Relaxation of synaptic inhibitory events as a compensatory mechanism in fetal SOD spinal motor networks
Figures
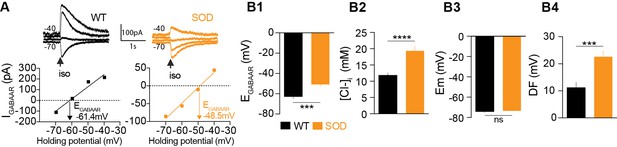
Alterations in chloride homeostasis.
(A) Representative traces of isoguvacine (Iso) responses illustrating the reversal of the evoked GABAAR-related current (EGABAAR) in WT and SOD MNs. Holding voltage −70 mV, −60 mV, −50 mV and −40 mV. I/V relationships, plotted below the traces, revealed that EGABAAR was −61.4 mV and −48.5 mV in the representative WT and SOD MNs, respectively. (B1) Mean EGABAAR was significantly lower in E17.5 WT (n = 25) compared to SOD (n = 24) MNs from the same litter. (B2) Accordingly, the mean [Cl-]i, calculated from individual EGABAAR values, indicated that SOD MNs had a higher [Cl-]i than WT. (B3) Mean resting membrane potential (Em) did not differ in WT and SOD MNs. (B4) Mean driving force (DF) for chloride ions was doubled in SOD MNs compared to WT MNs. Values illustrated were from the same set of MNs. ****p<0.0001, ***p<0.001, ns non-significant, Mann Whitney test.
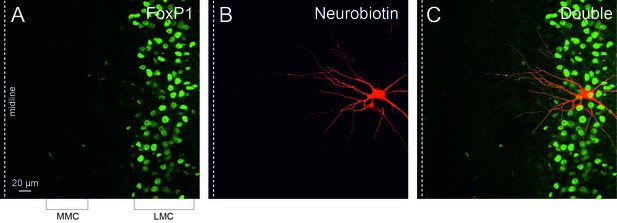
Recorded MNs belong to the lateral motor column (LMC).
(A) FoxP1 transcription factor expressed in the LMC (green staining of nuclei). (B) A recorded MN filled with neurobiotin (red staining). (C) Merged image showing the LMC location of the recorded MN. MMC: medial motor cortex. Midline is indicated by a dashed line on the left of each panel.
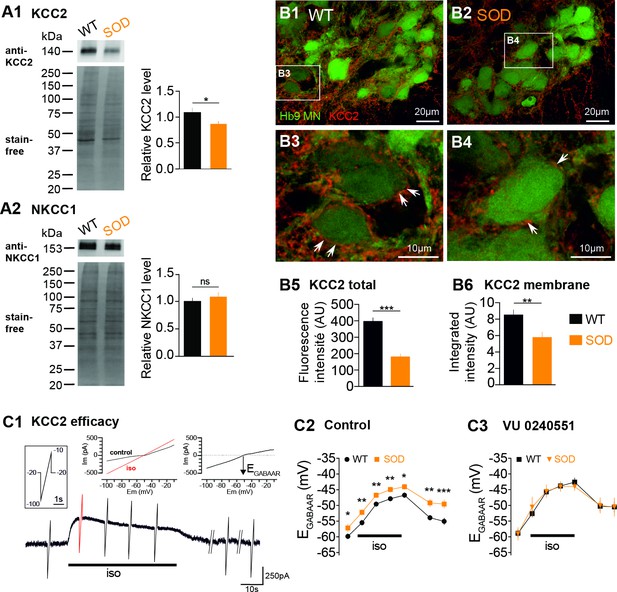
Expression of KCC2 and NKCC1 in the E17.5 SOD spinal cord.
(A1) Analysis of the KCC2 protein in SOD and WT SCs. The stain-free staining of total proteins loaded (lower left panel) was used as the normalization control to quantify the ~140 kDa KCC2 band (upper left panel). Right panel: quantification of KCC2 WB. (A2) Analysis of the NKCC1 protein in SOD and WT SCs. The ~150 kDa NKCC1 band (upper left panel) was analyzed. Quantification of NKCC1 WB. Three loads (20 μg of protein) from SOD and WT lumbar spinal cord extracts were used, each load including five individual SCs from four different litters. (B1–B4) Representative immunohistochemical KCC2 (red) staining in WT (B1–B3) and SOD (B2–B4) lumbar 0.2 µm thickness optical sections (L3-L5 level) containing Hb9-eGFP MNs (green). Images correspond to x60 confocal acquisitions. White arrows point the KCC2 labeling surrounding spinal MNs. (B5–B6) Quantification of the global KCC2 staining (B5) in the MN area and membrane KCC2 staining (B6) in E17.5 SOD and WT SCs. (C1) Illustration of the protocol applied to assess the KCC2 efficacy as explained in the Materials and method section. (C2–C3) Evolution of ECl values with time during the isoguvacine (iso) application in control aCSF (C2) and in the presence of the highly specific KCC2 blocker VU0240551 (10 µM) (C3). ***p<0.001, **p<0.01, *p<0.05, ns non-significant, Mann Whitney test.
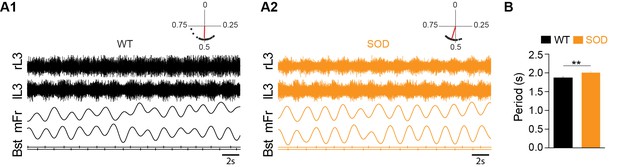
Motor activity in the E17.5 SOD spinal cord.
(A) Fictive locomotor-like activity evoked by 5-HT/NMDA/DA (see Materials and methods) recorded from contralateral lumbar ventral roots (lL3, rL3; two first traces) displayed regular alternating activities (see circular plots above each raw data panel) in both WT (A1) and SOD (A2) SCs. Below the raw data are traces of the floating mean spike frequencies (mFr) and the corresponding troughs of activity (burst start, Bst) from which the phase relationships between the left and right SC sides were calculated (circular plots). (B) The cycle period was significantly longer in SOD SCs (n = 9) compared to WT (n = 8) SCs. **p<0.01, ns non-significant, Mann Whitney test.
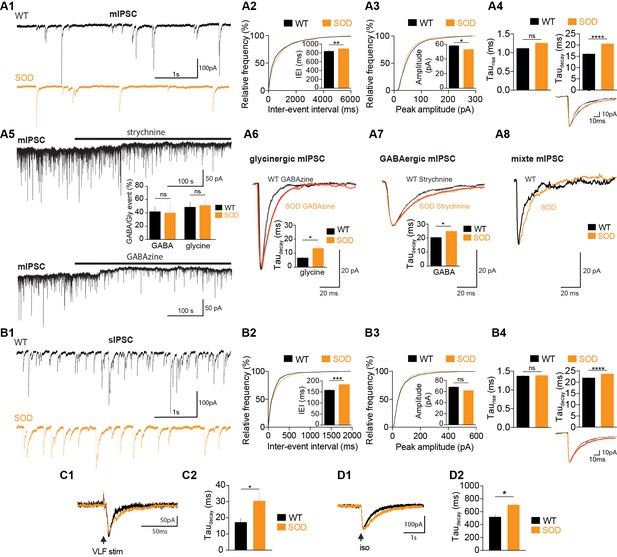
SOD E17.5 MNs exhibit a reduced IPSC frequency compared with WT MNs along with a persistent increase in their taudecay.
(A1) Sample miniature IPSC (mIPSC) recordings obtained from representative SOD and WT MNs (holding membrane potential −70 mV). (A2) Cumulative frequency of inter-event interval (IEI) and mean values of mIPSCS revealed a small but significant reduction in frequency in SOD MNs (n = 19) compared with WT (n = 15) MNs. (A3) mIPSC peak amplitude was slightly smaller in SOD than in WT MNs (same layout as in A2). (A4) Mean taurise was unchanged but mean taudecay was significantly increased in SOD mIPSCs compared with WT mIPSCs. Inset traces (mean of 30 events) from representative experiments. (A5) Blocking the GlyR or GABAAR (black horizontal bars) reveals a tonic current. The distribution of pure GABAAR-mediated mIPSCs in WT (n = 5) and SOD (n = 3) MNs and pure GlyR-mediated mIPSCs in WT (n = 3) and SOD (n = 5) MNs is shown between the two traces. (A6) Taudecay of pure GlyR-mediated mIPSCs is significantly increased in SOD MNs (n = 3) compared to WT MNs (n = 5). Traces are mean of 53 events (WT) and 57 events (SOD) from representative experiments. (A7) Relaxation of pure GABAAR-mediated mIPSCs is significantly longer in SOD MNs (n = 3) compared to WT MNs (n = 5). Traces are mean of 35 events (WT) and 35 events (SOD) from representative experiments. (A8) Mixed GlyR-GABAAR-mediated events from representative experiments. (B1) Samples of spontaneous IPSC (sIPSC) recordings from representative SOD and WT MNs. (B2) Cumulative frequency of IEI and mean values of sIPSCS revealed a slight frequency reduction in SOD MNs (n = 17) compared to WT MNs (n = 14). (B3) sIPSC peak amplitudes were similar in SOD and WT MNs. (B4) Mean taurise was not significantly different in WT sIPSCs, but SOD sIPSCs exhibited a higher taudecay. Inset traces (mean of 30 events) from representative experiments. (C1–C2) SOD eIPSCs (n = 6) displayed a higher taudecay than WT eIPSCs (n = 10). (D1–D2) Puff-evoked SOD GABAAR currents (n = 21) exhibited a higher taudecay than WT GABAAR currents (n = 16). Left traces in C1) and D1) are from representative SOD and WT eIPSCs and puff-evoked GABAAR currents. ****p<0.0001, ***p<0.001, **p<0.01, *p<0.05, ns non-significant, Mann Whitney test.
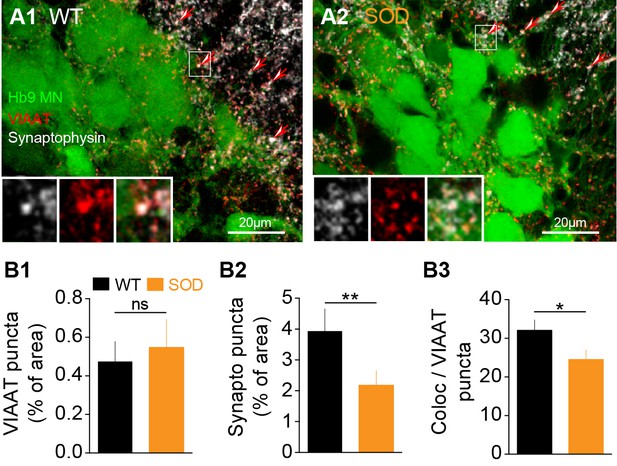
Inhibitory terminal staining in E17.5 SOD and WT SCs.
(A1-A2) Confocal visualization of Hb9-eGFP MNs (green) along with VIAAT (red) and synaptophysin (white) staining in lumbar E17.5 WT (A1) and SOD1 SCs (A2) (frontal sections). Arrows point the synaptophysin-VIAAT co-localized staining highlighted in the left corner insets (0.2 µm thickness optical section). (B1) The density of VIAAT puncta was similar in WT (n = 6) and SOD1 (n = 5) SCs. (B2) SOD SCs again exhibited a significant reduction in their density of synaptophysin terminals. (B3) VIAAT puncta co-localizing with synaptophysin puncta were slightly reduced in SOD SCs compared with WT SCs. ***p<0.001, **p<0.01, *p<0.05, ns non-significant, Mann Whitney test.
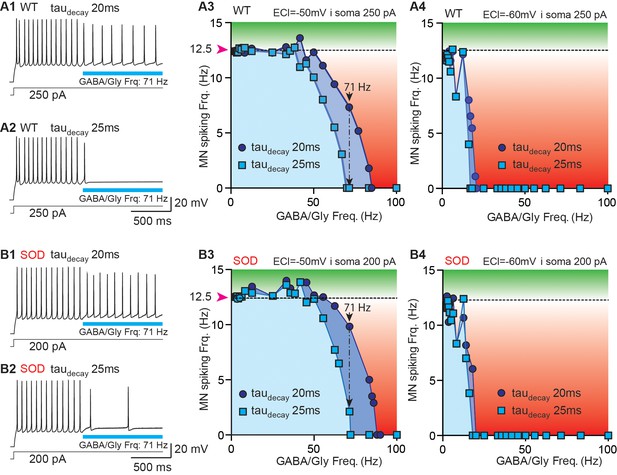
Simulation of a putative compensatory role of taudecay for the inhibitory strength of GABA/gly synaptic events in SOD-like MNs.
(A) Simulations made with a WT-like MN whose soma was continuously injected with depolarizing current (250 pA) to induce spiking discharge at a rate of ~12.5 Hz. After the spiking discharge was stabilized (t = 2 s), a train of GABA/Gly synaptic events was delivered to the soma at a frequency of 71 Hz, with either a taudecay of 20 ms (A1) or 25 ms (A2) and EGABAAR set at −50 mV. Using these two values of GABA/Gly taudecay (20 ms: dark blue circles; 25 ms: light blue squares), various frequencies of GABA/Gly synaptic train (from 0 to 100 Hz) were tested with either EGABAAR = -50 mV (A3) or EGABAAR = −60 mV (A4). The horizontal dashed line represents the stabilized spiking frequency before application of the GABA/Gly synaptic train. Inhibitory effects (below dashed line) are in red, excitatory effects (above this line) are in green. (B1–B5) Simulations made with a SOD-like MN, in which the soma was continuously depolarized (200 pA injection) to induce spiking discharge at ~12.5 Hz (same layout as in A). Note that in the SOD-like MN with EGABAAR = −50 mV, excitatory effects are observed when the GABA/Gly synaptic frequency is below 50 Hz (B3). This effect is not observed when EGABAAR = −60 mV (B4) or is almost absent in the WT-like MN with EGABAAR = −50 mV.
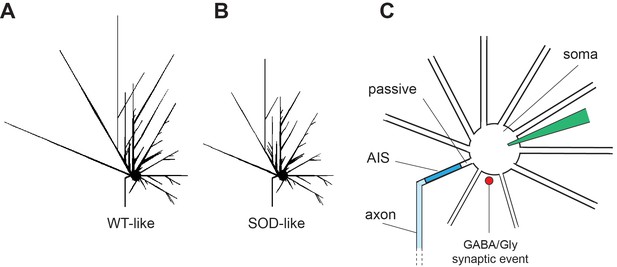
Simulated E17.5 WT-like and E17.5 SOD-like MNs.
(A, B) The two simulated MNs differ only in the length of their terminal dendrites, being shorter in the SOD-like MN. (C) Schematic drawing of the simulated cell body, dendrites and axon along with the location of GABA/Gly synaptic input. AIS: axon initial segment.
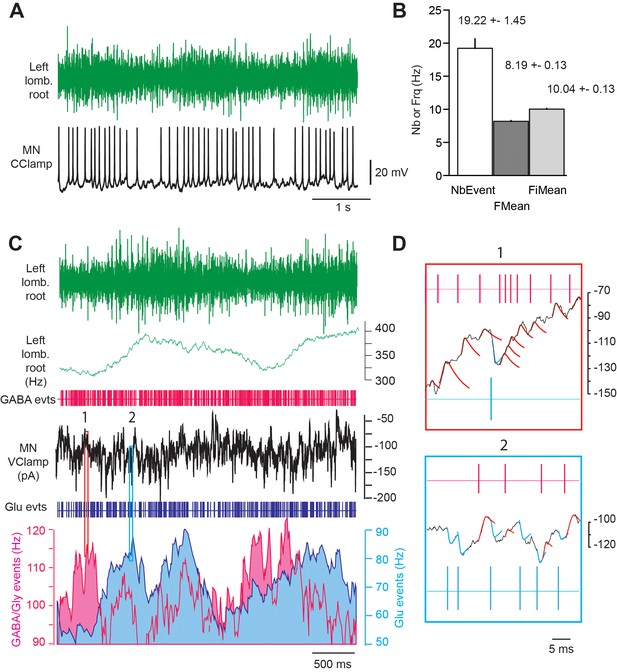
Firing activity and synaptic inputs recorded from E17.5 MNs during fictive locomotion.
(A) Activity recorded simultaneously in a lumbar ventral root (L5) and a spinal MN (L5 level). (B) Quantification of the ventral root activity during a locomotor-related burst, including number of events (spikes), mean spike frequency (FMean) and mean instantaneous frequency (FiMean). (C) Analysis of the instantaneous frequency of excitatory (Glu, blue) and inhibitory (GABA/Gly, pink) synaptic events recorded in voltage-clamp mode (holding membrane potential −45 mV) from an E17.5 lumbar MN during a locomotor-like sequence. Note that GABA/Gly synaptic events may recur at a frequency of >100 Hz during locomotor bursts. (D) Detection of a barrage of inhibitory (upward projecting) synaptic events (upper panel, corresponding to position one in C) and mixed inhibitory (upward) and excitatory (downward) synaptic events (lower panel, position two in C).
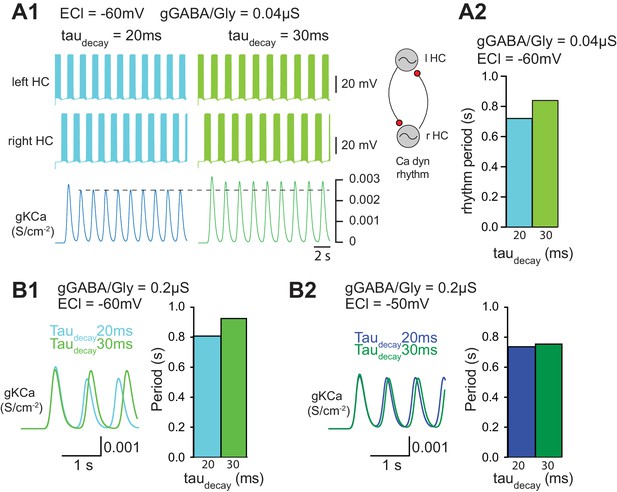
Functional consequence of increasing taudecay on locomotor activity.
(A) Effect of taudecay on the locomotor rhythm when EGABAAR (ECl) is set to −60 mV (A1-A2, B1) and −50 mV (B2).
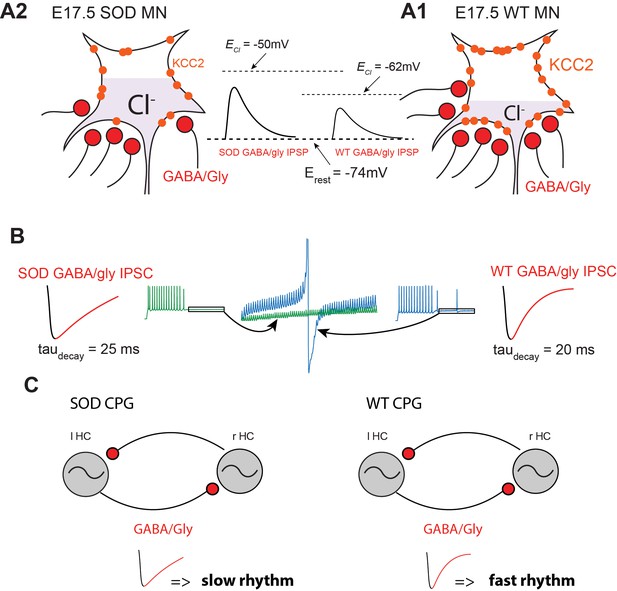
Summary.
(A) Schematic drawing summarizing the altered inhibitory inputs (~25% reduction, see Figure 3B3) to fetal SOD MNs. [Cl-]i is higher in SOD MNs (A1) than in WT MNs (A2) because of a KCC2 down-regulation, leading to an increased GABA/Gly-induced depolarizing effect (see insets). (B) Consequence of increasing taudecay on the GABA/gly inhibitory effect in SOD-like MNs. Due to an accumulation in the intracellular compartment, EGABAAR exerts a strong depolarizing effect. A burst of spikes generated by MNs is hardly blocked by a barrage of GABA/gly events (see blue traces) when taudecay is set to 20 ms. Increasing taudecay to 25 ms allows a better summation of the shunting component of the depolarizing GABA/gly post-synaptic event leading to a better clamp of Em towards EGABAAR and to the blockade of MN discharge (see green traces). (C) Impact of a larger taudecay in SOD MNs on the frequency of the locomotor rhythm.
Tables
Passive membrane properties of E17.5 MNs and GABAAR conductances.
Data are from perforated patch-clamp recordings and are shown as mean ± SEM. Statistical significance was calculated by a non-parametric Mann Whitney test. ns, p>0.05; *p<0.05.
Rin (MΩ) | Cm (pF) | gGABAAR (pS) | gGABAAR/Cm (MΩ/pS) | N | N | |
---|---|---|---|---|---|---|
WT | 104.9 ± 10.8 | 143.7 ± 14.2 | 3.90 ± 0.97 | 0.029 ± 0.007 | 16 | 10 |
SOD | 148.2 ± 13.5* | 105.6 ± 5.9* | 1.91 ± 0.24ns | 0.019 ± 0.003ns | 21 | 16 |
Reagent type (species) or resource | Designation | Source or reference | Identifiers | Additional information |
---|---|---|---|---|
Strain (Mus musculus) | B6SJL-Tg(SOD1*G93A)1Gur/J | The Jackson Laboratory | RRID:IMSR_JAX:002726 | |
Antibody | anti-KCC2 rabbit polyclonal | Millipore | Cat#07–432 RRID:AB_11213615 | 1:1000 (WB) 1:400 (IHC) |
Antibody | anti-NKCC1 mouse monoclonal | Developmental Studies Hybridoma Bank | T4 | 1:400 (WB) |
Antibody | anti-VIAAT rabbit polyclonal | Provided by B. Gasnier, Paris Descartes University | VIAAT | 1:1000 (IHC) |
Antibody | anti-synaptophysin mouse monoclonal | Millipore Sigma | Clone SVP38 RRID:AB_2315393 | 1:500 (IHC) |
Antibody | anti-FoxP1 rabbit polyclonal | Millipore Sigma | Cat#AB2277 RRID:AB_10631732 | 1:500 (IHC) |
Antibody | goat anti-rabbit secondary antibody Alexa Fluor 546 | Invitrogen | Cat#A-11035 | 1:500 (IHC) |
Antibody | goat anti-mouse secondary antibody Alexa Fluor 647 | Invitrogen | Cat#A-21235 RRID:AB_2535804 | 1:500 (IHC) |
Antibody | Goat anti-Rabbit IgG-heavy and light chain cross-adsorbed Antibody HRP Conjugated | Euromedex | Cat#A120-201P | 1:20000 (WB) |
Antibody | Immun-Star GAM-HRP Conjugate | Bio-Rad | Cat#1705047 | 1:20000 (WB) |
Commercial assay, kit | Qproteome Mammalian Protein Prep Kit | Qiagen | Cat#37901 | |
Chemical compound, drug | Phosphatase Inhibitor Cocktail 2 | Millipore Sigma | Cat#P5726 | |
Commercial assay, kit | DC protein assay | Bio-Rad | Cat#5000113 Cat#5000114 Cat#5000115 | |
Commercial assay, kit | Mini-PROTEAN TGX Stain-Free Gels | Bio-Rad | Cat#4568034 | |
Chemical compound, drug | 10x Tris/Glycine/SDS | Bio-Rad | Cat#1610732 | |
Commercial assay, kit | Precision Plus Protein All Blue Standards | Bio-Rad | Cat#1610373 | |
Commercial assay, kit | Trans-Blot Turbo Transfer Pack, nitrocellulose | Bio-Rad | Cat#1704158 | |
Chemical compound, drug | Clarity Western ECL, Substrate | Bio-Rad | Cat#1705060 | |
Chemical compound, drug | Tetrodotoxin (citrate free) | Latoxan Laboratory, France | Cat#L8503 | 0.2 µM |
Chemical compound, drug | Kynurenic acid | Millipore Sigma | Cat#K3375 | 4 mM |
Chemical compound, drug | Tubocurarine hydrochloride pentahydrate | Millipore Sigma | Cat#2379 | 10 µM |
Chemical compound, drug | Dihydro-β-erythroidine hydrobromide | Bio-techne, France | Cat#2349/10 | 5 µM |
Chemical compound, drug | Strychnine Hemisulfate salt | Millipore Sigma | Cat#S7001 | 3 µM |
Chemical compound, drug | (2R)-amino-5-phosphonovaleric acid (DL-AP5) | Bio-techne, France | Cat#0105/10 | 40 µM |
Chemical compound, drug | 6-cyano-7-nitroquinoxaline-2,3-dione (CNQX) | Bio-techne, France | Cat#0190/10 | 20 µM |
Chemical compound, drug | SR 95531 hydrobromide (GABAzine) | Bio-techne, France | Cat#1262/10 | 3 µM |
Chemical compound, drug | bumetanide | Millipore Sigma | Cat#B3023 | 10 µM |
Chemical compound, drug | VU0240551 | Bio-techne, France | Cat#3888/10 | 10 µM |
Chemical compound, drug | Isoguvacine hydrochloride | Bio-techne, France | Cat#0235/100 | 50 µM |
Chemical compound, drug | Dopamine hydrochloride | Millipore Sigma | Cat#H8502 | 100 µM |
Chemical compound, drug | Serotonin hydrochloride (5-HT) | Millipore Sigma | Cat#H9523 | 10 µM |
Chemical compound, drug | N-Methyl-D-aspartic acid (NMDA) | Millipore Sigma | Cat#M3262 | 10 µM |
Chemical compound, drug | Gramicidin from Bacillus aneurinolyticus (Bacillus brevis) | Millipore Sigma | Cat#G5002 | 10–20 μgml−1 |
Software, algorithm | NEURON 7.3 | NEURON | RRID:SCR_005393 |