The NTP generating activity of pyruvate kinase II is critical for apicoplast maintenance in Plasmodium falciparum
Figures
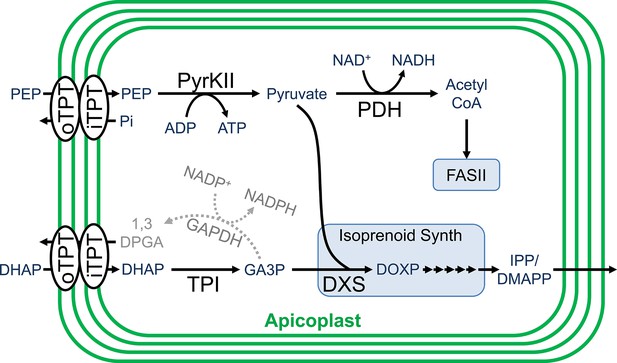
Carbon metabolism in the apicoplast.
Carbon backbones are imported into the apicoplast organelle in the form of 3-carbon phosphates by the apicoplast membrane outer triose phosphate transporter (oTPT) and inner triose phosphate transporter (iTPT). Pyruvate kinase II (PyrKII) converts the substrates phophoenolpyruvate (PEP) and adenosine diphosphate (ADP) into the products pyruvate and adenosine triphosphate (ATP). Pyruvate is used by PDH to generate acetyl CoA, which is fed into the FASII fatty acid biosynthesis pathway. Triose phosphate isomerase (TPI) converts dihydroxyacetone phosphate (DHAP) into glyceraldehyde-3-phosphate (GA3P). Pyruvate and GA3P are used by 1-deoxy-D-xylulose-5-phosphate (DOXP) synthase (DXS) to form DOXP, which is further converted by a series of enzymes to form the essential isoprenoid precursors isopentenyl pyrophosphate (IPP) and dimethylallyl pyrophosphate (DMAPP). Colored in gray, a hypothetical glyceraldehyde 3-phosphate dehydrogenase (GAPDH) could convert GA3P into 1,3-diphosphoglycerate (1,3-DPGA).
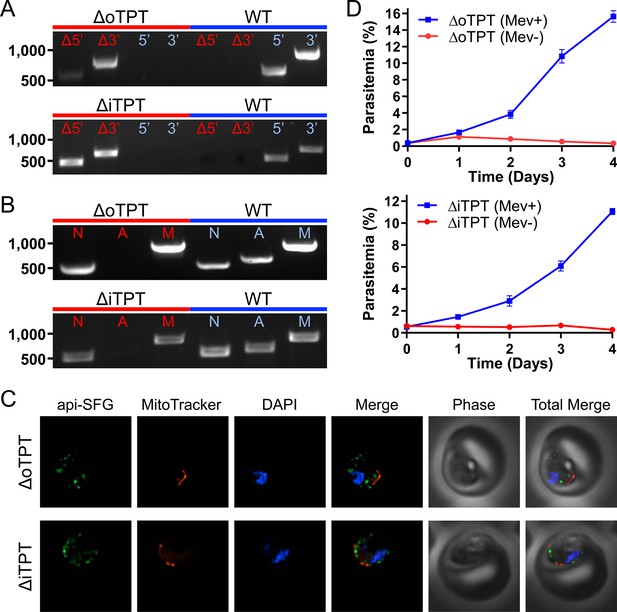
Characterization of the PfMev Δotpt and Δitpt parasite lines.
(A) Genotyping PCR confirming deletion of oTPT or iTPT, with amplification demonstrating integration of the drug resistance cassette at the 5’ and 3’ loci of the target gene (Δ5’ and Δ3’), and lack of wild type parasites due to failure to amplify at the wild type 5’ and 3’ loci in the PfMev Δotpt or Δitpt parasites, as compared to the parental control (WT). (B) Attempted PCR detection of the ldh, sufB, and cox1 genes from the nuclear (N), apicoplast (A), and mitochondrial (M) genomes, respectively. We failed to amplify sufB from the PfMev Δotpt or Δitpt parasite lines, indicating loss of the apicoplast organelle genome. (C) Live epifluorescence microscopy of the PfMev Δotpt and Δitpt parasite lines, which express api-SFG (green), and are also stained with MitoTracker (red) and DAPI (blue). Microscopy images represent fields that are 10 µM long by 10 µM wide. (D) Growth curves of the PfMev Δotpt and Δitpt parasite lines cultured with or without 50 μM mevalonate. Error bars represent the standard error of the mean from two independent experiments, each conducted in quadruplicate.
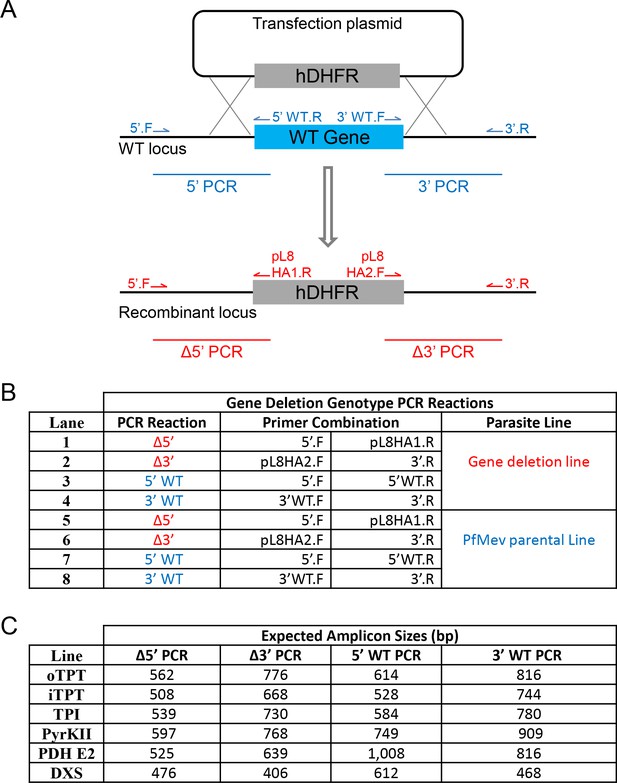
Schematic and details describing gene deletion experiments.
(A) Schematic representation showing how targeted genes (WT Gene) were replaced with the hDHFR drug resistance cassette. The positions of genotyping PCR products designed to identify the wild-type locus (blue) or the recombinant locus (red) are show. (B) Table demonstrating how diagnostic primers were used to validate the genotypes of knockout parasite lines. (C) Table showing the expected amplicon sizes for the genotype PCR reactions shown in Figures 2A, 3A and 4A.
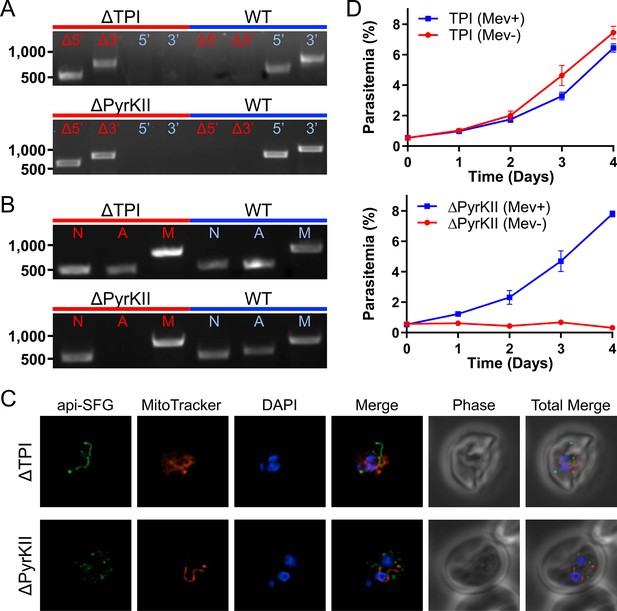
Characterization of the PfMev Δtpi and ΔpyrkII parasite lines.
(A) Genotyping PCR confirming deletion of TPI or PyrKII, with amplification demonstrating drug cassette integration at the 5’ and 3’ loci of the target gene (Δ5’ and Δ3’), and lack of wild-type parasites due to failure to amplify at the wild-type 5’ and 3’ loci in the PfMev Δtpi or ΔpyrkII parasite lines, as compared to the parental control (WT). (B) Attempted PCR detection of the ldh, sufB, and cox1 genes from the nuclear (N), apicoplast (A), and mitochondrial (M) genomes, respectively. We were successful in amplifying sufB from the PfMev Δtpi parasite line, indicating retention of the apicoplast organelle genome. We failed to amplify sufB from the PfMev ΔpyrkII parasite line, indicating loss of the apicoplast organelle genome. (C) Live epifluorescence microscopy of the PfMev Δtpi and ΔpyrkII parasite lines. These parasite lines express api-SFG (green) and are also stained with MitoTracker (red) and DAPI (blue). Microscopy images represent fields that are 10 µM long by 10 µM wide. (D) Growth curves of the PfMev Δtpi and ΔpyrkII parasite lines cultured with or without 50 μM mevalonate. Error bars represent the standard error of the mean from two independent experiments, each conducted in quadruplicate.
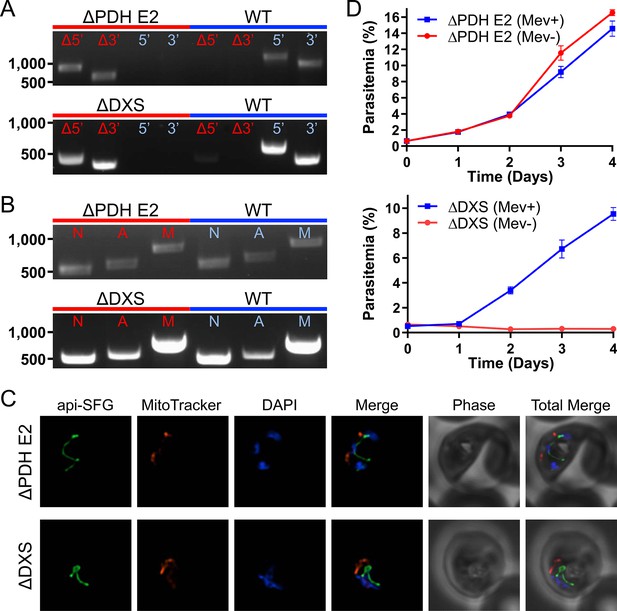
Characterization of the PfMev Δpdh E2 and Δdxs parasite lines.
(A) Genotyping PCR confirming deletion of PDH E2 or DXS, with amplification demonstrating drug cassette integration at the 5’ and 3’ loci of the target gene (Δ5’ and Δ3’), and lack of wild-type parasites due to failure to amplify at the wild-type 5’ and 3’ loci in the PfMev Δpdh E2 or Δdxs parasites, as compared to the parental control (WT). (B) Attempted PCR detection of the ldh, sufB, and cox1 genes from the nuclear (N), apicoplast (A), and mitochondrial (M) genomes, respectively. We successfully amplified sufB from the PfMev Δpdh E2 and Δdxs parasite lines, indicating retention of the apicoplast organelle genome. (C) Live epifluorescence microscopy of the PfMev Δpdh E2 and Δdxs parasite lines. These parasite lines express api-SFG (green) and are also stained with MitoTracker (red) and DAPI (blue). Microscopy images represent fields that are 10 µM long by 10 µM wide. (D) Growth curves of the PfMev Δpdh E2 and Δdxs parasite lines cultured with or without 50 μM mevalonate. Error bars represent the standard error of the mean from two independent experiments, each conducted in quadruplicate.
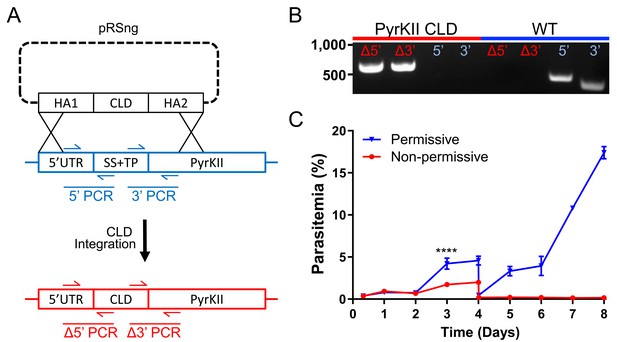
Growth of the PfMev CLD-PyrKII line under permissive and non-permissive conditional localization conditions.
(A) Schematic representation showing how the endogenous signal sequence (SS) and transit peptide (TP) of PyrKII were replaced with the CLD using plasmid pRSng. The positions of genotyping PCR products designed to identify the wild type locus (blue) or the recombinant locus (red) are shown. (B) Amplification of the 5’ and 3’ loci of the integrated region (Δ5’ and Δ3’) demonstrates integration of the CLD (PyrKII CLD), and lack of wild type parasites due to failure to amplify at the wild type 5’ and 3’ regions of the endogenous gene, as compared to the parental control (WT). (C) Synchronized PfMev CLD-PyrKII parasites were seeded at 0.5% parasitemia and cultured in the permissive (no Shield1) or non-permissive (500 nM Shield1) conditions for 8 days. Parasites were collected daily for determination of parasitemia by flow cytometry and were cut 1:10 on day 4. Treatment of the parasites with Shield1 resulted in a relatively rapid inhibition of parasite growth beginning at day 3 (two-way ANOVA, followed by Bonferroni’s correction; ****, p<0.0001). Error bars represent the standard error of the mean from two independent experiments, each conducted in quadruplicate.
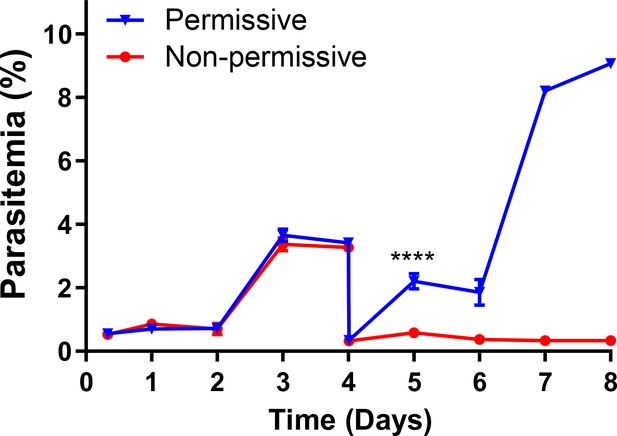
Growth of the PfMev PyrKII TetR-DOZI line under permissive and non-permissive knockdown conditions.
Synchronized PfMev PyrKII TetR-DOZI parasites were seeded at 0.5% parasitemia and cultured under permissive (with aTc) or non-permissive (no aTc) conditions for 8 days. Parasites were collected daily for determination of parasitemia by flow cytometry and were cut 1:10 on day 4. Upon the removal of aTc, parasites exhibited a decrease in growth that became significant on day 5 (two-way ANOVA, followed by Bonferroni’s correction; ****, p<0.0001). Error bars represent the standard error of the mean from two independent experiments, each conducted in quadruplicate.
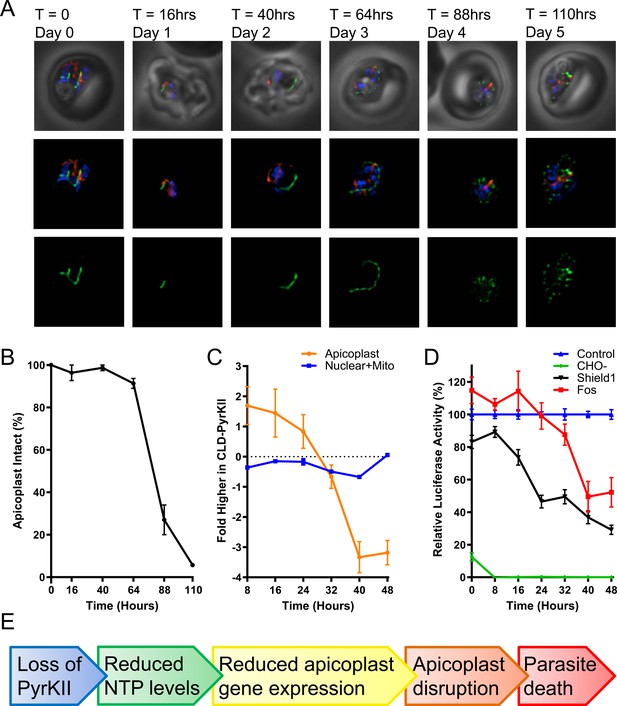
Characterization of PfMev CLD-PyrKII parasites after mislocalization of PyrKII.
(A) Live epifluorescence microscopy of the PfMev CLD-PyrKII parasite line after Shield1 treatment. This parasite line expresses api-SFG (green) and is also stained with MitoTracker (red) and DAPI (blue). Representative images show the state of the apicoplast organelle at the corresponding time points. Microscopy images represent fields that are 10 µM long by 10 µM wide. (B) Graph of the percentage of disrupted apicoplast organelles in synchronized PfMev CLD-PyrKII parasites after Shield1 treatment. At each time point, a minimum of 15 microscopy images were taken to determine apicoplast morphology (intact or disrupted) based on api-SFG fluorescence. The percentage of parasites containing an intact apicoplast dropped precipitously between the second and third growth cycle of the parasite, corresponding to the 64 and 88 hr time points. Error bars represent the standard deviation from three independent experiments with at least 20 observations each. (C) Synchronized ring-stage PfMev CLD-PyrKII parasites were treated with Shield1, with samples collected every 8 hr for microarray analysis (biological triplicates were collected). Nuclear and mitochondrial mRNA levels (blue) were compared to an untreated control and plotted as the fold difference. The same analysis for apicoplast genes (orange) shows that there is a sharp reduction in RNA levels in the treated parasites between 24 and 40 hr after Shield1 treatment. (D) Synchronized ring-stage PfMev CLD-PyrKII api-luciferase parasites were prepared for a 48 hr time course under four conditions, each of which contained 500 nM aTc: exchange into glucose-free medium (CHO-, green), 500 nM Shield1 treatment (Shield1, black), 50 µM fosmidomycin treatment (Fos, red), and no treatment (Control, blue). Samples were collected every 8 hr for analysis of luciferase activity (biological triplicates were collected, each with three technical replicates). We observed a significant reduction in luminescence ~8–24 hr after triggering mislocalization of PyrKII with Shield1. For parasites treated with fosmidomycin, luminescence levels did not meaningfully decrease until 24–40 hr, while levels in parasites cultured in glucose-free medium dropped within 8 hr. For all treatment groups, luminescence levels were compared to an untreated parasite control. Error bars represent the standard error of the mean. (E) Proposed progression of events leading from loss of PyrKII to parasite death.
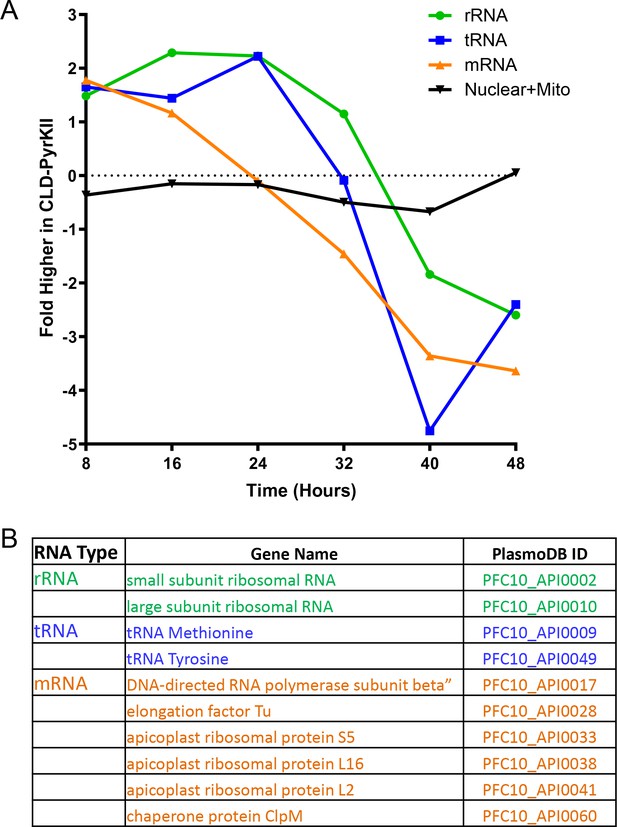
Analysis of apicoplast RNA levels in PfMev CLD-PyrKII parasites after treatment with Shield1.
(A) The microarray data shown in Figure 6C are plotted showing the averages of each RNA type, indicating that mRNAs (orange) seem to decrease in abundance prior to tRNA (blue) and rRNA (green) species. (B) The apicoplast RNAs studied in the microarray analysis are listed with their PlasmoDB identification numbers.
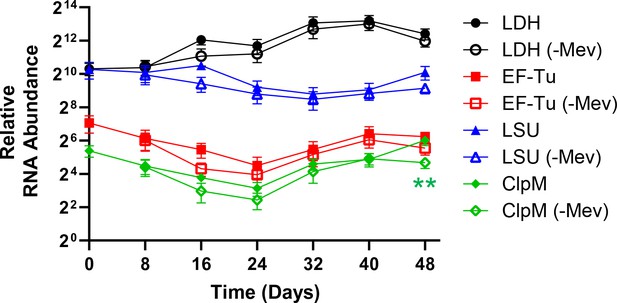
Mevalonate dependence of apicoplast and nuclear transcripts in PfMev Δdxs parasites quantified using RT-qPCR.
The relative transcript abundance of nuclear-encoded LDH and apicoplast encoded EF-Tu, LSU, and ClpM is shown in PfMev Δdxs parasites cultured with mevalonate (filled symbols) or without mevalonate (open symbols). Samples from biological duplicates were collected at 8 hr intervals for 48 hr followed by RNA extraction and cDNA synthesis. RT-qPCR was performed with technical triplicates and quantified relative to samples of pure parasite DNA. Mevalonate deprivation and loss of isoprenoid synthesis does not cause a significant reduction in transcript abundance until the 48 hr time point, indicating that reduced apicoplast transcription (as observed in the CLD-PyrKII line) is not an early consequence of general metabolic dysfunction (two-way ANOVA, followed by Bonferroni’s correction; **, p<0.01). Error bars represent the standard error of the mean (SEM).
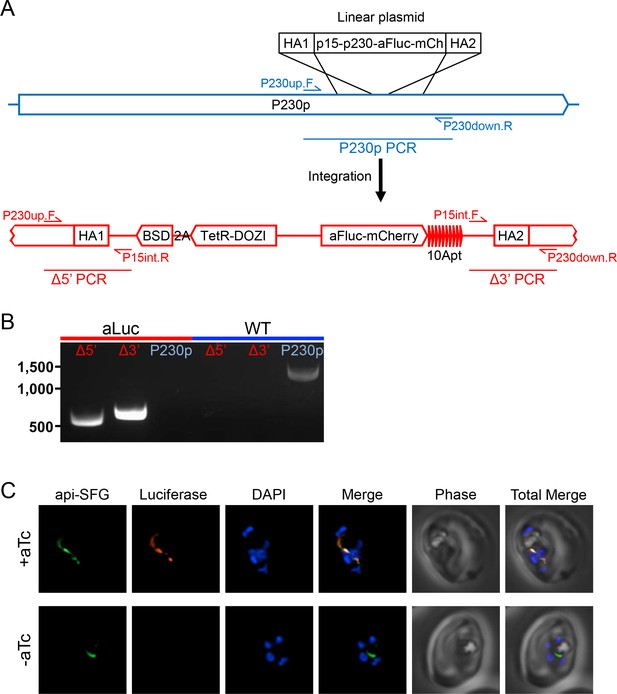
Design and validation of the PfMev CLD-PyrKII api-luciferase parasite line.
(A) Schematic representation showing how p15-p230-aFluc-mCh plasmid linearized with SalI digestion was integrated into the p230p gene. Linearization results in the homology arms (HA1 and HA2) flanking the contents of the plasmid including the apicoplast luciferase mCherry construct (aFluc-mCherry) and its 3’ ten-aptamer array (10Apt). The drug selection enzyme blasticidin-S deaminase (BSD) is separated from the TetR-DOZI fusion protein by a viral 2A skip peptide (2A). The positions of genotyping PCR products designed to identify the wild type locus (blue) or the recombinant locus (red) are show. (B) Diagnostic PCR products using the four primers shown in panel A of this figure. Amplicons corresponding to the 5’ (Δ5’) and 3’ (Δ3’) integration sites are found in the PfMev CLD-PyrKII api-luciferase parasite line (red), but not the parental line (WT). Similarly, an amplicon corresponding to the native P230p locus (P230p) is only found in the parental line (blue). (C) Live epifluorescence microscopy of the PfMev CLD-PyrKII api-luciferase parasite line. This parasite line expresses api-SFG (green) and an apicoplast-targeted luciferase tagged with mCherry (red) and are also stained with DAPI (blue). Luciferase expression is only detectable in parasites treated with 500 nM aTc (top row) and co-localizes with the apicoplast api-SFG with the following Manders coefficients: M1 (mCherry in SFG)=0.70, SD = 0.12, n = 23; and M2 (SFG in mCherry)=0.87, SD = 0.08, n = 23. Microscopy images represent fields that are 10 µM long by 10 µM wide.
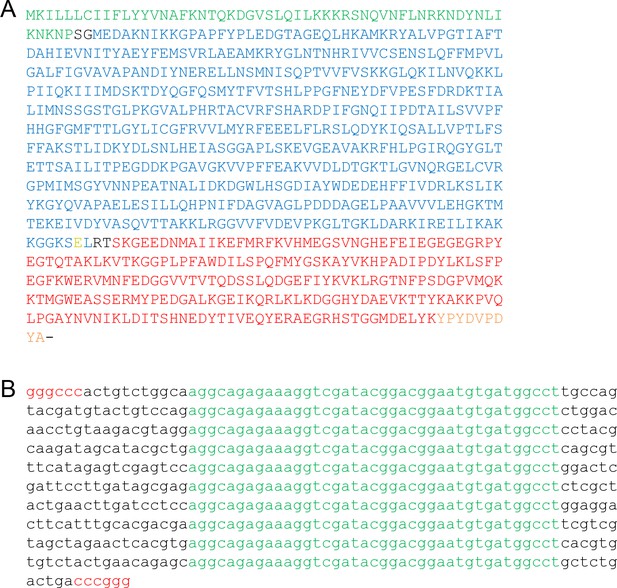
Sequences found in plasmid p15-p230-aFluc-mCh.
(A) The firefly luciferase protein (blue) is shown with an apicoplast-targeting peptide (green), mCherry fusion protein (red) and HA tag (orange). The K549E mutation to limit peroxisomal trafficking is colored yellow. (B) The sequence of the modified 10 aptamer sequence is shown flanked by endonuclease sites (red). The 23 bp aptamer regions (green) are separated by spacers with unique sequences (black).
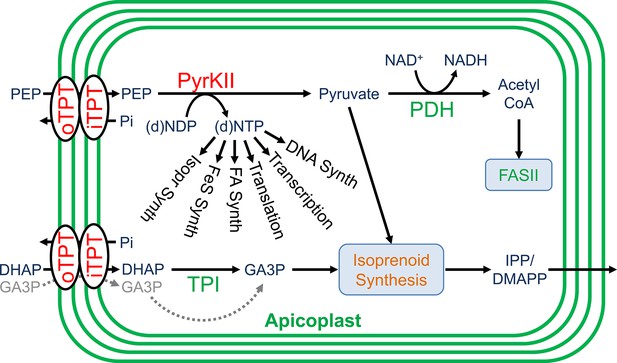
Roles of carbon metabolism proteins in the apicoplast.
Apicoplast proteins and pathways are colored according to gene deletion results (dispensable = green, essential = orange, and essential for apicoplast maintenance = red). Pyruvate kinase II (PyrKII) generates nucleoside triphosphate (NTP) products used for transcription, translation, and for several metabolic pathways including the synthesis of fatty acids (FA), isoprenoids (isopr) and iron-sulfur clusters (FeS). The dispensability of the triose phosphate isomerase (TPI) suggests that glyceraldehyde-3-phosphate (GA3P) may be directly imported by oTPT/iTPT for use in the isoprenoid synthesis pathway (gray arrow). This result also indicates that malaria parasites do not rely on a two-enzyme reductive pathway involving TPI and a hypothetical GAPDH enzyme.
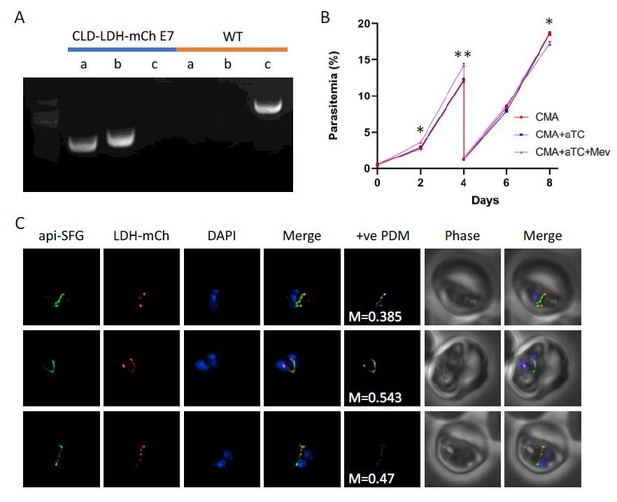
Expression and localization of lactate dehydrogenase (LDH) in the apicoplast.
A) Genotyping PCR confirming insertion of CLD-LDH-mCherry (clone E7), with amplification demonstrating plasmid integration at the 5’ and 3’ loci of the P230p locus (lanes a, b), and lack of wild-type parasites due to failure to amplify at the wild type locus (lane c), as compared to the parental control (WT). B) Growth curve of the CLD-LDH-mCh parasite line cultured with 500nM aTC (aTC), aTC and 50μM mevalonate (Mev), or without supplements (CMA). C) Live epifluorescence microscopy of the CLD-LDH-mCherry parasite line. This line expresses api-SFG (green) and CLD-LDH-mCherry (red), while DAPI (blue) marks the nucleus. Microscopy images represent fields that are 10μM long by 10μM wide and include Mander’s values and positive product of the differences from the mean (+vePDM) panels.
Tables
Enzyme kinetics of pure recombinant PyrKII as compared to T. gondii PyrKII.
Activity of PyrKII was measured using various NDPs and dNDPs as substrates. Values and standard deviations from triplicate measurements are reported for the kinetic parameters Kcat and Km. The ratio Kcat/Km represents the catalytic efficiency. Kinetic parameters for T. gondii PyrKII from Saito and coworkers are shown for comparison (Saito et al., 2008).
Substrate | Kcat (min−1) | Km (mM) | Kcat/Km (mM−1 min−1) | Organism |
---|---|---|---|---|
ADP | 793 + / - 186 | 0.25 + / - 0.11 | 3200 | P. falciparum |
GDP | 397 + / - 45 | 0.07 + / - 0.04 | 5890 | |
CDP | 438 + / - 69 | 1.01 + / - 0.13 | 432 | |
UDP | 252 + / - 40 | 0.82 + / - 0.22 | 306 | |
dADP | 401 + / - 31 | 0.38 + / - 0.04 | 1066 | |
dGDP | 90 + / - 5 | 0.28 + / - 0.03 | 318 | |
dCDP | 78+ / - 3 | 0.52 + / - 0.12 | 149 | |
ADP | 2850 + / - 80 | 8.0 + / - 0.40 | 360 | T. gondii |
GDP | 6600+ / - 240 | 0.05 + / - 0.006 | 121,320 |
-
Table 1—source data 1
Nucleotide sequence of P. falciparum PyrKII codon harmonized for expression in E. coli.
The endonuclease sites used for cloning (EcoRI and HindIII) are shown in lower case italics and the stop codon is highlighted in red.
- https://cdn.elifesciences.org/articles/50807/elife-50807-table1-data1-v2.pdf
-
Table 1—source data 2
Purification of recombinant P. falciparum PyrKII.
A maltose binding protein (MBP), along with a histidine (His) tag were appended to the N-terminus of harmonized PyrKII sequence and expressed in E. coli. The MBP-His-PyrkII fusion protein (126,273 Da) can be seen in lane 7, after purification using an MBP column. The TEV cleaved product, His-PyrKII (83,014 Da) can be seen in lane 8. Lane 10 shows the purified product and a minor band consistent with some covalent dimer (166 kDa).
- https://cdn.elifesciences.org/articles/50807/elife-50807-table1-data2-v2.pdf
-
Table 1—source data 3
Nucleotide substrate kinetic plots for His-PyrKII.
Dose-response curves from triplicate data for seven nucleotide substrates are shown fitted with hyperbolic curves based on the Michaelis-Menton equation. Insets show the same data plotted in double reciprocal (Lineweaver-Burk) plots. Error bars represent the standard error of the mean and the program Prism (GraphPad) was used to generate the plots in this figure.
- https://cdn.elifesciences.org/articles/50807/elife-50807-table1-data3-v2.pdf
-
Table 1—source data 4
Nucleotide substrate kinetic plots for His-PyrKII.
Dose-response curves from triplicate data for seven nucleotide substrates are shown fitted with hyperbolic curves based on the Michaelis-Menton equation. Insets show the same data plotted in double reciprocal (Lineweaver-Burk) plots. Error bars represent the standard error of the mean and the program Prism (GraphPad) was used to generate the plots in this figure.
- https://cdn.elifesciences.org/articles/50807/elife-50807-table1-data4-v2.pdf
Additional files
-
Supplementary file 1
Oligonucleotides used in this study.
- https://cdn.elifesciences.org/articles/50807/elife-50807-supp1-v2.xls
-
Supplementary file 2
Analysis of microarray data.
- https://cdn.elifesciences.org/articles/50807/elife-50807-supp2-v2.xls
-
Transparent reporting form
- https://cdn.elifesciences.org/articles/50807/elife-50807-transrepform-v2.docx