations within the total cell population.
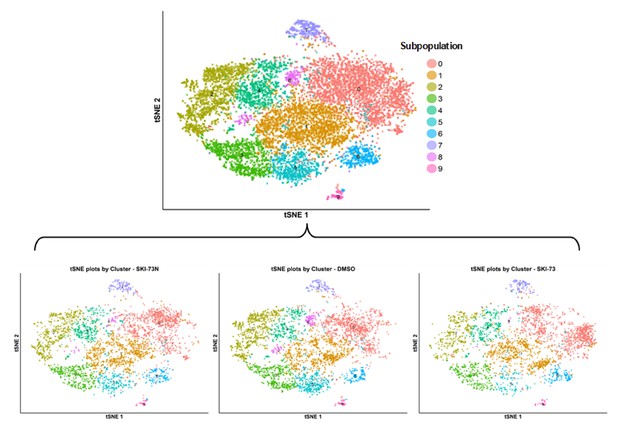
tSNE plot of the subpopulations of the combined cells treated with DMSO, SKI-73 (6a) or SKI-73N (6b).
The combined cell population treated with DMSO, SKI-73 (6a) or SKI-73N (6b) (the top panel) was clustered into ten subpopulations with the resolution granularity of 0.5. The tSNE plot of the ten clustered subpopulations was split according to their three treatment origins: SKI-73N (6b), DMSO or SKI-73 (6a).
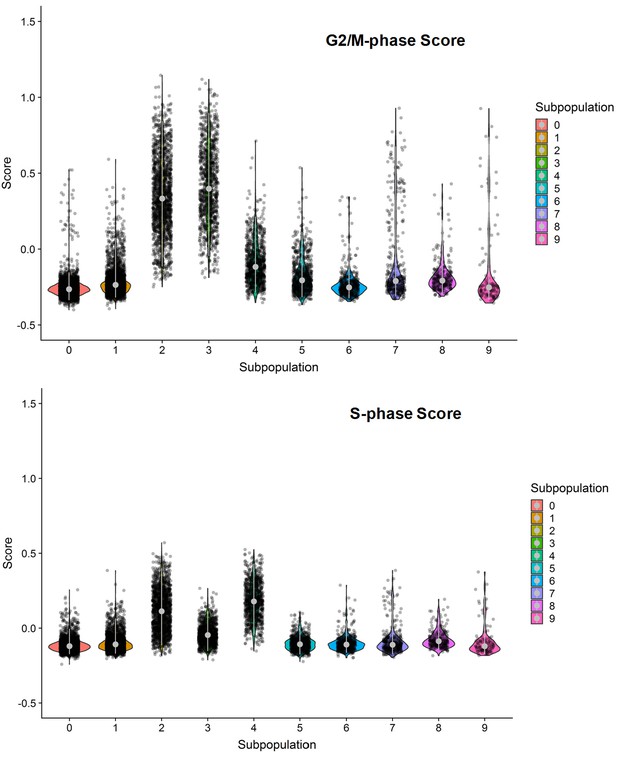
G2/M- and S-phase scores of the ten clusters within the combined cell population treated with DMSO, SKI-73 (6a) or SKI-73N (6b).
Subpopulations 2, 3 and 4 were dominated by G2/M- or S-phase scores.
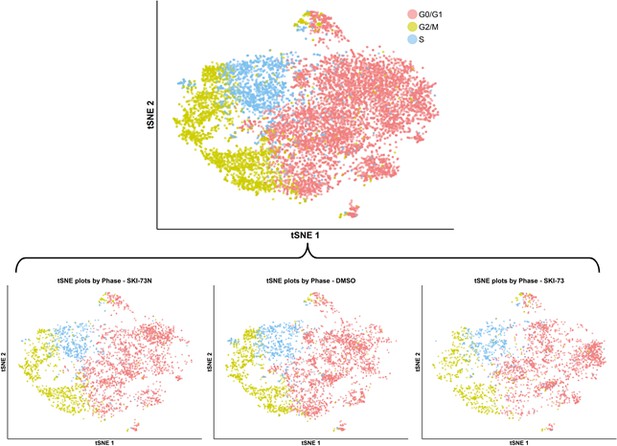
tSNE plot with cell-cycle awareness for the combined cell population treated with DMSO, SKI-73 (6a) or SKI-73N (6b).
The total population of cells treated with DMSO, SKI-73 (6a) or SKI-73N (6b) (the top panel) was classified according to the cell cycle scores of individual cells as described in the Materials and methods. The tSNE plot of the cell-cycle-aware population was split according to the three treatment origins of the cells: SKI-73N (6b), DMSO or SKI-73 (6a).
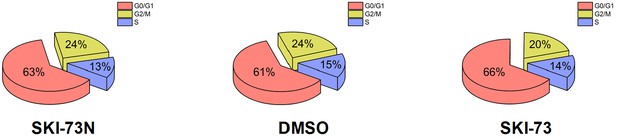
Assignment of cell-cycle stages to the cells treated with SKI-73N (6b), DMSO, or SKI-73 (6a).
Individual cells were classified according to their highest cell-cycle scores for G2/M or S phases, as described in the Materials and methods. Those cells that could not be scored for G2/M and S phases were assigned as G0/G1-phase cells. The two-day treatment with SKI-73 (6a) or SKI-73N (6b) had a negligible effect on cell cycle.
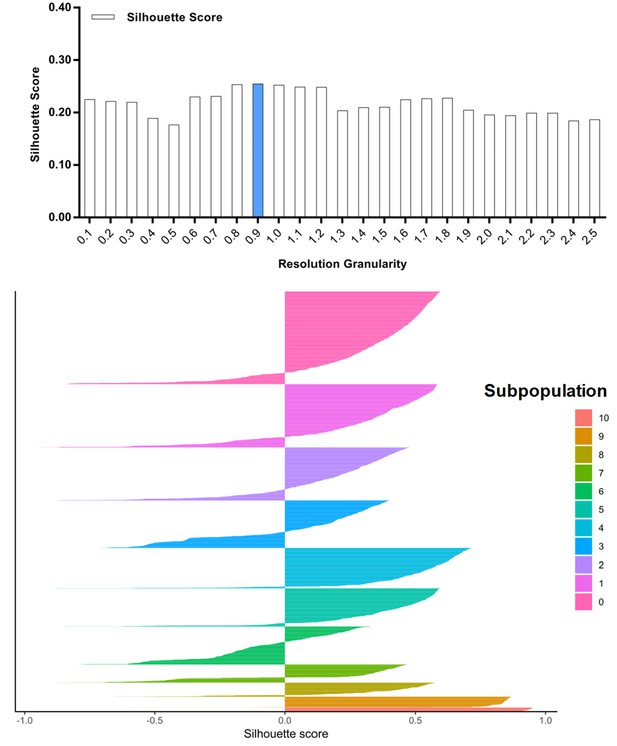
Silhouette analysis of the DMSO-treated cells guided by the resolution granularity.
A resolution granularity of 0.9 for the highest Silhouette score was used for clustering and led to the identification of six subpopulations within the DMSO-treated cells.
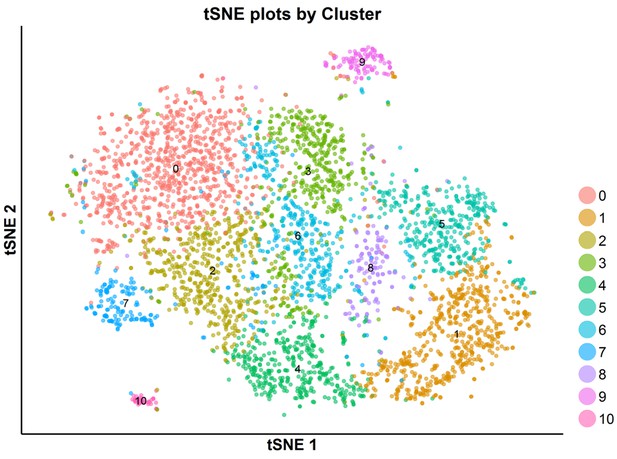
tSNE plot of the subpopulations of the DMSO-treated cells.
The DMSO-treated cells were clustered into 11 subpopulations with the resolution granularity of 0.9.
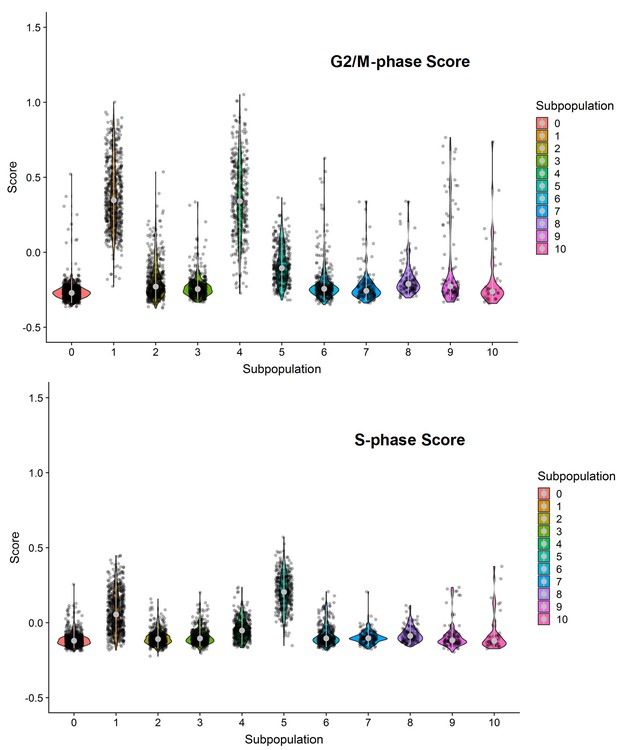
G2/M- and S-phase scores of the 11 clustered subpopulations of DMSO-treated cells.
Subpopulations 1, 4 and 5 were dominated by G2/M- or S-phase scores.
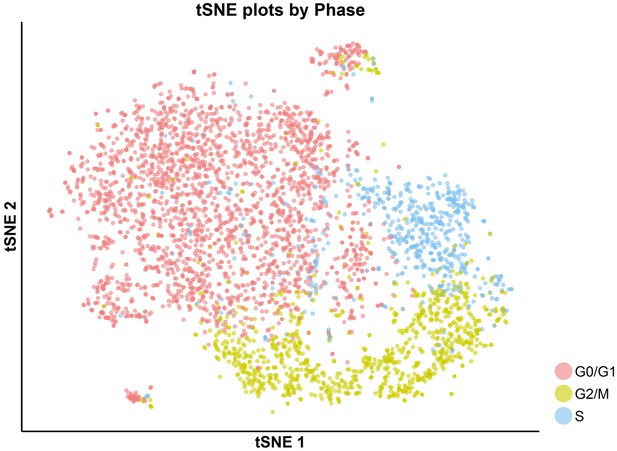
tSNE plot with cell-cycle awareness for the DMSO-treated cells.
Individual cells were classified according to their cell-cycle scores, as described in the Materials and methods.
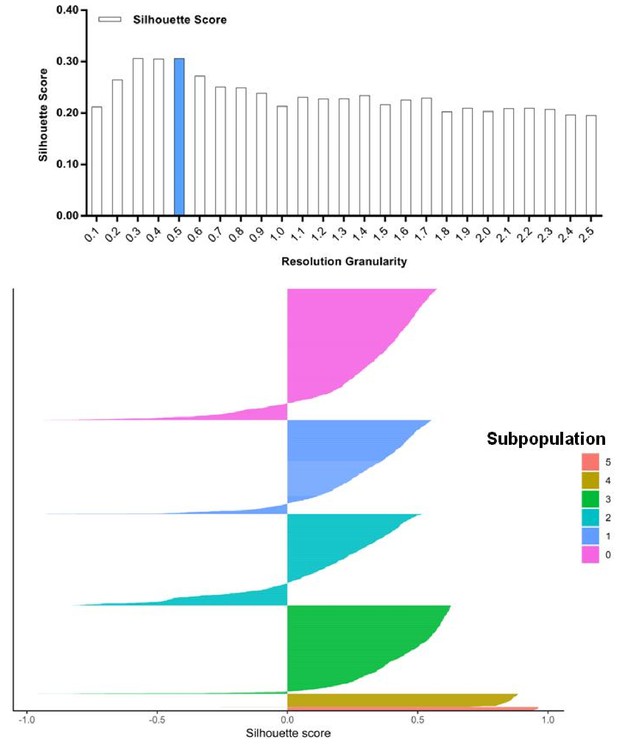
Silhouette analysis of the SKI-73N(6b)-treated cells guided by the resolution granularity.
A resolution granularity of 0.5 for the highest Silhouette score was used for clustering and led to the identification of six subpopulations within the SKI-73N(6b)-treated cells.
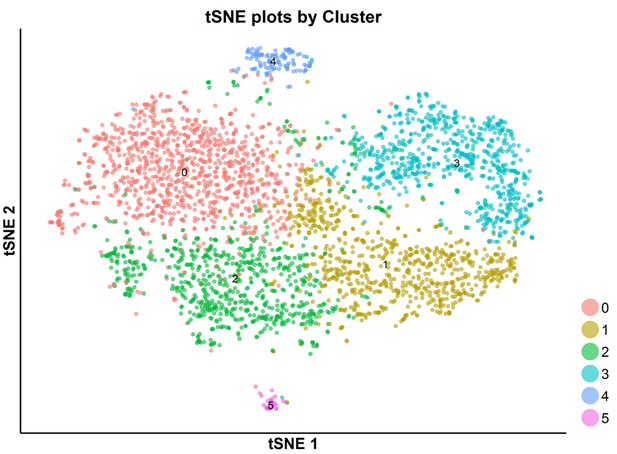
tSNE plot of the subpopulations of SKI-73N(6b)-treated cells.
The SKI-73N(6b)-treated cells were clustered into six subpopulations using a resolution granularity of 0.5.
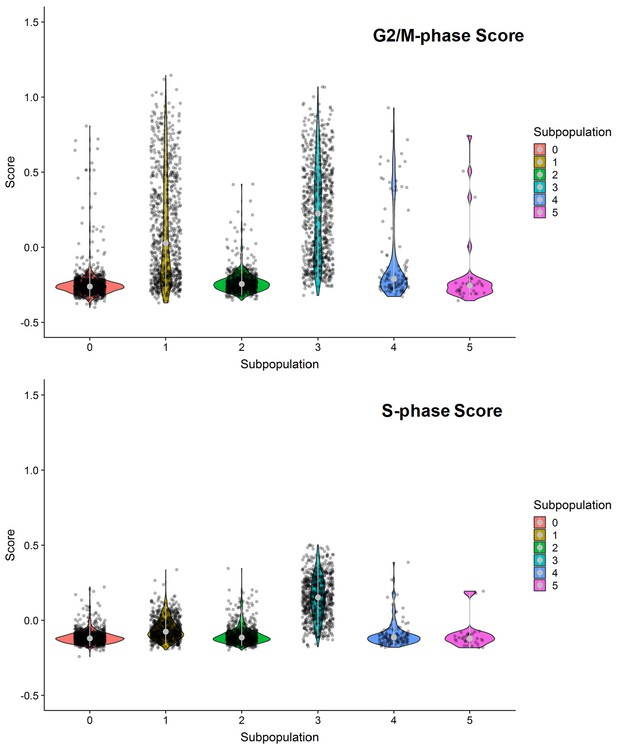
G2/M- and S-phase scores of the six clustered subpopulations of SKI-73N(6b)-treated cells.
Subpopulations 1 and 3 were dominated by G2/M- or S-phase scores.
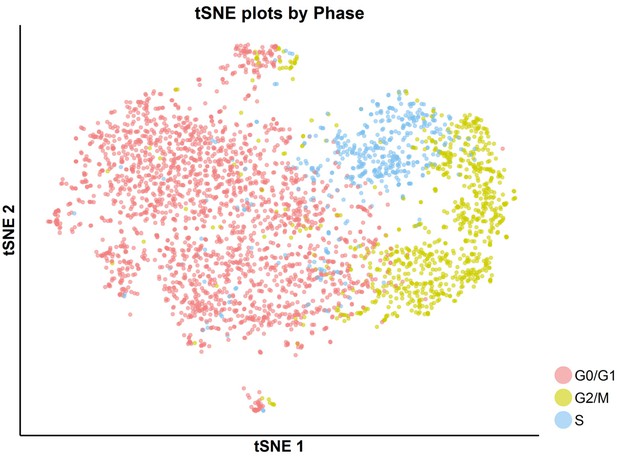
tSNE plot with cell-cycle awareness for the SKI-73N(6b)-treated cells.
Individual cells were classified according to their cell-cycle scores as described in the Materials and methods.
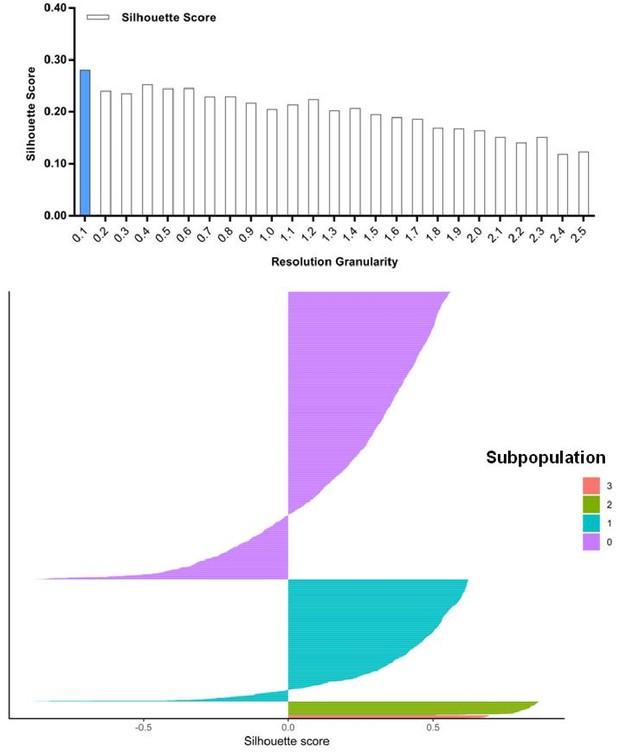
Silhouette analysis of the SKI-73(6a)-treated cells guided by resolution granularity.
A resolution granularity of 0.1 for the highest Silhouette score was used for clustering and led to the identification of four subpopulations within the SKI-73(6a)-treated cells.
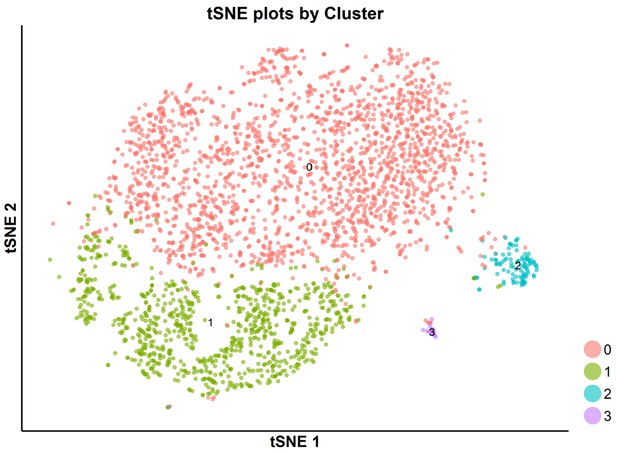
tSNE plot of the subpopulations of SKI-73(6a)-treated cells.
The SKI-73(6a)-treated cells were clustered into four subpopulations using a resolution granularity of 0.1.
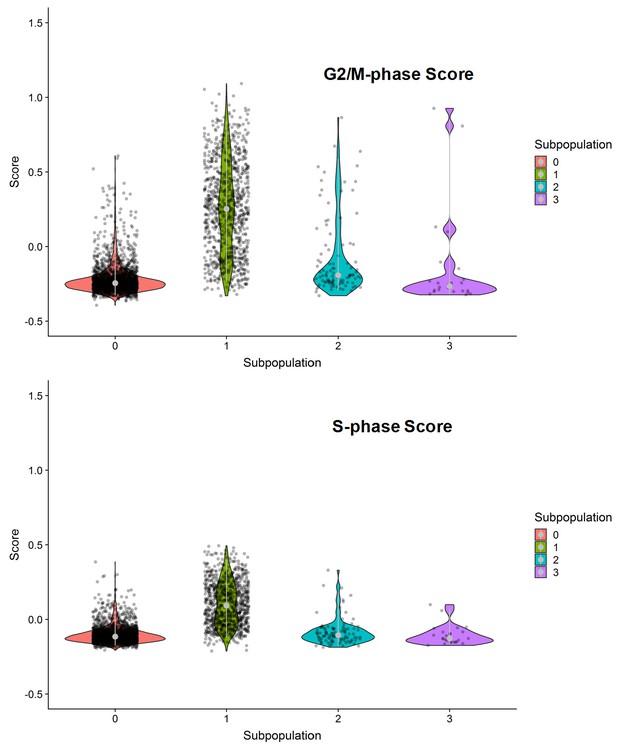
G2/M- and S-phase scores of the four clustered subpopulations within the SKI-73(6a)-treated cells.
Subpopulation 1 was dominated by G2/M- or S-phase scores.
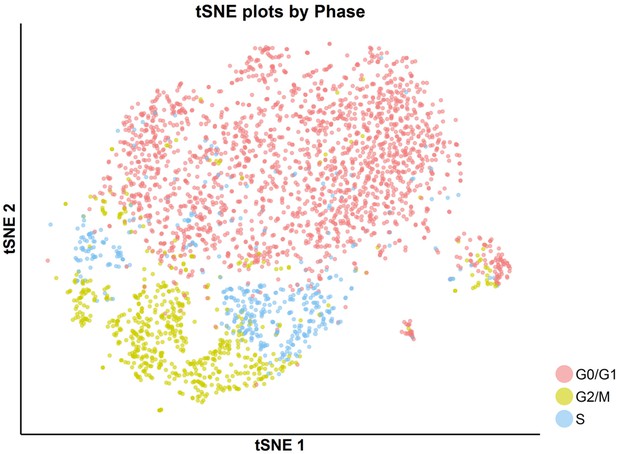
tSNE plot with cell-cycle awareness for the SKI-73(6a)-treated cells.
Individual cells were classified according to their cell-cycle scores as described in the Materials and methods.
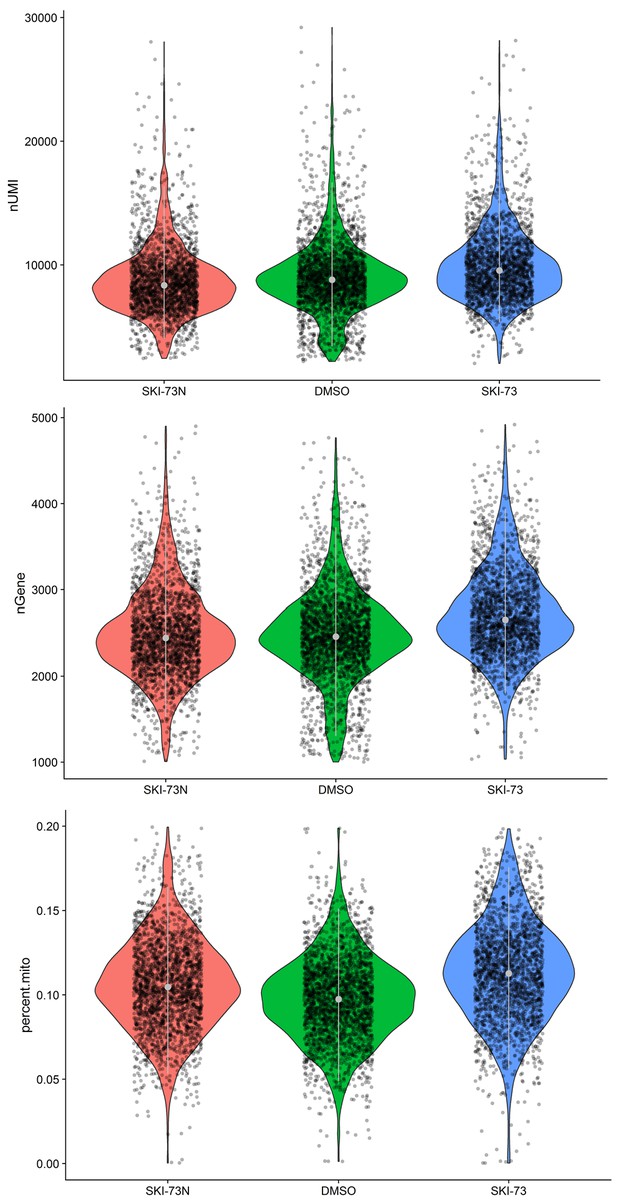
Quality control for the G0/G1-phase cells subjected to scRNA-seq analysis.
A subset of MDA-MB-231 cells after the treatment with SKI-73N (6b), DMSO or SKI-73 (6a) was assigned as G0/G1-phase cells (as described in the Materials and methods). Thresholds of 1000~5000 genes and <20% mitochondrial RNA transcripts were used to select the G0/G1-phase cells for scRNA-seq analysis, with their UMIs (top), the number of genes per cell (middle) and the fraction of mitochondrial genes (bottom) shown.
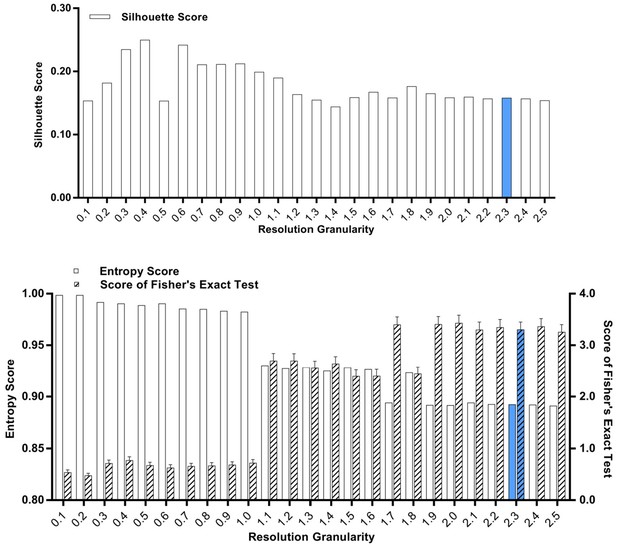
Silhouette analysis, entropy analysis and Fisher’s exact test for the classified G0/G1-phase cells after the treatment with SKI-73N (6b), DMSO or SKI-73 (6a).
A resolution granularity of 2.3 for a low entropy score and a high Fisher’s exact test score were used for clustering.
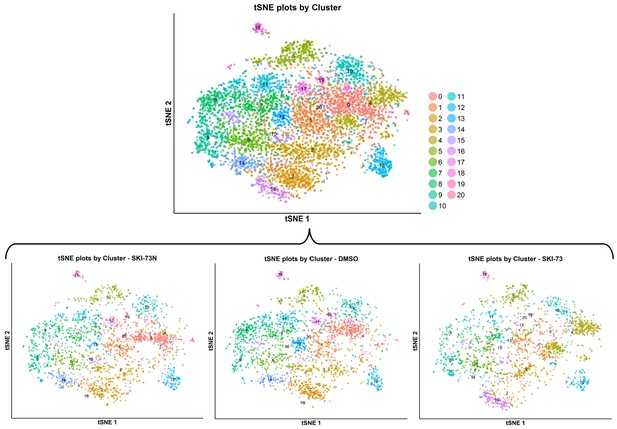
tSNE plot of the subpopulations of the classified G0/G1-phase cells after treatment with SKI-73N (6b), DMSO or SKI-73 (6a).
The combined cell population under the three treatment conditions (the top panel) was clustered into 21 subpopulations with a resolution granularity of 2.3. The tSNE plot of the 21 clustered subpopulations was split according to the three treatment origins of the cells: SKI-73N (6b), DMSO or SKI-73 (6a).
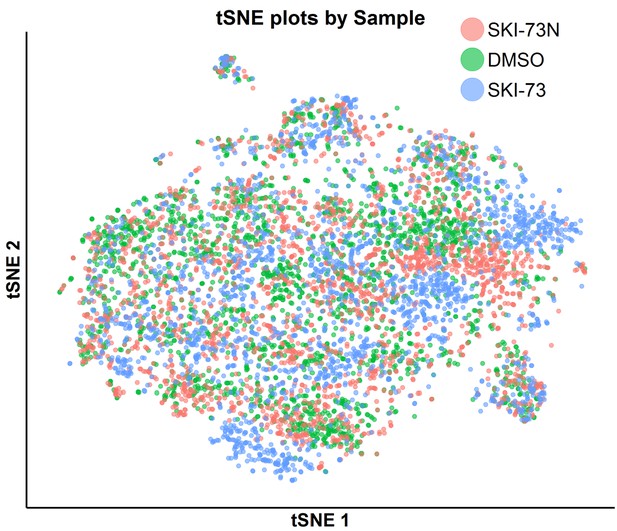
tSNE plot of the classified G0/G1-phase cells with awareness of their three treatment origins: SKI-73N (6b), DMSO or SKI-73 (6a).
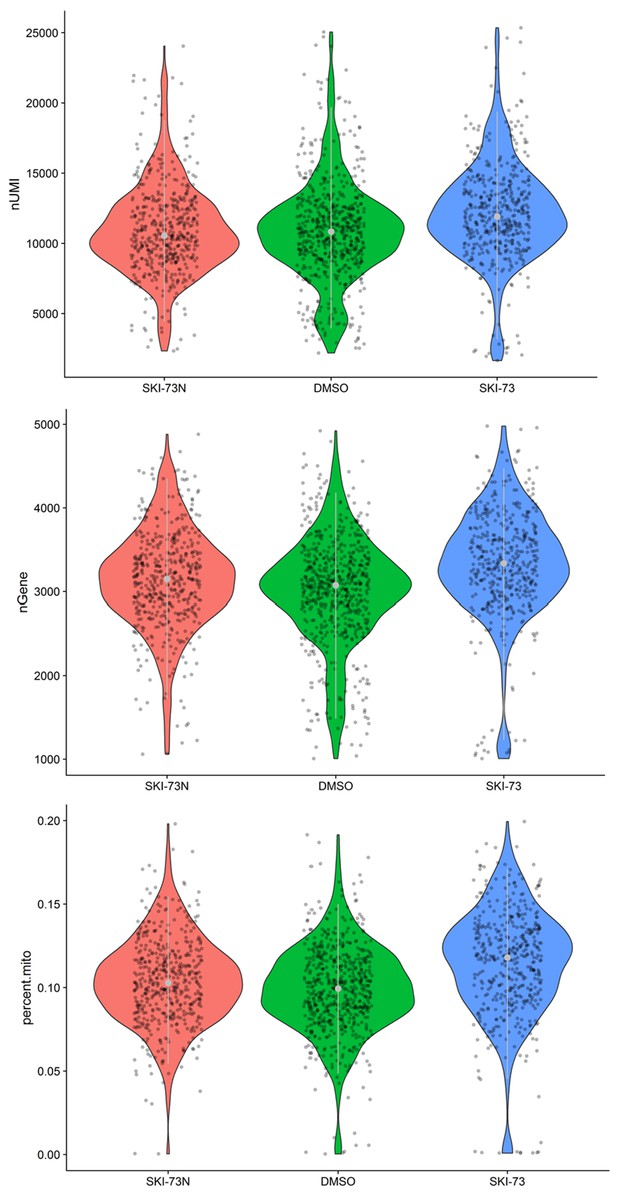
Quality control of the S-phase cells that were subjected to scRNA-seq analysis.
A subset of MDA-MB-231 cells after treatment with SKI-73N (6b), DMSO or SKI-73 (6a) was assigned as S-phase cells (as described in the Materials and methods). Thresholds of 1000~5000 genes and <20% mitochondrial RNA transcripts were used to select the S-phase cells for scRNA-seq analysis, with their UMIs (top), the number of genes per cell (middle) and the fraction of mitochondrial genes (bottom) shown.
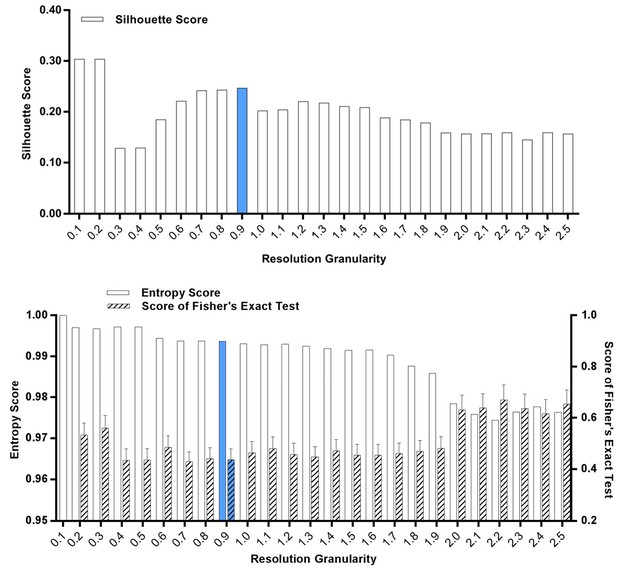
Silhouette analysis, entropy analysis and Fisher’s exact test of the classified S-phase cells after treatment with SKI-73N (6b), DMSO or SKI-73 (6a).
A resolution granularity of 0.9 for a low entropy score and a high Fisher’s exact test score were used for clustering.
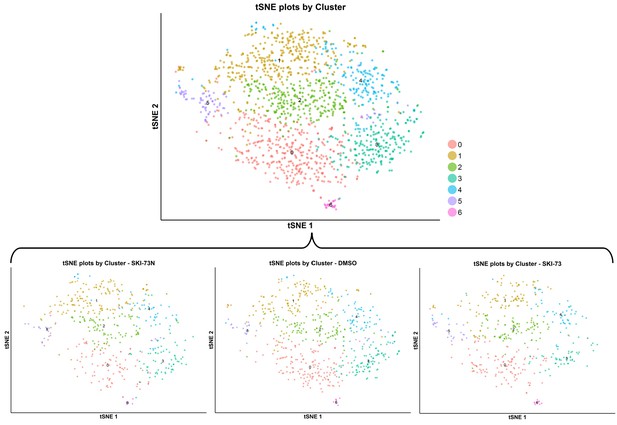
tSNE plot of the subpopulations of the classified S-phase cells after treatment with SKI-73N (6b), DMSO or SKI-73 (6a).
The combined population of cells from all three treatment conditions (the top panel) was clustered into seven subpopulations with the resolution granularity of 0.9. The tSNE plot of the seven clustered subpopulations was split according to their three treatment origins: SKI-73N (6b), DMSO and SKI-73 (6a).
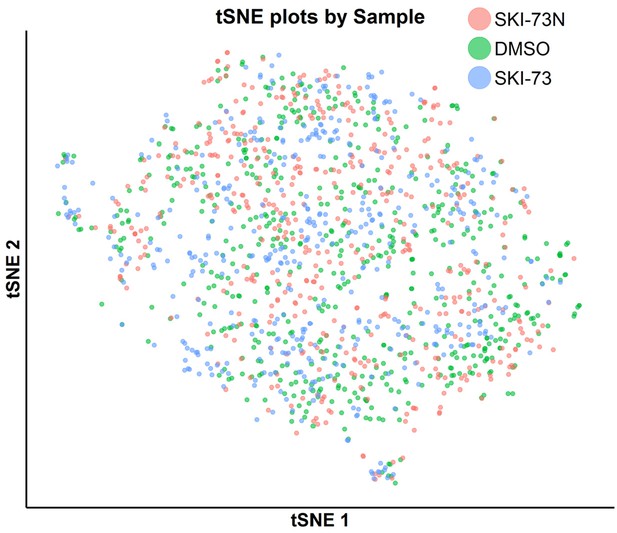
tSNE plot of the classified S-phase cells with awareness of their three treatment origins: SKI-73N (6b), DMSO and SKI-73 (6a).
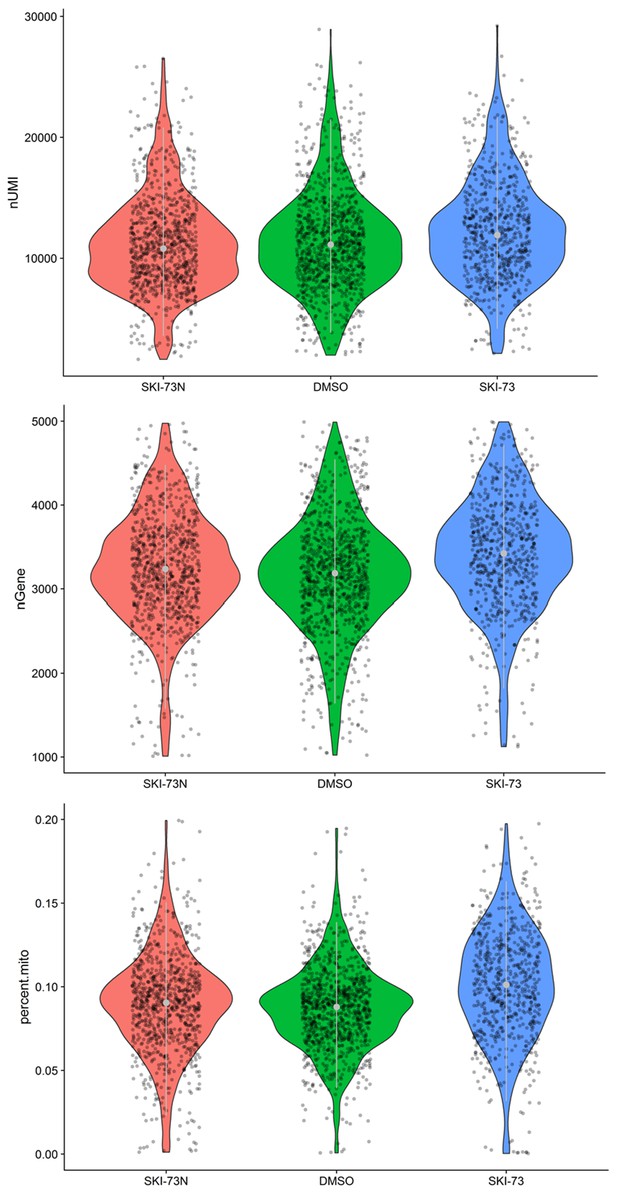
Quality control for the G2/M-phase cells that were subjected to scRNA-seq analysis.
A subset of MDA-MB-231 cells after treatment with SKI-73N (6b), DMSO or SKI-73 (6a) were designated as G2/M-phase cells, as described in the Materials and methods. Thresholds of 1000~5000 genes and <20% mitochondrial RNA transcripts were used to select the G2/M-phase cells for scRNA-seq analysis. Their UMIs (top), the number of genes per cell (middle) and the fraction of mitochondria genes (bottom) are shown.
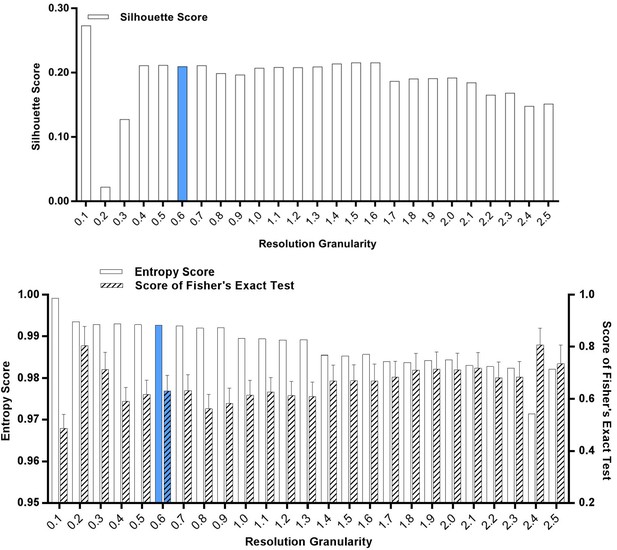
Silhouette analysis, entropy analysis and Fisher’s exact test of the classified G2/M-phase cells after treatment with SKI-73N (6b), DMSO or SKI-73 (6a).
A resolution granularity of 0.6 for a low entropy score and a high Fisher’s exact test score were used for clustering.
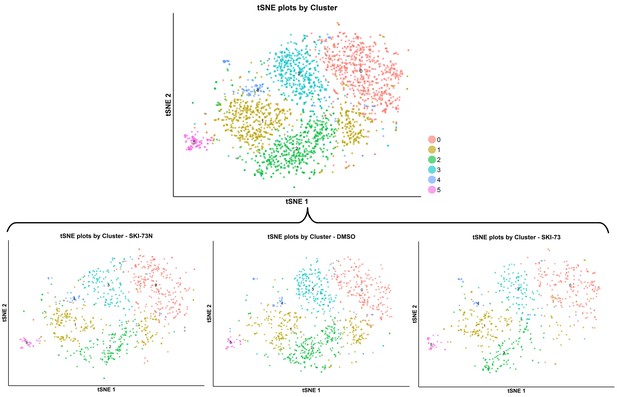
tSNE plot of the subpopulations of the classified G2/M-phase cells after the treatment with SKI-73N (6b), DMSO or SKI-73 (6a).
The combined cell population from the three treatment conditions (the top panel) was clustered into six subpopulations with the resolution granularity of 0.6. The tSNE plot of the six clustered subpopulations was split according to their three treatment origins: SKI-73N (6b), DMSO or SKI-73 (6a).
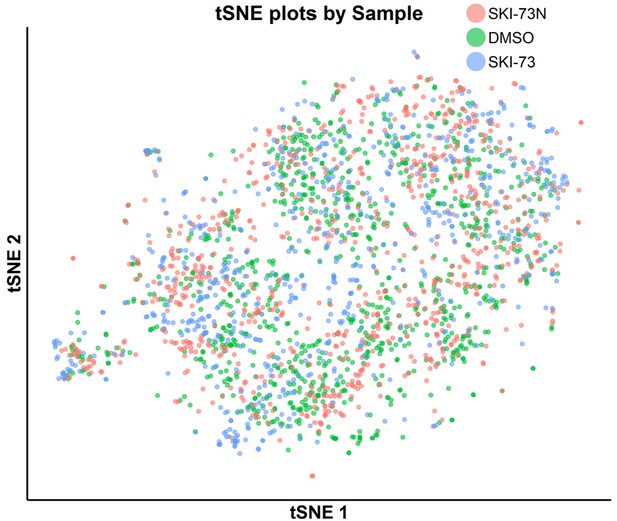
tSNE plot of the classified G2/M-phase cells with awareness of their three treatment origins: SKI-73N (6b), DMSO and SKI-73 (6a).
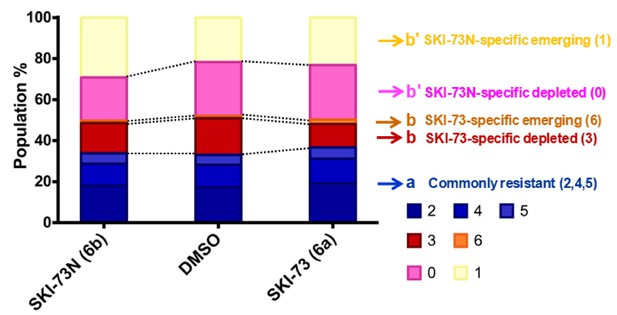
Population analysis for the S-phase cells after treatment with SKI-73 (6a) or SKI-73N (6b).
Depleted or emerging subpopulations were defined as the <0.8 and>1.2 ratios, respectively, for d(SKI-73N,i),total/d(DMSO,i),total or d(SKI-73,i),total/d(DMSO,i),total, as described in Materials and methods. Resistant subpopulations were defined within the 0.8~1.2 ratio range for both d(SKI-73N,i),total/d(DMSO,i),total and d(SKI-73,i),total/d(DMSO,i),total. Here commonly resistant (2, 4 and 5), SKI-73(6a)-specific depleted/emerging (3 and 6), and SKI-73N(6b)-specific depleted/emerging (0 and 1) subpopulations are color-coded in blue, red/orange, and pink/yellow, respectively.
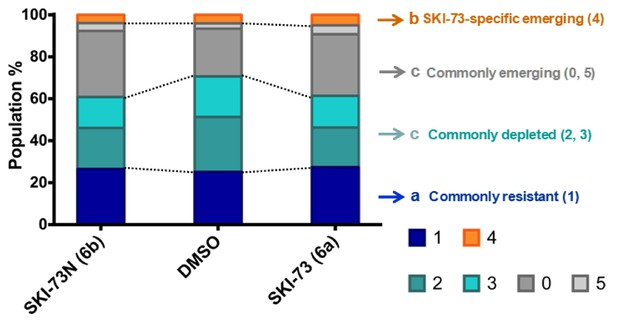
Population analysis of the G2/M-phase cells after treatment with SKI-73 (6a) or SKI-73N (6b).
Depleted or emerging subpopulations were defined as the <0.8 and>1.2 ratios, respectively, for d(SKI-73N,i),total/d(DMSO,i),total or d(SKI-73,i),total/d(DMSO,i),total, as described in Materials and methods. Resistant subpopulations were defined within the 0.8~1.2 ratio range for both d(SKI-73N,i),total/d(DMSO,i),total and d(SKI-73,i),total/d(DMSO,i),total. Here commonly resistant/depleted/emerging (1/2, 3/0, 5) and SKI-73(6a)-specific emerging (4) subpopulations are color-coded in blue/cyan/gray and orange, respectively.
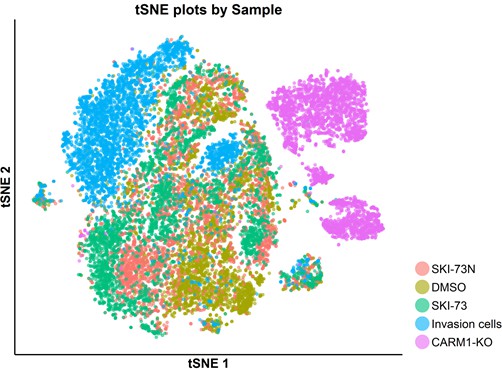
tSNE plot of the total cell population with five origins: SKI-73N(6b)-, DMSO- and SKI-73(6a)-treated cells, invasive cells and CARM1-KO cells.
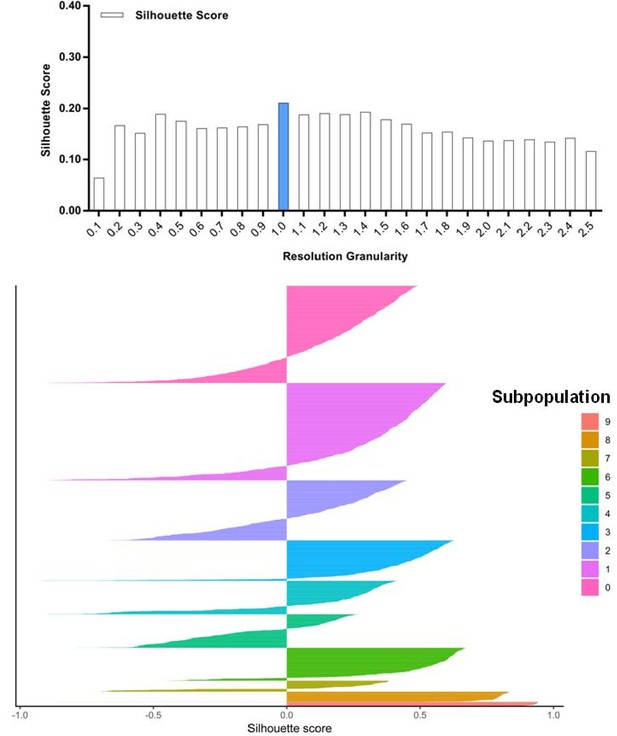
Silhouette analysis of invasive cells guided by the resolution granularity.
A resolution granularity of 1.0 for the highest Silhouette score was used for clustering and led to 10 subpopulations of the invasive cells.
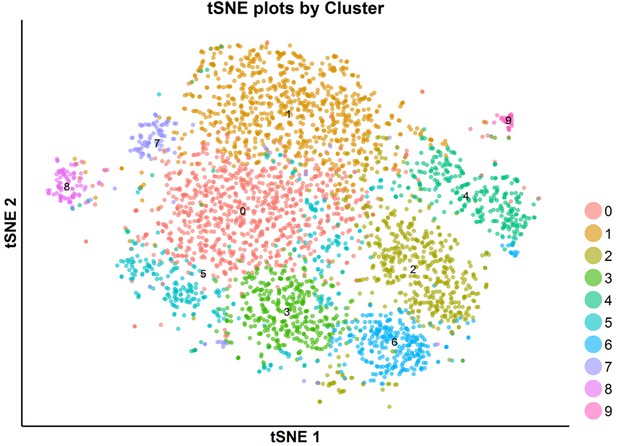
tSNE plot of the subpopulations of invasion cells.
The invasive cells were clustered into 10 subpopulations with a resolution granularity of 1.0.
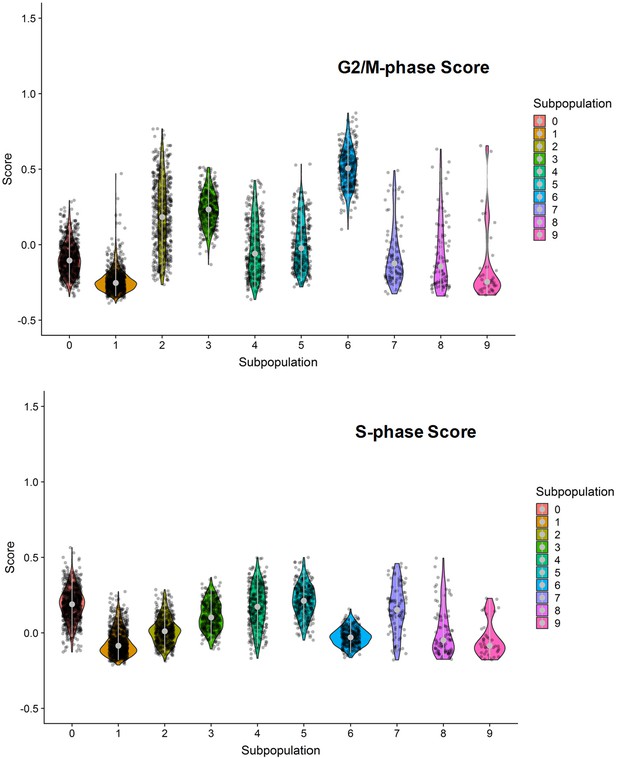
G2/M- and S-phase scores of the 10 clustered subpopulation of invasive cells.
Subpopulations 0, 2, 3 and 6 were dominated by G2/M- or S-phase scores.
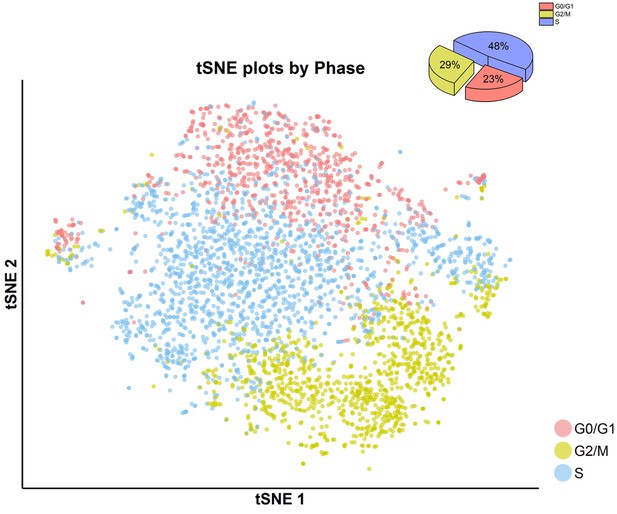
tSNE plot (center) with cell-cycle awareness and cell-cycle assignment (right upper corner) of invasive cells.
Individual cells were classified according to their cell-cycle scores, as described in the Materials and methods. Invasive cell showed more S- and G2/M-phase characters in comparison with the cells treated with DMSO, SKI-73N (6b) or SKI-73 (6a).
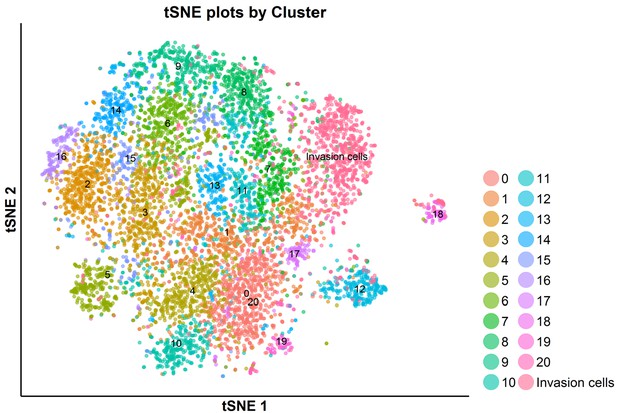
tSNE plot of the total G0/G1-phase cells analyzed by scRNA-seq: SKI-73N(6b)-, DMSO- and SKI-73(6a)-treated cells and invasive cells.
The G0/G1-phase cells upon the treatment with SKI-73N (6b), DMSO or SKI-73 (6a) were clustered into 21 subpopulations as described above.
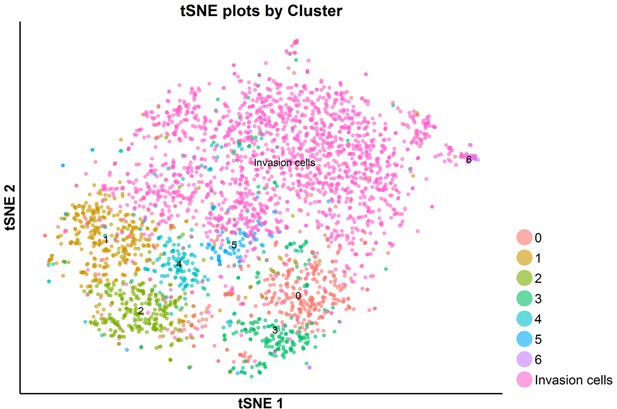
tSNE plot of all of the S-phase cells analyzed by scRNA-seq: SKI-73N(6b)-, DMSO- and SKI-73(6a)-treated cells and invasive cells.
The S-phase cells upon the treatment with SKI-73N (6b), DMSO or SKI-73 (6a) were clustered into seven subpopulations, as described above.
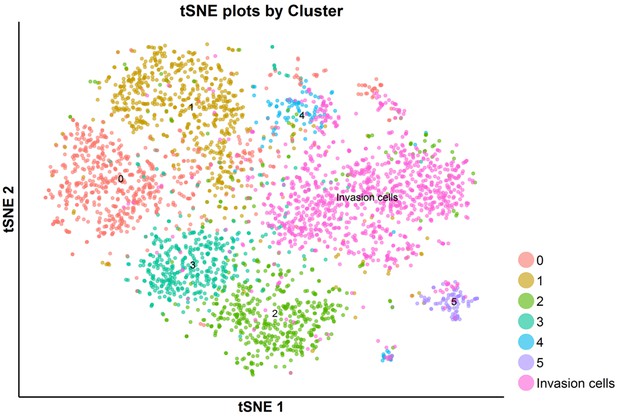
tSNE plot of the total G2/M-phase cells analyzed by scRNA-seq: SKI-73N(6b)-, DMSO- and SKI-73(6a)-treated cells and invasive cells.
The G2/M-phase cells upon the treatment with SKI-73N (6b), DMSO or SKI-73 (6a) were clustered into six subpopulations as described above.
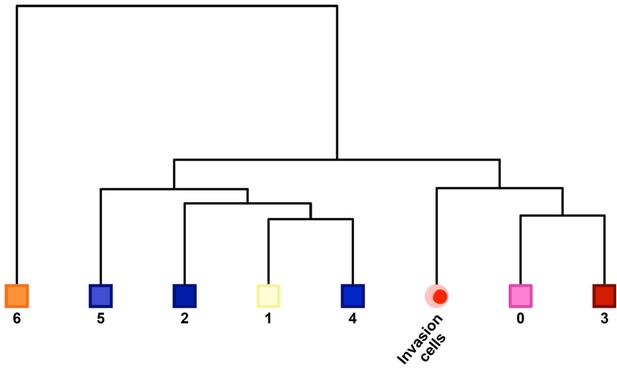
Unsupervised correlation analysis of the S-phase subpopulations.
For the SKI-73N(6b)-, DMSO- and SKI-73(6a)-treated S-phase cells (seven subpopulations) and the S-phase invasive cells, the correlation analysis of the eight subsets was conducted with the algorithm in the Seurat package, as described in Materials and methods. The subpopulations 0 and 3 are closely related to invasive cells.
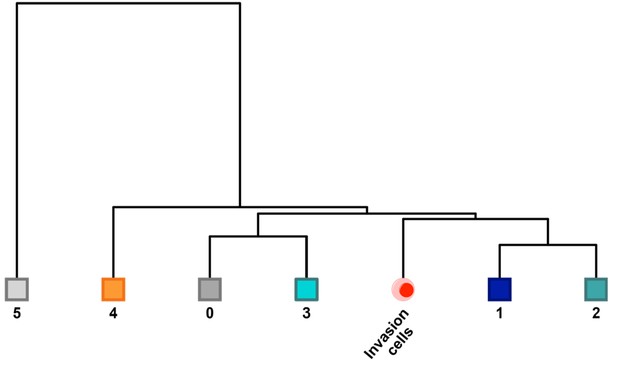
Unsupervised correlation analysis of the G2/M-phase subpopulations.
For the SKI-73N(6b)-, DMSO- and SKI-73(6a)-treated G2/M-phase cells (six subpopulations) and the G2/M-phase invasive cells, the correlation analysis of the seven subsets was conducted with the algorithm in the Seurat package, as described in Materials and methods. Subpopulations 1 and 2 are closely related to invasive cells.
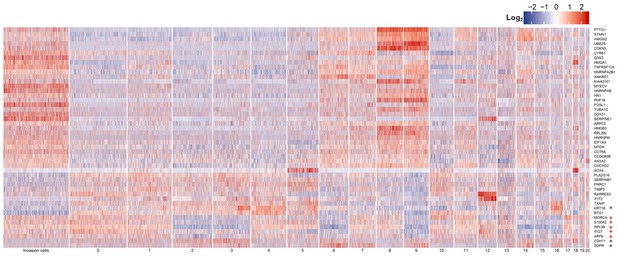
Heatmap of representative cancer-associated genes for comparison between invasive cells and the 21 subpopulations of G0/G1 cells.
From top to bottom, the top 20 upregulated and then top 10 downregulated transcripts in invasive cells with implicated cancer relevance as revealed by differential analysis and listed in Supplementary file 1-Table H. From the bottom, the top two downregulated (blue star) and the top four upregulated transcripts (red star) in both invasive cells and the most invasion-prone cells (Subpopulation 8 of G0/G1 cells) with implicated cancer relevance as revealed by differential analysis and listed in Supplementary file 1-Table L. ‘KRT18’ is the top downregulated transcript shared in Supplementary file 1-Table H, L.
Tables
Crystallography data and refinement statistics of the X-ray structures of CARM1 in complex with 5a.
Ligands | 5a |
---|---|
PDB Code | 6D2L |
Data collection | |
Wavelength (Å) | 0.97918 |
Space group | P21 |
Cell dimensions | |
a, b, c (Å) | 75.6, 155.6, 95.3 |
α, β, γ (°) | 90.0, 101.0, 90.0 |
Resolution (Å) | 50.0–2.00 |
Unique reflections | 142, 452 |
Redundancy | 4.5 |
Completeness (%) | 97.0 |
I/σ(I) | 10.4 |
Rsyma | 0.155 |
Rpim | 0.081 |
Refinement | |
No. protein molecules/ASU | 6 |
Resolution (Å) | 50.0–2.00 |
Reflections used or used/free | 139,748/1400 |
Rwork(%) | 18.7 |
Rfree(%) | 23.6 |
Average B value (Å2) | 30.8 |
Protein | 30.5 |
Compound | 34.8 |
Other | n/a |
Water | 35.5 |
Number of Atoms | 16,868 |
Protein | 15,819 |
Compound | 253 |
Other | n/a |
Water | 766 |
RMS bonds (Å) | 0.008 |
RMS angles (°) | 1.273 |
Wilson B (Å2) | 29.6 |
Ramachandran plot | |
Most favored (%) | 97.1 |
Additional allowed (%) | 2.9 |
Outliers (%) | 0.0 |
Crystallography data and refinement statistics for the X-ray structures of CARM1 in complex with 1.
Ligands | 1 |
---|---|
PDB code | 4IKP |
Data collection | |
Wavelength (Å) | 1.03321 |
Space group | P212121 |
Cell dimensions | |
a, b, c (Å) | 75.1, 98.8, 206.6 |
α, β, γ (°) | 90.0, 90.0, 90.0 |
Resolution (Å) | 50.0–2.00 |
Unique reflections | 104, 330 |
Redundancy | 8.1 |
Completeness (%) | 99.8 |
I/σ(I) | 30.4 |
Rsyma | 0.086 |
Rpim | 0.032 |
Refinement | |
No. protein molecules/ASU | 4 |
Resolution (Å) | 48.1–2.00 |
Reflections used or used/free | 103,958 |
Rwork(%) | 20.3 |
Rfree(%) | 23.1 |
Average B value (Å2) | 33.9 |
Protein | 33.4 |
Compound | 29.4 |
Other | n/a |
Water | 42.1 |
Number of atoms | 11,635 |
Protein | 10,770 |
Compound | 117 |
Other | n/a |
Water | 748 |
RMS bonds (Å) | 0.007 |
RMS angles (°) | 1.127 |
Wilson B (Å2) | 33.9 |
Ramachandran plot | |
Most favored (%) | 96.9 |
Additional allowed (%) | 3.03 |
Outliers (%) | 0.07 |
Reagent type (species) or resource | Designation | Source or reference | Identifiers | Additional information |
---|---|---|---|---|
Gene (Homo sapiens) | Human CARM1 catalytic domain | UniProtKB/Swiss-Prot:Q86 × 55.3 (positions140–480) | With an N-terminal6 × His tag in pFBOH-MHL, for crystallization | |
Cell line (Homo sapiens, female) | MDA-MB-231 (female) | ATCC | ||
Cell line (Homo sapiens, female) | MCF-7 (female) | ATCC | ||
Cell line (Homo sapiens, female) | MDA-MB-231; CARM1-KO | Wang et al., 2014a | ||
Cell line (Homo sapiens, female) | MCF-7; CARM1-KO | Wang et al., 2014a | ||
Software, algorithm | REFMAC | PMID: 15299926 | ||
Software, algorithm | SEquence Quality Control | https://github.com/ambrosejcarr/seqc.git | ||
Software, algorithm | fisher.test | http://mathworld.wolfram.com/FishersExactTest.html | ||
Software, algorithm | BuildClusterTree | https://rdrr.io/cran/Seurat | ||
Software, algorithm | FindMarkers | https://rdrr.io/cran/Seurat | ||
Software, algorithm | DoHeatmap | https://rdrr.io/cran/Seurat |
Additional files
-
Supplementary file 1
Tables A–L.
(A) IC50 values of SAH, SNF, 1, 2a, 2b, 5a and 5b against CARM1. (B–D) Structural analysis of CARM1 in complex with 1, SNF and SAH. (E–L) Analysis of single-cell RNA-seq data.
- https://cdn.elifesciences.org/articles/47110/elife-47110-supp1-v2.xlsx
-
Transparent reporting form
- https://cdn.elifesciences.org/articles/47110/elife-47110-transrepform-v2.docx