Measuring ligand-cell surface receptor affinities with axial line-scanning fluorescence correlation spectroscopy
Figures
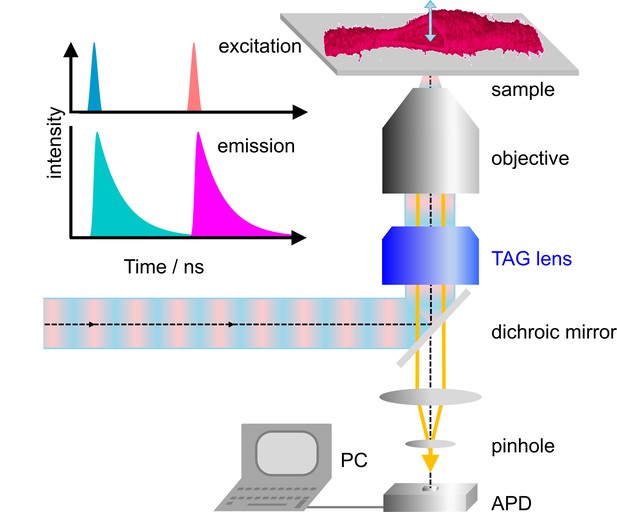
Dual-color confocal microscopy with pulsed interleaved excitation and ultrafast axial scanning with a tunable acoustic gradient index of refraction (TAG) lens.
Shown are a schematic depiction of the microscope and (upper left) the excitation pulse sequence and the ensuing fluorescence emission. The sample cell (shown as a 3D image) is cut open to visualize axial scanning across the top membrane. The 3D image has been merged from four image slices (80 × 80 µm2, 256 × 256 pixels, three scans, each with pixel dwell time 60 µs). APD, avalanche photodiode.
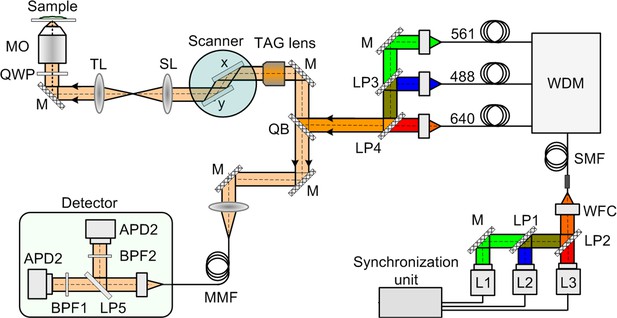
Schematic of the confocal microscope with fast axial scanning.
APD1 and APD2, avalanche photodiodes (τ-SPAD Single Photon Counting Module, PicoQuant, Berlin, Germany); BPF1 and BPF2, bandpass filters. For laser excitation at 470 nm: Brightline HC 525/50 or HC 520/35 with mCherry or tdTomato as receptor markers, respectively, for 561 nm: HC 600/37, for 640 nm: HC 676/37 (all Semrock, Rochester, NY); L1 – L3, picosecond pulsed lasers (L1: 561 nm (PDL 561, Abberior, Göttingen, Germany), L2: 470 nm (LDH-P-C-470B, Picoquant), L3: 640 nm (LDH-P-C-640B; PicoQuant)); LP1 – LP5, longpass dichroic mirrors (LP1: 532 nm longpass (AHF, Tübingen, Germany), LP2: 605 nm longpass (Thorlabs, Munich, Germany), LP3: 532 nm longpass (AHF), LP4: 575 nm longpass (Edmund Optics, Mainz, Germany), LP5: 555 nm longpass (FF555-Di02, Semrock); M, dichroic mirror; MMF, multimode fiber ((MMF-IRVIS-62.5/125–0.245 L, OZ Optics, Ottawa, Canada); MO, microscope objective (HCX PL APO W CORR CS 63x/1.2, Leica Microsystems, Wetzlar, Germany); Scanner, galvanometric laser scanner (Yanus V, Till Photonics, Gräfelfing, Germany); SMF, single mode fiber; SL, scan lens (AC254-040-A-ML, Thorlabs); TL, tube lens; TAG lens, tunable acoustic gradient index of refraction lens (model 2.0, TAG Optics, Princeton, NJ); QB, quad-band dichroic mirror (zt 405/473/561/640 RPC, AHF); QWP, quarter-wave plate (AQWP05M-600, Thorlabs); SMF, single-mode fiber; WDM, wavelength division multiplexer (RGB26HA, Thorlabs); WFC, wideband fiber coupler (TW630R5A1, Thorlabs).
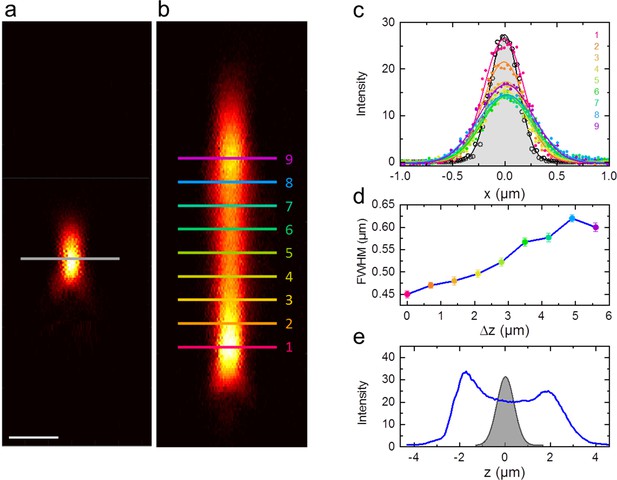
Characterization of the confocal spot upon TAG lens scanning.
Shown are x-z sections of the image of an 80 nm gold bead (EM.GC80, BBI solutions, Cardiff, UK) immobilized in an agarose hydrogel (3%, w/w) 30 µm above the cover glass, taken with 640 nm laser irradiation (a) without and (b) with the TAG lens oscillating in resonance. The scanned volume was 3 × 3 × 12 µm3. Scale bar, 1 µm. (c) Intensity profiles along lines 1–9 in panel b, which cross the focus laterally at different axial positions. The intensity profile without oscillating TAG lens is included for comparison (shaded in grey). (d) Full widths at half maximum (FWHM) of the lateral intensity distribution as a function of the axial position. The FWHM averaged over all axial positions is 0.53 ± 0.05 µm (mean ± SD). With the TAG lens switched off, the FWHM of the focus is 0.32 ± 0.01 µm laterally and 0.89 ± 0.02 µm axially. (e) Intensity within the x-y plane integrated within a circle of radius 0.25 µm around the center at various axial positions. Black curve: TAG lens turned off, blue curve: TAG lens turned on.
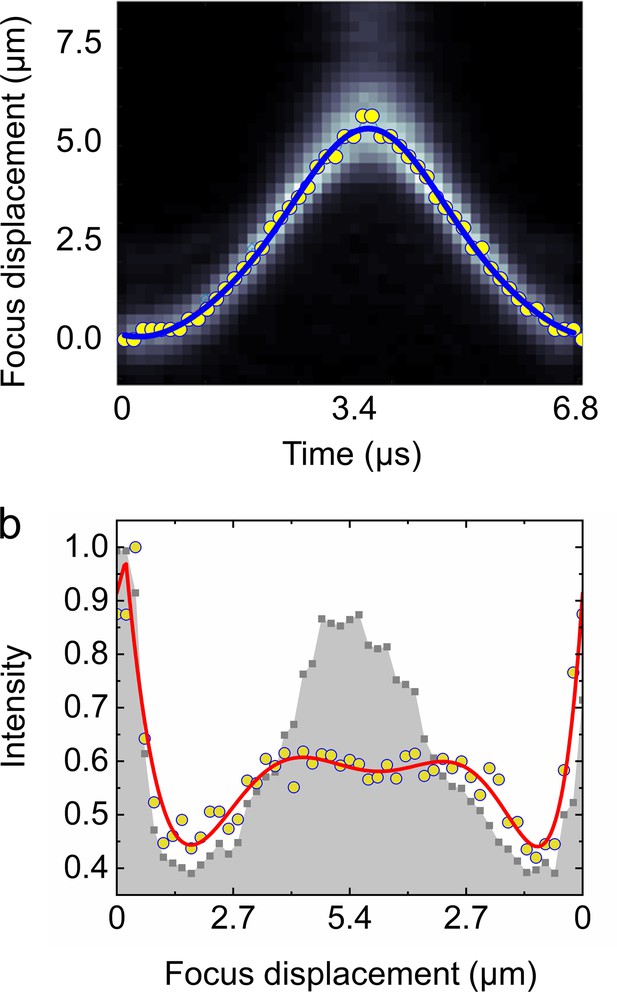
Compensation of the nonlinear axial scanning by the TAG lens.
(a) Nonlinear axial scan performed by the TAG lens resonantly driven at 147 kHz. The curve was acquired by scanning a cover glass at 300 equally spaced axial positions (displacement 50 nm each) by using the piezo transducer of the microscope (total scan range 15 µm, 8 µm selected for the analysis, 0.12 s/position), with the TAG lens turned on. The photons reflected from the bottom surface of the cover glass were registered by the TCSPC card. The data were rearranged into an image to establish a relation between the time elapsed since the preceding TAG lens timing pulse, t, and the axial position, z. The data points (shifted along the y-axis to represent the focus displacement with respect to the cover glass) are shown as yellow spheres, the blue line is a fit with a Fourier sum,, yielding z0 = 2.41 µm, z1 = –2.58 µm, z2 = 0.33 µm, z3 = –0.08 µm; = 1.25 rad, = 0.90 rad, = 0.56 rad, T = 6.8 µs. (b) The fit function was then used to reassign all registered photons to specific axial positions of the TAG lens, covering a complete TAG lens scanning period. (b) Symbols: Intensities of the brightest pixel in each column for 50 TAG lens positions equally spaced along the axial direction, normalized to a scale between 0 and 1. Red line: fit with a polynomial, , with a0 = 1.27, a1 = ‒12.53, a2 = 63.53, a3 = ‒87.72, a4 = ‒163.38, a5 = 616.21, a6 = ‒649.88, a7 = 233.49. We took the brightest pixel for correcting the intensities and not the integrated intensity shown in grey because, in the axial lsFCS data analysis, the membrane positions, zMEM1(t) and zMEM2(t), are also determined from the pixels with the highest photon counts.
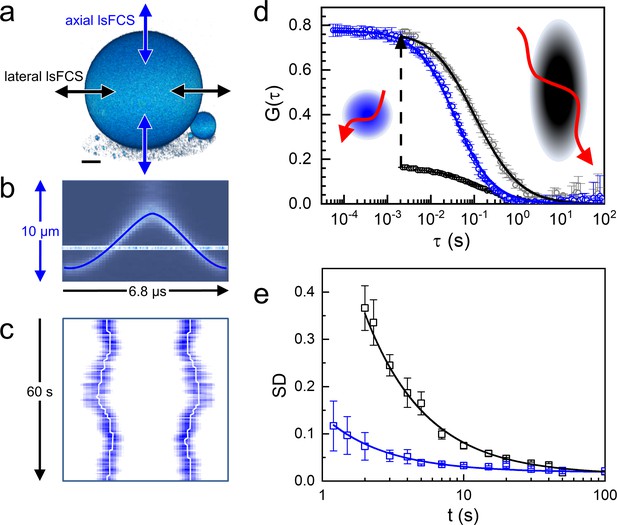
Axial and lateral line-scanning FCS on giant unilamellar vesicles (GUVs) labeled with lipid-bound Atto647N.
(a) 3D image of a GUV (diameter 60 µm), showing the scanning directions of the focus across the membrane in lateral and axial lsFCS; scale bar, 10 µm. (b) Axial focus position during a single oscillation cycle of 6.8 µs. (c) Kymograph, horizontal axis: intensity during axial focus oscillations (averaged over 500 ms), vertical axis: sequence of 500 ms periods during 60 s of axial scanning. Lines mark the membrane positions. (d) Autocorrelation curves for axial (blue) and lateral (black) scanning; points: experimental data; lines: fits with Equations (1) and (2), yielding τD = 36 ± 1 ms with axial scanning and τD = 46 ± 2 ms, S = 4.0 ± 0.3 with lateral scanning, respectively. The lateral lsFCS autocorrelation data have also been scaled to the axial ones (grey) for better comparison. Observation areas on the plasma membrane are depicted schematically in the corresponding colors, possible dye trajectories are shown in red. (e) Standard deviation (SD), calculated for two groups of seven normalized lateral (black) and axial (blue) lsFCS curves, plotted as a function of the overall time of data acquisition. For details, see Materials and methods.
-
Figure 2—source data 1
Numerical data of the plots in Figure 2d,e.
- https://cdn.elifesciences.org/articles/55286/elife-55286-fig2-data1-v2.xlsx
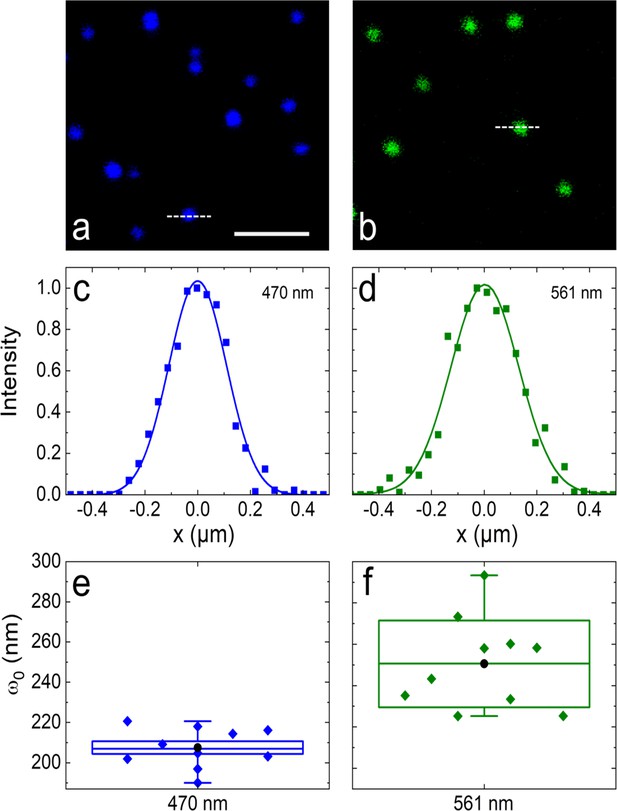
Focus size determination by imaging multi-color fluorescent beads.
Confocal images of multi-color spheres excited at (a) 470 nm and (b) 561 nm. (c, d) Symbols: intensity distribution along the dashed lines in panels a and b, respectively. Lines: Gaussian fits, yielding FWHM values of 258 ± 8 nm and 300 ± 10 nm for 470 and 561 nm excitation. Confocal width parameter, ω0 (corresponding to a 1/e2 intensity drop), measured on ten multi-color beads with excitation at (e) 470 nm and (f) 561 nm. Boxes mark the SE. The median is indicated by the central line, the mean as a black circle. Whiskers mark the full range.
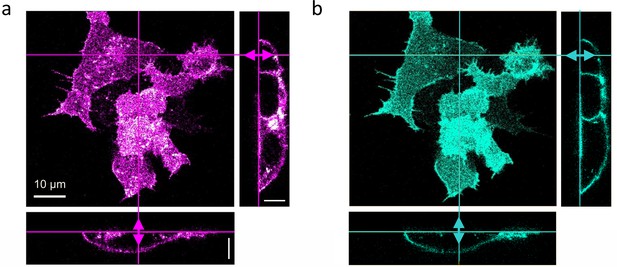
Dual-color confocal image stacks of live HEK293T cells, transiently transfected to express LRP6-mCherry (red-fluorescent) and incubated with CM containing 1 nM DKK1-eGFP (green-fluorescent) for 30 min.
Confocal images of cells were selected from image stacks taken sequentially (to avoid crosstalk) with 561 (3 µW) and 470 nm (5 µW) laser excitation to visualize (a) LRP6-mCherry and (b) DKK1-eGFP. A volume of 65 × 65 × 25 µm3 was scanned with 256 × 256 × 64 pixels (pixel dwell time, 40 µs). Shown are a lateral slice near the glass coverslip and cross-sections along the lines marked in the lateral view. Scale bars, 10 µm.
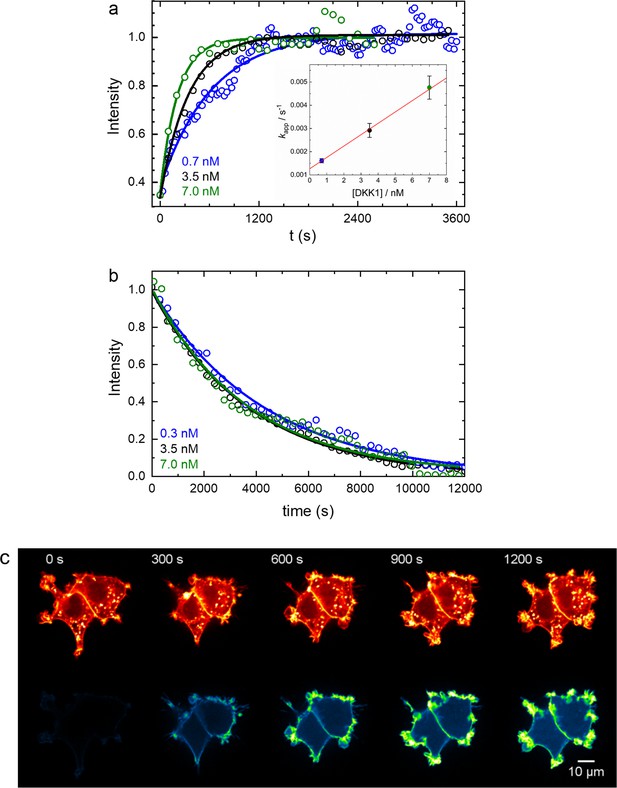
Kinetics of receptor-ligand association and dissociation measured on live cells kept at 37°C and 5% CO2.
(a) Time dependence of the DKK1-eGFP emission from the cell membrane of transfected HEK293T cells expressing LRP6-mCherry after adding conditioned medium (CM) containing the ligand at different concentrations. Time-lapse spinning disk confocal images (200 ms frames every 100 s) were recorded for 1 hr, λexc = 488 nm, λdet = 525 ± 25 nm. Symbols, data; lines, exponential fits. Inset: concentration dependence of the apparent association rate coefficients obtained by exponential fits of the kinetic traces. Linear regression yields kon = (5.0 ± 0.2)×10‒4 nM‒1 s‒1, koff = (1.3 ± 0.1)×10‒3 s‒1, resulting in Kd = 2.6 ± 0.1 nM. We note that such measurements on individual cells are highly unreliable due to large systematic errors. (b) DKK1-eGFP emission decrease after removing unbound DKK1-eGFP ligands by two successive washing steps. 200 ms frames were recorded every 300 s for up to 3 hr. Symbols, data; lines, exponential fits. For an ensemble of 50 cells, koff = (2.6 ± 0.2)×10‒4 s‒1 was obtained as an average. Fixing this koff intercept in a linear regression of two apparent rate coefficients (at 3.5 and 7 nM, averages over 50 cells) yielded kon = (6.7 ± 0.2)×10‒4 nM‒1 s‒1 and Kd = 0.4 ± 0.1 nM. We note that the Kd value obtained by these extremely tedious experiments agrees within the error with the one measured by axial lsFCS (Table 1). (c) Confocal images of LRP6-mCherry (top) before (0 s) and after (300, 600, 900 and 1200 s) adding CM with 5.1 nM DKK1-eGFP (bottom).
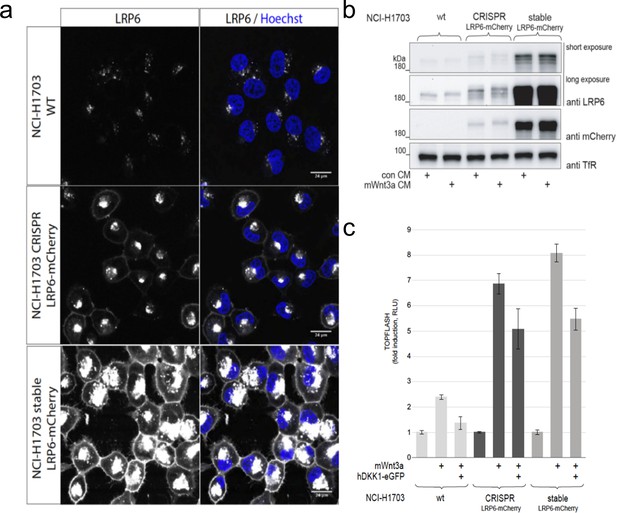
Expression and activity analysis of endogenously-tagged LRP6-mCherry in CRISPR/Cas9 edited NCI-H1703 cells and exogenous LRP6-mCherry in NCI-H1703 cells with stable gene insertion.
(a) Confocal laser scanning microscopy images showing cell surface localization and relative expression levels of LRP6-mCherry in live cells. Non-specific autofluorescence is seen only adjacent to the nucleus (top panel with wt cells). (b) SDS-PAGE/western blot analysis showing relative expression levels of LRP6 receptor proteins in lysates from CRISPR/Cas9 and stable cell lines, compared to endogenous LRP6 from wt NCI-H1703 cells. Prior to harvest of cell lysates, cells were treated overnight with either control (con) CM or mWnt3a CM as indicated. Note the expected size increase of the LRP6 fusion protein upon tagging as well as the characteristic upshift of the LRP6 receptor protein upon Wnt stimulation, confirming Wnt-dependent receptor modification. The latter effect is less obvious in the stably expressing LRP6-mCherry cell line. Transferrin receptor (TfR) was used as a protein loading control. Transiently transfected cells were not included on the western blot as NCI-H1703 cell transfection efficiency is too low to allow comparison of average expression levels and instead requires analysis of single cells by microscopy. (c) TOPFLASH Wnt/β-catenin reporter assay showing the relative responsiveness of the LRP6-mCherry cell lines to both Wnt stimulation and DKK1-eGFP inhibition. Cells in 96-well plates were transfected with 20/5 ng of TOPFLASH/Renilla reporters, 8 ng mouse Wnt3a and 25 ng human DKK1-eGFP as indicated. 48 hr post transfection, cell lysates were harvested for luciferase assays. Note that both the CRISPR/Cas9 edited cell line and the cells stably expressing LRP6-mCherry respond to Wnt3a and DKK1 in the expected manner.
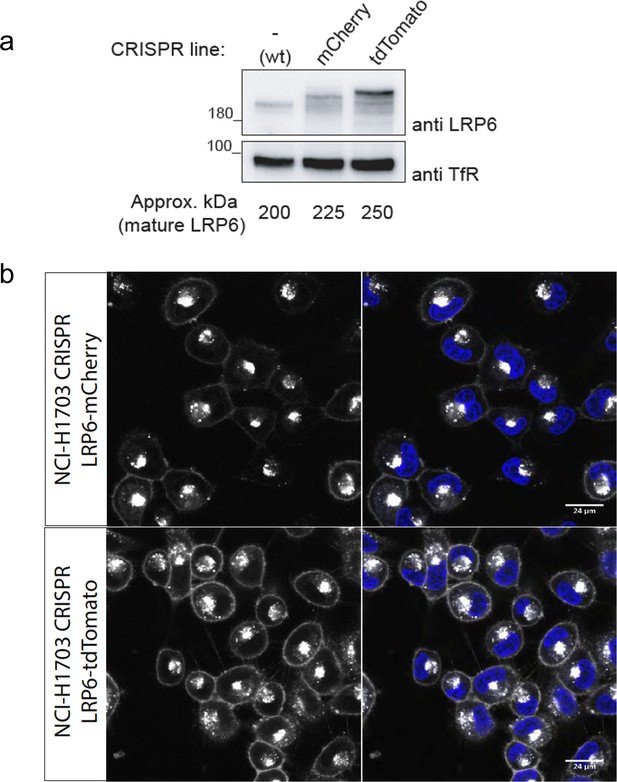
Comparison of endogenously tagged LRP6-mCherry and LRP6-tdTomato expressed in CRISPR/Cas9 edited NCI-H1703 cells.
(a) SDS-PAGE/Western blot analysis showing relative expression levels of LRP6-mCherry and LRP6-tdTomato from lysates of the corresponding CRISPR/Cas9 cell lines. Note the presence of lower molecular weight wt LRP6 bands in both endogenously-tagged cell lines, in line with heterozygous tagging of the endogenous LRP6 locus in NCI-H1703 cells. Note also the higher apparent expression of the endogenously tagged LRP6 allele compared to the unedited allele in lysates from both CRISPR/Cas9 cell lines. (b) Confocal laser scanning microscopy images of CRISPR/Cas9 edited cells showing a direct comparison of cell surface localization and fluorescence intensity of the two endogenously tagged LRP6 receptor proteins.
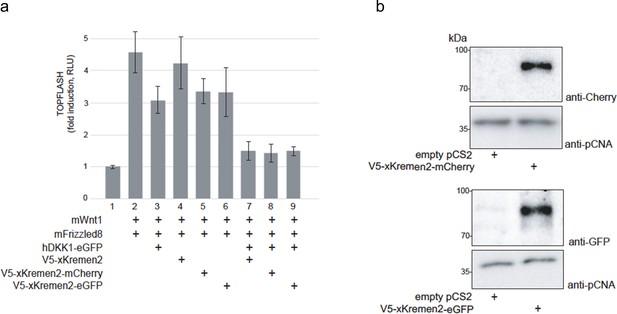
Activity tests and western blot analysis of xKremen2-mCherry and xKremen2-eGFP.
(a) TOPFLASH Wnt/β-catenin reporter assay. HEK293T cells in 96-wells were transfected with 25/5 ng TOPFLASH/Renilla reporters, 8 ng mWnt1, 1 ng mFrizzled8, 1 ng hDKK1-eGFP, 1 ng V5-xKremen2, 1 ng V5-xKremen2-mCherry and 1 ng V5-xKremen2-eGFP as indicated. 24 hr post transfection, cell lysates were harvested for luciferase reporter assay. Note that, in the absence of DKK1-eGFP, the fluorescently tagged Kremen2 receptors show marginally greater inhibition of Wnt signaling compared to non-fluorescently tagged Kremen2 (compare lanes 4, 5 and 6). In the presence of DKK1, however, all Kremen2 constructs show a strong reduction (compare lane 3 with lanes 7, 8 and 9), confirming that they retain their ability to synergize with DKK1 in Wnt inhibition. (b) Western blot analysis to visualize the integrity of both V5-xKremen2-mCherry and V5-xKremen2-eGFP.
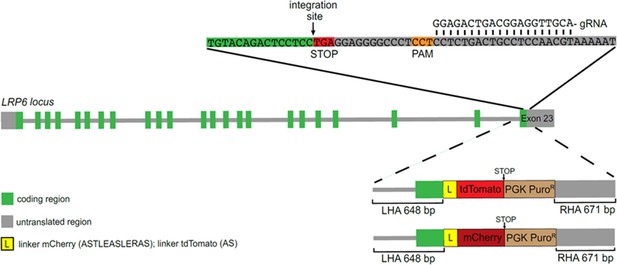
CRISPR/Cas9 editing of LRP6.
Schematic depiction of the LRP6 tagged locus at exon 23. The gene sequence of LRP6, the sequence and position of the guide RNA (gRNA) and the PAM site as well as the integration site are shown. The donor DNA includes the left and right homology arms (LHA, RHA) of indicated length, the FP gene and the resistance cassette.
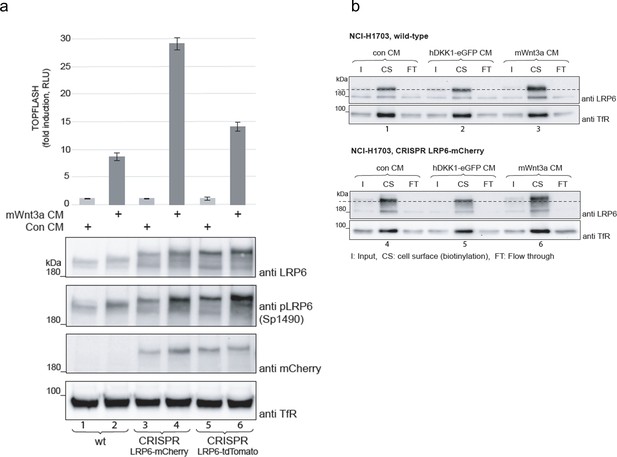
Characterization of CRISPR/Cas9 edited NCI-H1703 cell lines harboring endogenous, fluorescently tagged LRP6.
(a) Wnt/β-catenin signaling response of different cell lines and their corresponding LRP6 receptor proteins to Wnt3a ligand stimulation. Upper graph: TOPFLASH Wnt reporter assay under both control and Wnt3a stimulated conditions. Wt and CRISPR/Cas9 edited NCI-H1703 cells harboring LRP6 tagged with either mCherry or tdTomato were transfected with TOPFLASH reporter and treated overnight with either control CM (con CM) or Wnt3a CM as indicated. Lower panels: Western blots showing either wt LRP6, LRP6-mCherry or LRP6-tdTomato proteins expressed by the different cell lines. Note the expected size increase of the LRP6 fusion protein upon endogenous tagging with either mCherry (25 kDa, compare lanes 1 and 3) or tdTomato (50 kDa, compare lanes 1 and 5). The fainter, lower molecular weight LRP6 bands visible in CRISPR/Cas9 edited cells correspond to wt LRP6 expressed from the unedited LRP6 allele of the heterozygous edited cells, which are not visible in the anti-mCherry Western blot. Note also the Wnt-induced upshifts of bands for wt LRP6 (compare lanes 1 and 2) as well as the LRP6-mCherry (compare lanes 3 and 4) and LRP6-tdTomato (compare lanes 5 and 6) fusion proteins, confirming that correct covalent modification of LRP6 occurs upon Wnt pathway stimulation. Wnt-dependent activation of endogenously fluorescently tagged LRP6 was further confirmed by analysis of LRP6 phosphorylation, using a phospho-specific LRP6 antibody (Sp1490). As can be seen in the pLRP6 western blot panel, an increase in phosphorylation levels accompanies the upshifted LRP6 bands (compare lane 2 with 1, 4 with 3 and 6 with 5). Total LRP6 protein levels were assessed using LRP6 specific antibodies, a mCherry antibody was used to specifically detect LRP6 fused to either mCherry or tdTomato and an antibody specific for the TfR was used as a loading control. (b) Cell surface biotinylation assay and western blot analysis to detect LRP6 located specifically on the plasma membrane and the effects of Wnt and DKK1 CM on membrane localization. Wt (upper panels) as well as CRISPR/Cas9 edited NCI-H1703 cells harboring tagged LRP6-mCherry (lower panels) were treated with con CM, DKK1-eGFP CM or mWnt3a CM for 2 hr. Cell surface proteins were biotinylated and purified by affinity pull-down using NeutrAvidin-agarose beads, LRP6 was specifically detected by immunoblotting. Note that slightly greater amounts of cell surface LRP6-mCherry were obtained from CRISPR/Cas9 edited cells compared to wt cells (compare lanes 1 and 4), in line with our observations that endogenously tagged LRP6 is expressed at somewhat higher levels. Note also the Wnt-dependent upshift of cell surface localized LRP6 (compare lanes 1 and 3) and LRP6-mCherry (compare lanes 4 and 6) from wt and CRISPR edited cells, respectively. Incubation with DKK1-eGFP resulted in a small reduction of cell surface LRP6 levels as well as LRP6 size. I: input; CS: cell surface (biotinylated proteins); FT: flow through. The TfR was used as a loading control. The sample loading ratio for I:CS:FT was 2:20:1.
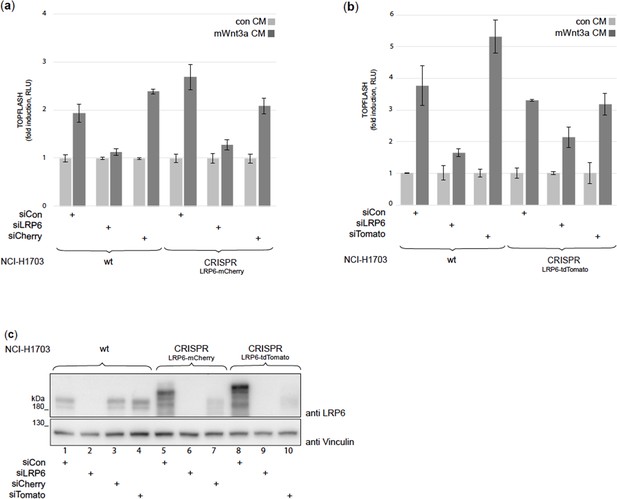
siRNA-mediated knockdown to characterize CRISPR/Cas9 edited NCI-H1703 cells.
(a, b) TOPFLASH Wnt reporter assays showing the effect of siRNA-mediated silencing of all LRP6 receptors (siLRP6) or only the endogenously tagged LRP6 receptors (siCherry/siTomato). CRISPR/Cas9 edited NCI-H1703 cells expressing endogenously-tagged LRP6-mCherry (a) and LRP6-tdTomato (b) as well as wt cells (a and b) were co-transfected in 96-well format with 3.3 pmol siRNA (targeting either LRP6, mCherry or tdTomato) and 25/5 ng TOPFLASH/Renilla reporters. 18 hr post-transfection, cells were treated overnight with control or Wnt3a CM before harvesting cell lysates for luciferase assays. For each treatment, luciferase values were set to one for the control samples in order to compare Wnt responsiveness between cell lines. As expected, knockdown of all LRP6 receptors with siLRP6 resulted in a strong reduction of the Wnt-induced TOPFLASH signal, although the LRP6-tdTomato CRISPR cell line displayed a somewhat weaker reduction. For specific knockdown of the endogenously tagged LRP6-mCherry species using siCherry, the degree of Wnt activation was significantly reduced when compared to the effect of siCherry on the corresponding wt cells. This partial reduction confirms that LRP6-mCherry contributes to Wnt signaling activity seen in these cells but that siRNA resistant, non-tagged LRP6 remains. Similarly, for LRP6-tdTomato silencing using siTomato, a significantly reduced Wnt activation was observed when compared to the effect of siTomato on wt cells. These results confirm that LRP6-mCherry and LRP6-tdTomato are active in Wnt reception, and, in agreement with our sequencing results of the CRISPR/Cas9 cell lines, that both cell lines are heterozygous, such that only one of the LRP6 alleles has been endogenously tagged. (c) Western blot analysis of LRP6, LRP6-mCherry and LRP6-tdTomato from wt and CRISPR/Cas9 edited NCI-H1703 cell lines after the indicated siRNA transfections. Cells were transfected with 39.6 pmol siRNA in 24-well format and cell lysates were harvested after 48 hr for Western blot analysis. Note that knockdown using siLRP6 leads to complete loss of all LRP6 receptor proteins (lanes 2, 6 and 9), whereas knockdown using siCherry (lane 7) or LRP6-tdTomato (lane 10) results in specific loss of the endogenously tagged LRP6 bands with higher molecular weight, and not the lower molecular weight wt LRP6 bands. These results further confirm that the CRISPR/Cas9 edited cell lines are heterozygous with respect to targeted endogenous tagging of LRP6. The band intensities also confirm that the endogenously tagged LRP6 receptors are expressed at higher levels than their corresponding wt receptors. Vinculin was used as a loading control.
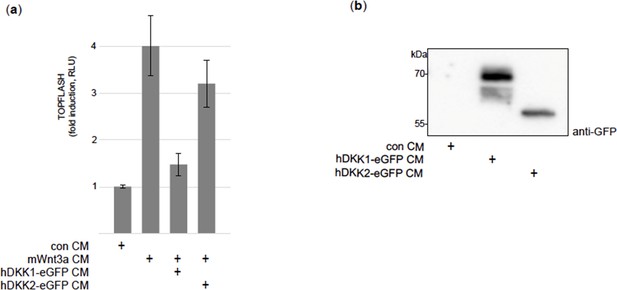
Activity tests and western blot analysis of DKK1-eGFP and DKK2-eGFP.
(a) NCI-H1703 cells in 96-well format were transfected with 20/2 ng TOPFLASH/Renilla reporters. 16 hr post transfection, mWnt3a CM was added for 6 hr, followed by a 12 hr treatment with either DKK1-eGFP or DKK2-eGFP CM, as indicated, before cell lysates were harvested for the luciferase assay. (b) Western blot analysis to visualize the integrity of the DKK1-eGFP and DKK2-eGFP fusion proteins present in the CM. The DKK1 and DKK2 fusion proteins show major bands at ~68 and~58 kDa, respectively. The expected molecular weights are 56 kDa for DKK1-eGFP and 55 kDa for DKK2-eGFP, suggesting that DKK1-eGFP has more post-translational modification(s), which is consistent with the appearance of additional bands for DKK1-eGFP. The amounts of original CM loaded were 0.33 µl for DKK1-eGFP and 10 µl for DKK2-eGFP.
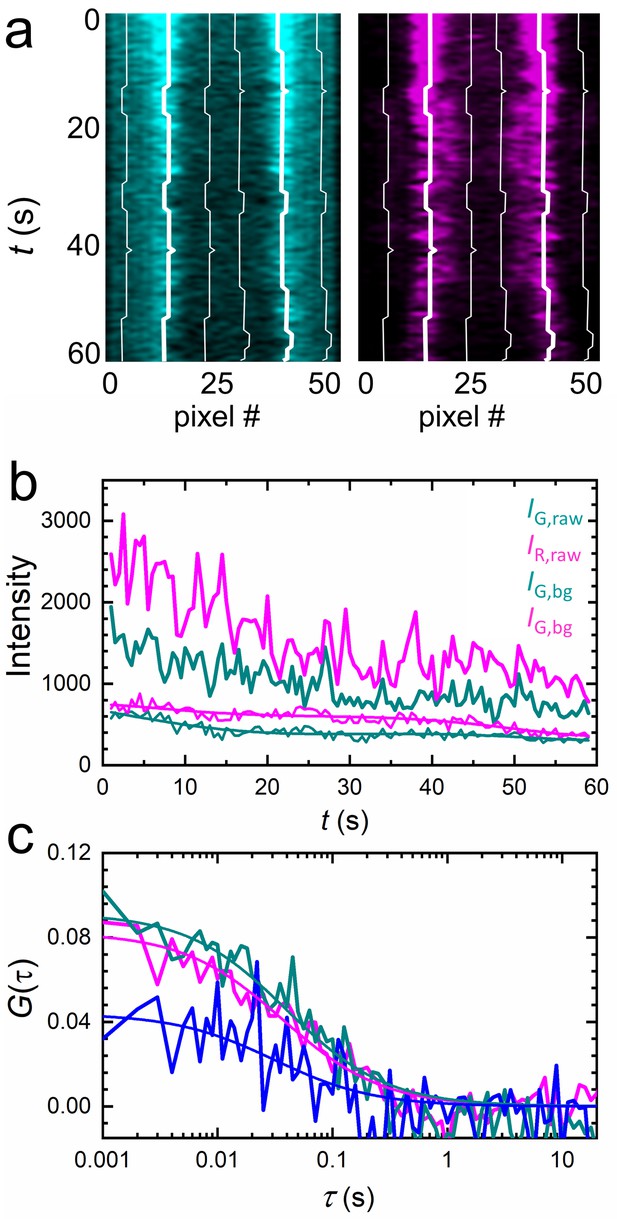
Extraction of correlation functions from axial lsFCS intensity time traces on live NCI-H1703 cells stably transfected with LRP6-mCherry and immersed in CM containing 5 nM DKK1-eGFP.
(a) Kymograph of the membrane scan recorded in the green (ligand, left) and red (receptor, right) color channels, showing the fluorescence from the membrane during 60 s of axial scanning (500 ms binning time). Thick white lines trace the membrane positions. (b) Time traces of the raw ligand (IG,raw) and receptor (IR,raw) intensities from the membrane and the corresponding background, IG,bg and IR,bg, determined ±10 pixels away from the membrane (thin white lines in panel a). Lines through, IG,bg and IR,bg are sums of six sine functions fitted to the data for noise filtering. (c) Autocorrelation (cyan, DKK1-eGFP; magenta, LRP6-mCherry) and cross-correlation (blue) functions after background and photobleaching corrections.
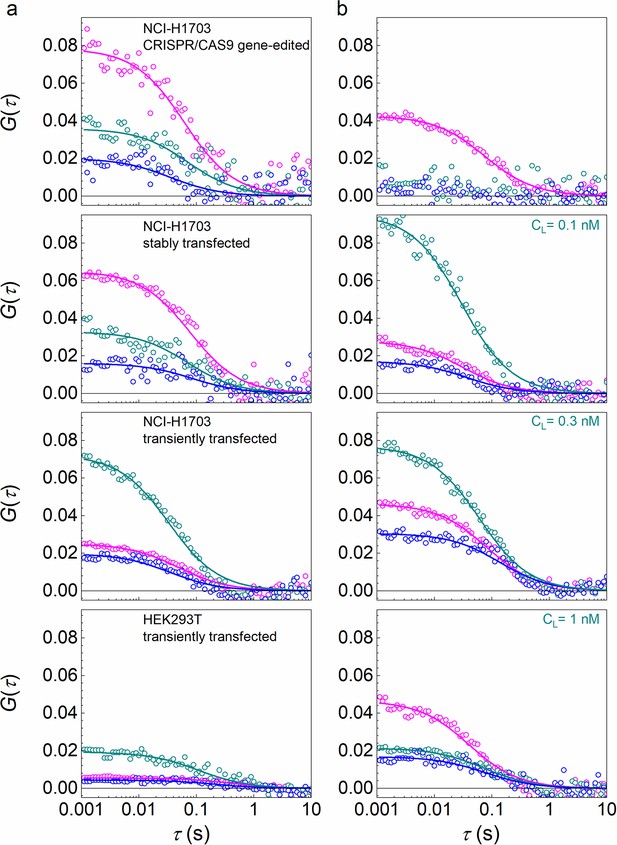
Examples of autocorrelation (cyan, DKK1-eGFP; magenta, LRP6-mCherry) and cross-correlation (blue) functions after background and photobleaching corrections, calculated from individual 60 s axial lsFCS intensity time traces.
(a) Correlation curves at various different receptor densities due to different cell types and receptor expression techniques, all at 0.1 nM DKK1-eGFP. (b) Correlation curves measured on transiently transfected NCI-H1703 cells at various DKK1-eGFP concentrations indicated in the individual panels.
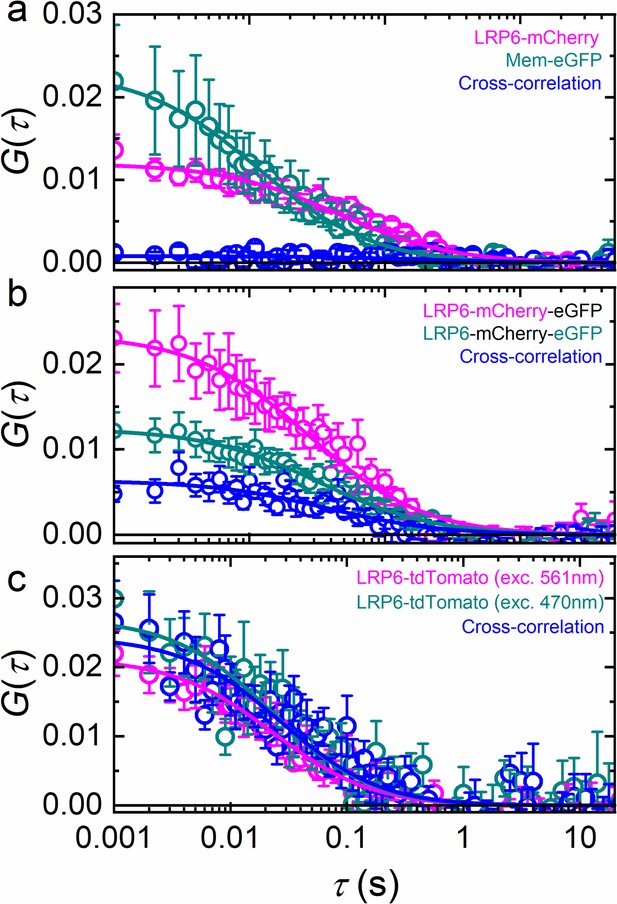
Control experiments demonstrating proper functioning of the axial lsFCS method and analysis, using HEK293T cells transiently transfected to express fluorescently labeled moieties and dual-color fluorescence excitation (PIE) at 470 and 561 nm.
Symbols (cyan and magenta, autocorrelations; blue, cross-correlation) represent averaged data (mean ± SEM) over at least nine axial lsFCS measurements of 60 s each; lines are fits with Equation 1 (a) Correlation functions from cells expressing LRP6-mCherry (magenta) and Mem-eGFP (cyan). Mem is a membrane marker peptide consisting of the 20 N-terminal residues of neuromodulin, which contains a palmitoylation sequence. As expected, the cross-correlation amplitude is zero because both labeled moieties diffuse independently in the plasma membrane. (b) Correlation curves of LRP6 fused to both mCherry and eGFP. The autocorrelation amplitudes are different and reflect the greatly different maturation yields of the two fluoresscent proteins. The cross-correlation amplitude is significant, revealing a sizeable fration of constructs carrying both fluorophores. Notably, the function Γ (Equation 5) equals 0.14 ± 0.02 and is thus identical to the value obtained for HEK293T cells transiently transfected with LRP6-mCherry and exposed to saturating concentrations of DKK1-eGFP (see Figure 6—figure supplement 1, Table 1), as we should expect. (c) Correlation curves of LRP6-tdTomato, with tdTomato excited with 470 nm (4–5 µW) and 561 nm (<1 µW) laser light; emission was detected in the red channel with a bandpass filter 600/37 nm (center/width). Using the same fluorophore for dual-color excitation (PIE), photobleaching is identical for both color channels and perfect cross-correlation is ensured. The cross-correlation amplitude is expected to be (GG(0) + GR(0))/2 for complete overlap of the green and red foci, as we observe in the data.
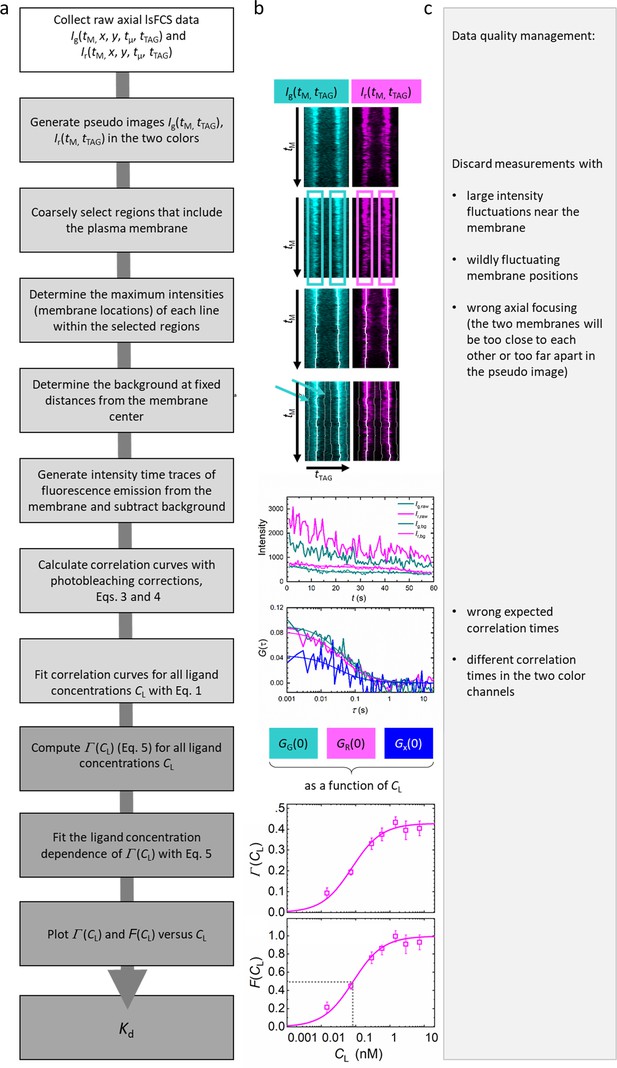
Axial lsFCS - data analysis.
(a) The flow chart lists the individual steps of the data analysis procedure. Tasks in light gray boxes are performed using Matlab. Tasks in dark gray boxes are processed in Origin Pro. (b) Source data files illustrate the individual steps. (c) To obtain reliable and reproducible results, data selection occurs according to the stringent criteria given here.
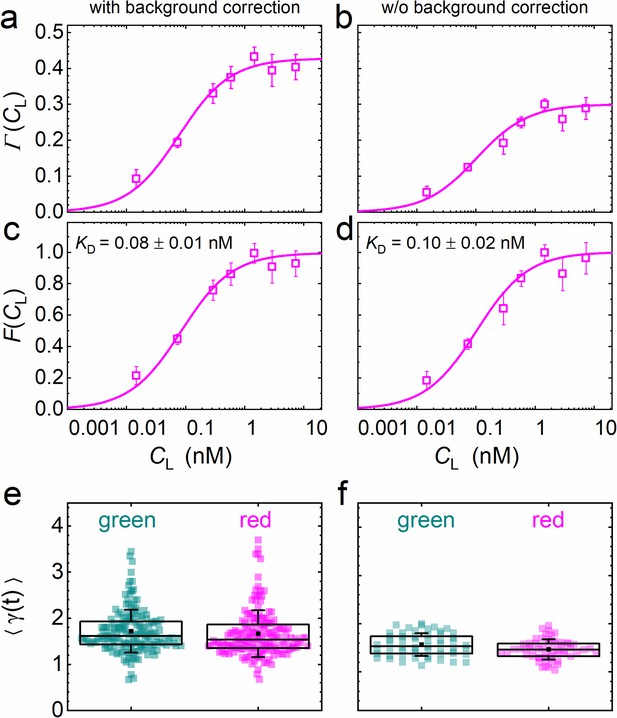
Analysis of the concentration dependence of ligand-receptor binding using axial lsFCS on NCI-H1703 cells stably transfected with LRP6-mCherry and immersed in CM containing DKK1-eGFP in varying concentrations.
Panels on the left and right show the data with and without background correction, respectively. (a, b) Ligand concentration dependence of the function defined in Equation 5. (c, d) Fractional occupancies, F(CL), of receptors with DKK1 ligands. Symbols: experimental data (mean ± SEM) from five axial scans of 60 s each; lines: fits with Equation 5, yielding the parameters KD = 0.08 ± 0.01 nM and β = 0.44 ± 0.02 with background correction and KD = 0.10 ± 0.02 nM and β = 0.31 ± 0.01 without. (e, f) Parameter ⟨γ(t)⟩ used for photobleaching correction of the autocorrelation functions. Each point represents an individual 60 s scan. Boxes mark the 25–75% range. The median is indicated by the central line, the mean as a black square; whiskers indicate the SD.
-
Figure 5—source data 1
Numerical data of the plots in Figure 5.
- https://cdn.elifesciences.org/articles/55286/elife-55286-fig5-data1-v2.xlsx
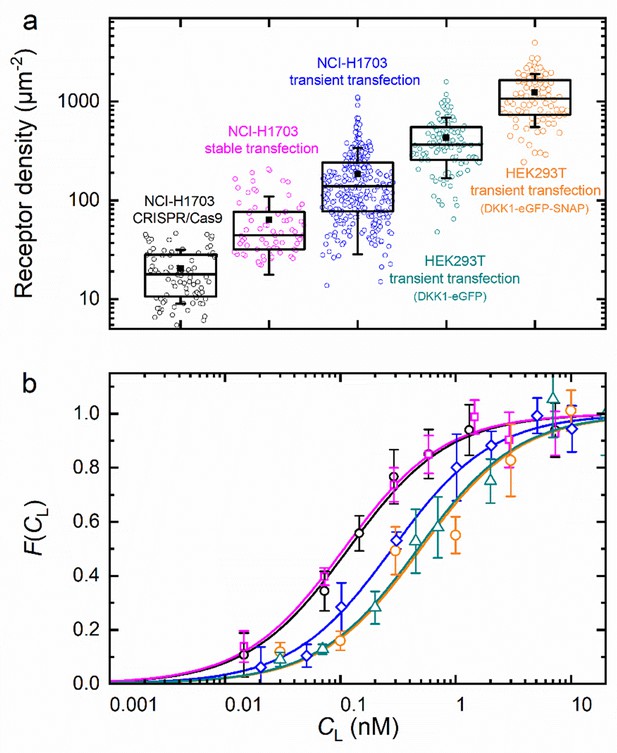
Axial lsFCS on transiently and stably transfected as well as CRISPR/Cas9 gene edited NCI-H1703 cells and transiently transfected HEK293T cells, all expressing LRP6-mCherry on their plasma membranes.
(a) Densities of fluorescently labeled receptors, determined from the autocorrelation amplitudes, GR(0), using ω0 = 0.25 µm. Each data point corresponds to an individual 60-s experiment. Boxes mark the 25 – 75% range; the median is shown as the central line and the mean as a black square. Whiskers indicate the SD. (b) Fractional occupancies, , of receptors with Dkk1-eGFP as a function of the ligand concentration in the CM. Symbols (shapes/colors as in panel a), experimental data (mean ± SEM from at least three axial scans of 60 s each); lines, fits with Equation 5.
-
Figure 6—source data 1
Numerical data of the plots in Figure 6.
- https://cdn.elifesciences.org/articles/55286/elife-55286-fig6-data1-v2.xlsx
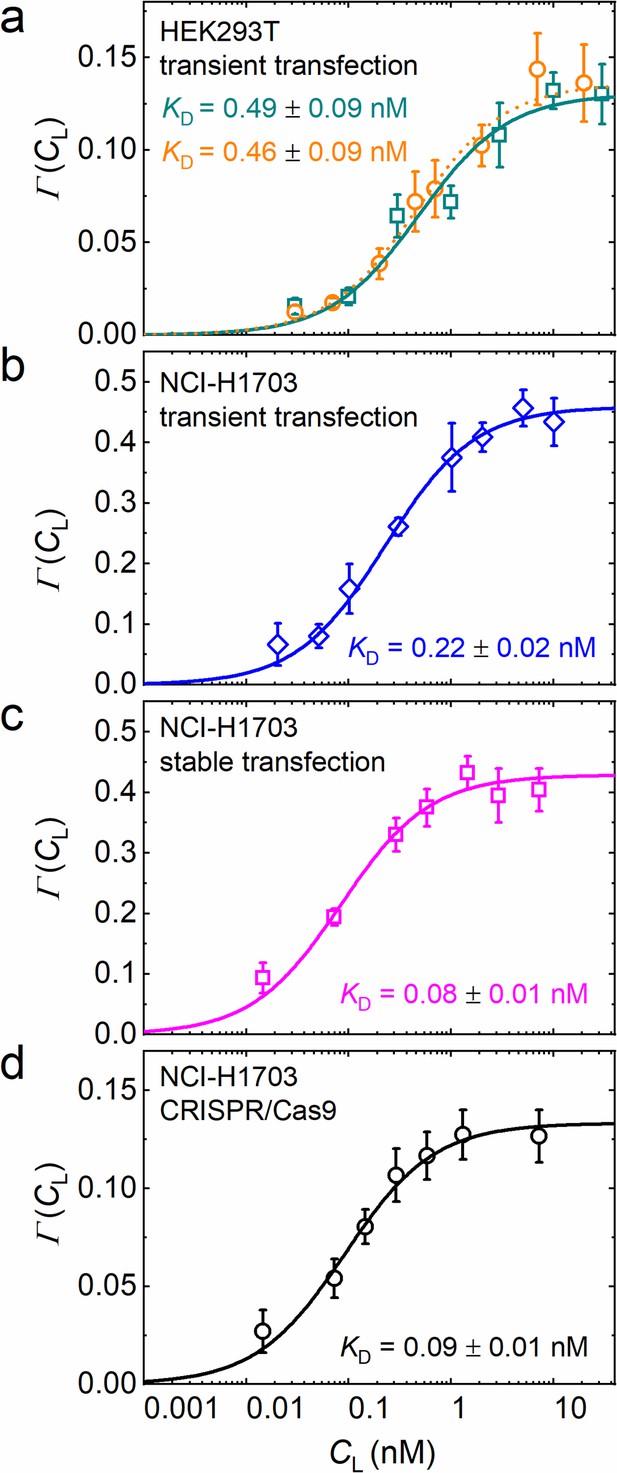
Axial lsFCS on HEK293T and NCI-H1703 cells transfected with LRP6-mCherry, measured at 37°C and 5% CO2.
Г(CL) is plotted as a function of the DKK1-eGFP concentration CL. (a) Transiently transfected HEK293T cells. Data taken with DKK1-eGFP-SNAP as a ligand are included in cyan. (b) Transiently transfected NCI-H1703 cells. (c) Stably transfected NCI-H1703 cells. (d) NCI-H1703 cells expressing endogenously tagged LRP6-mCherry after CRISPR/Cas9 genome editing. Symbols, data; lines, fits with Equation 5.
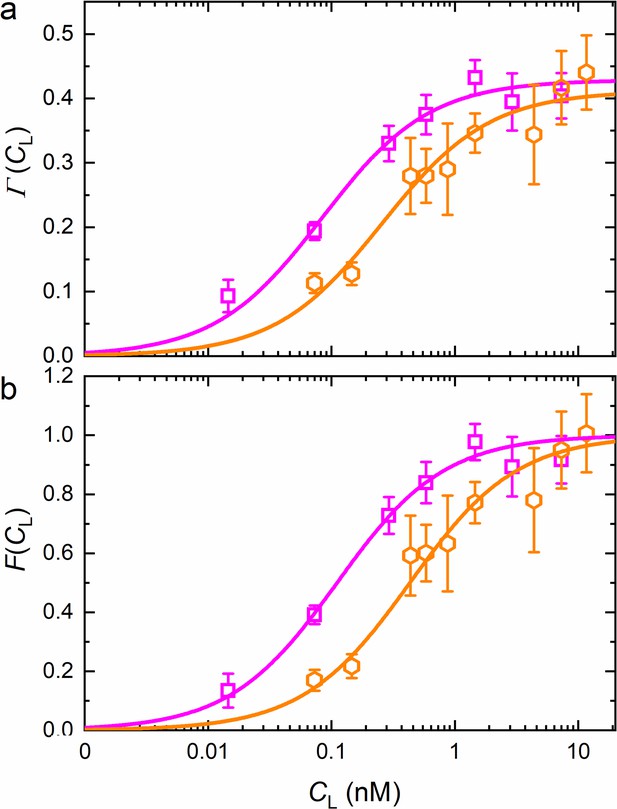
Comparison of DKK1-eGFP and DKK2-eGFP binding to NCI-H1703 cells stably transfected with LRP6-mCherry.
(a) Г(CL) as a function of DKK1-eGFP (magenta) and DKK2-eGFP (orange) ligand concentrations CL. Symbols represent data (mean ± SEM) from five axial scans of 60 s each; lines are fits with Equation 5, yielding the parameters KD = 0.08 ± 0.01 nM and β = 0.44 ± 0.02 for DKK1-eGFP and KD = 0.26 ± 0.04 nM and β = 0.43 ± 0.02 for DKK2-eGFP. (b) Corresponding binding isotherm F(CL) as a function of DKK1-eGFP and DKK2-eGFP ligand concentrations.
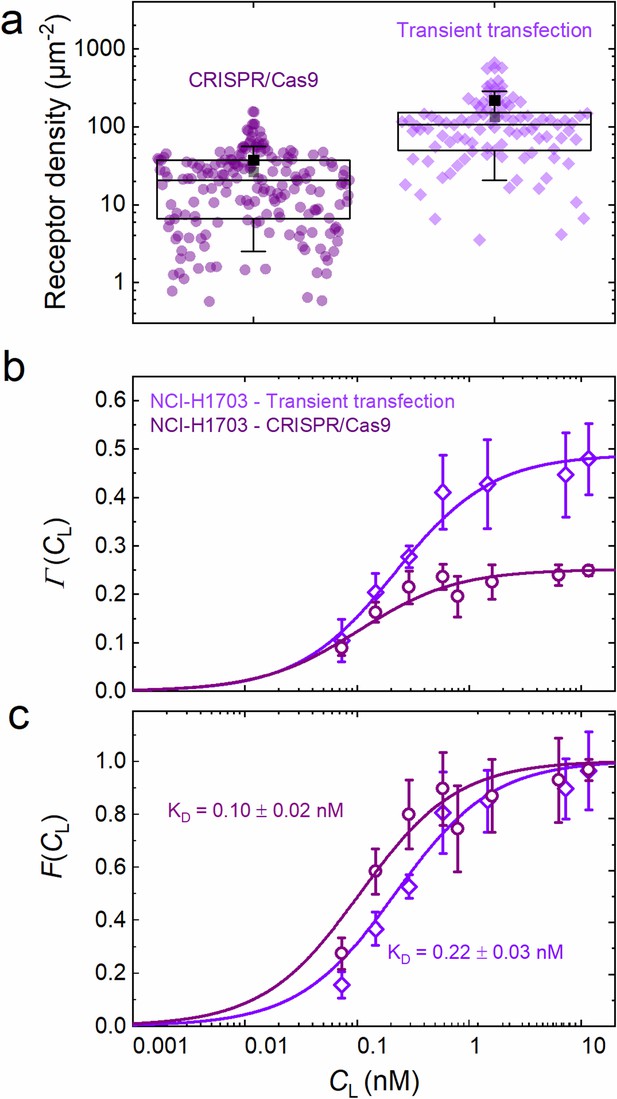
Axial lsFCS on transiently transfected and CRISPR/Cas9 endogenously tagged NCI-H1703 cells expressing LRP6-tdTomato.
(a) Receptor densities, each data point represents an individual cell, boxes mark the 25–75% range. The median is indicated by the central line, the mean as a small black square. Whiskers mark the SD. (b) Г(CL) and (c) corresponding binding isotherms F(CL) as functions of DKK1-eGFP ligand concentration CL. Symbols represent data obtained from five axial scans of 60 s each (mean ± SD); lines are fits with Equation 5.
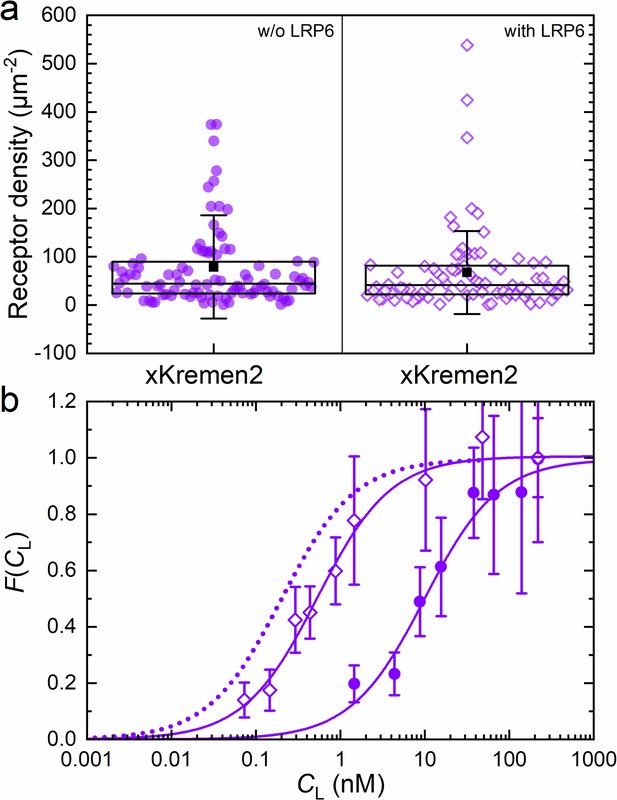
Axial line scanning FCS on transiently transfected NCI-H1703 cells expressing xKremen2-mCherry on the plasma membrane.
(a) Densities of fluorescently labeled receptors, determined from the autocorrelation amplitudes, GR(0), with ω0 = 0.25 µm. Data on the left and right show results without and with LRP6 cotransfection. Each symbol represents data from an individual 60-s experiment. Boxes mark the 25 – 75% range. The median is shown as the central line and the mean as a black square; whiskers indicate the SD. (b) Fractional occupancies, , of receptors with DKK1-eGFP as a function of the ligand concentration in the CM. Open and closed symbols, experimental data (mean ± SEM from at least three axial scans of 60 s each) from cells with and without LRP6 co-transfection, respectively; solid lines, binding isotherms from fits with Equation 5; dotted line, DKK1-eGFP binding curve upon transfection with LRP6 only (for comparison).
-
Figure 7—source data 1
Numerical data of the plots in Figure 7.
- https://cdn.elifesciences.org/articles/55286/elife-55286-fig7-data1-v2.xlsx
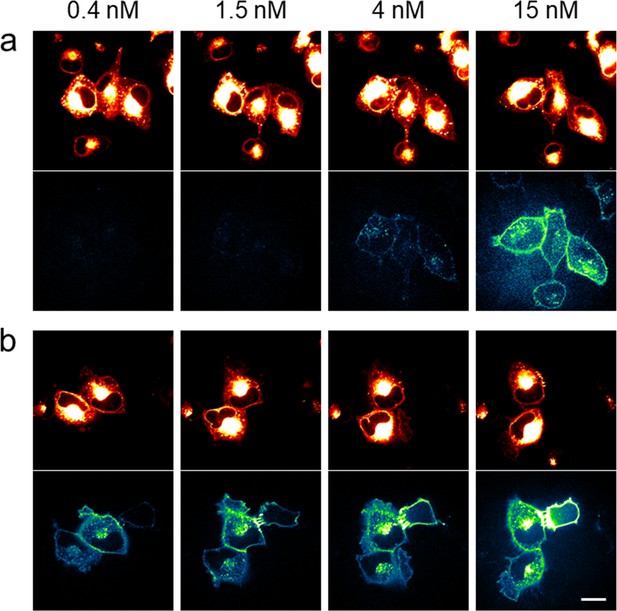
Dual-color spinning disk confocal images of live NCI-H1703 cells incubated in CM with four different DKK1-eGFP concentrations (indicated on top).
Red, xKremen2-mCherry; green, DKK1-eGFP. (a) Transiently transfected cells expressing xKremen2-mCherry. (b) Cells additionally co-transfected to express unlabeled LRP6. With LRP6 cotransfection, DKK1-eGFP staining is already pronounced at 0.4 nM, whereas it is only well visible at 15 nM without, suggesting that the presence of LRP6 strongly enhances the binding affinity of DKK1 toward xKremen2. Within each data set, images are shown with the same intensity and contrast settings. Scale bar, 20 µm.
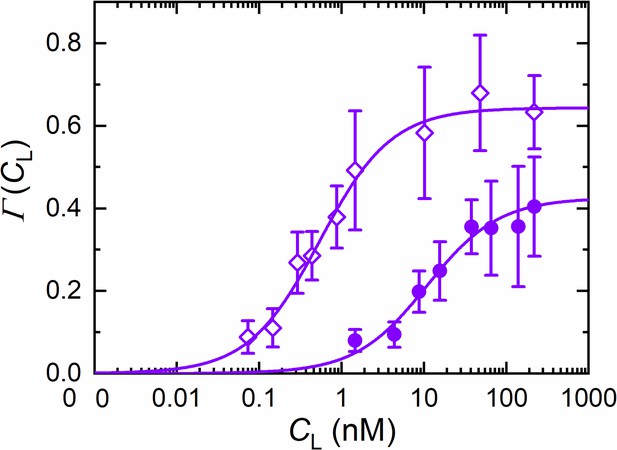
Axial lsFCS on NCI-H1703 cells transfected with xKremen2-mCherry.
Dependence of Г(CL) on the DKK1-eGFP ligand concentration CL. Open symbols: with LRP6 cotransfection; closed symbols: without LRP6 cotransfection; symbols represent data (mean ± SD) obtained from five axial scans of 60 s each. Lines are fits with Equation 5; fit parameters are given in Table 2.
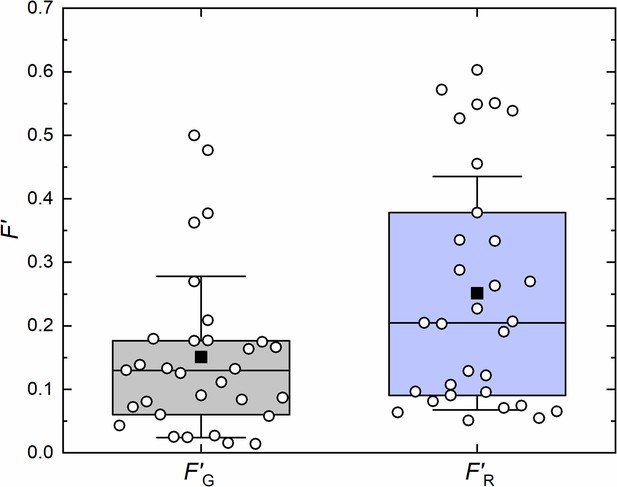
Apparent fractions of red and green receptors engaged in heterodimeric complexes in the plasma membrane of NCI-H1703 cells stably expressing LRP6-mCherry.
Each symbol represents a data point from an axial lsFCS measurement on a single cell. Only data with correlation amplitudes GG > 0.02 and F’<1 have been included to remove statistical outliers. Box indicates the 25–75% range; the median is shown as the central line and the mean as a black square. Whiskers mark the SD.
Tables
Parameters obtained from the analysis of axial lsFCS data of DKK1 binding to LRP6-mCherry.
Cell line | HEK293T | NCI-H1703 | |||
---|---|---|---|---|---|
Receptor gene insertion | Transient transfection | Transient transfection | Stable transfection | CRISPR/Cas9 genome editing | |
Ligand | DKK1-eGFP | DKK1-eGFP-SNAP | DKK1-eGFP | ||
Receptor density (µm‒2) * | 370 ± 150 | 1080 ± 470 | 140 ± 80 | 45 ± 23 | 18 ± 9 |
KD (nM) | 0.49 ± 0.09 | 0.46 ± 0.09 | 0.22 ± 0.02 | 0.08 ± 0.01 | 0.09 ± 0.01 |
β | 0.13 ± 0.01 | 0.15 ± 0.01 | 0.47 ± 0.01 | 0.44 ± 0.02 | 0.14 ± 0.01 |
DG (µm2 s‒1) † | 0.44 ± 0.08 | 0.40 ± 0.07 | 0.45 ± 0.11 | 0.29 ± 0.06 | 0.33 ± 0.15 |
DR (µm2 s‒1) † | 0.46 ± 0.09 | 0.45 ± 0.11 | 0.42 ± 0.13 | 0.27 ± 0.05 | 0.33 ± 0.06 |
⟨γG⟩‡ | 1.32 ± 0.11 | 1.25 ± 0.24 | 1.36 ± 0.21 | 1.62 ± 0.25 | 1.54 ± 0.23 |
⟨γR⟩‡ | 1.21 ± 0.08 | 1.26 ± 0.13 | 1.33 ± 0.16 | 1.54 ± 0.25 | 1.42 ± 0.29 |
-
* Receptor densities are given as median ± half the range covered by the second and third quartile of the distribution. Individual data points were calculated from receptor autocorrelation amplitudes, GR(0), and the observation area (0.20 µm2).
† Diffusion coefficients of receptor-bound ligands and receptors were calculated from the translational diffusion times by using D = ω02/4τ.
-
‡ The photobleaching parameters ⟨γ⟩ are given as the median value ± half the range covered by the second and third quartile of the distribution compiled from all scans.
-
Table 1—source data 1
Source Data to Table 1.
- https://cdn.elifesciences.org/articles/55286/elife-55286-table1-data1-v2.docx
Parameters obtained from the analysis of axial lsFCS data on NCI-1703 cells.
Receptor | LRP6-mCherry | LRP6-tdTomato | LRP6-tdTomato | xKremen2-mCherry | xKremen2- mCherry, LRP6 |
---|---|---|---|---|---|
Receptor gene insertion | Stable transfection | Transient transfection | CRISPR/Cas9 genome editing | Transient transfection | Transient transfection |
Ligand | DKK2-eGFP | DKK1-eGFP | DKK1-eGFP | DKK1-eGFP | DKK1-eGFP |
Receptor density (µm‒2) * | 32 ± 16 | 106 ± 52 | 21 ± 15 | 44 ± 33 | 41 ± 30 |
KD (nM) | 0.26 ± 0.04 | 0.22 ± 0.03 | 0.10 ± 0.02 | 10.3 ± 2.1 | 0.54 ± 0.06 |
β | 0.43 ± 0.02 | 0.51 ± 0.02 | 0.26 ± 0.01 | 0.45 ± 0.02 | 0.66 ± 0.03 |
DG (µm2 s‒1) † | 0.27 ± 0.08 | 0.35 ± 0.19 | 0.27 ± 0.19 | 0.25 ± 0.15 | 0.32 ± 0.18 |
DR (µm2 s‒1) † | 0.30 ± 0.07 | 0.44 ± 0.34 | 0.39 ± 0.19 | 0.19 ± 0.08 | 0.27 ± 0.09 |
⟨γG⟩‡ | 1.22 ± 0.17 | 1.31 ± 0.15 | 1.47 ± 0.25 | 1.36 ± 0.22 | 1.30 ± 0.22 |
⟨γR⟩‡ | 1.41 ± 0.19 | 1.41 ± 0.16 | 1.66 ± 0.39 | 1.42 ± 0.29 | 1.35 ± 0.16 |
-
*Receptor densities were calculated from the amplitudes of the receptor autocorrelation functions, GR(0), and the observation area (0.20 µm2).
† Diffusion coefficients of receptor-bound ligands and receptors were calculated from the translational diffusion times by using D = ω02/4τ.
-
‡ The photobleaching parameters ⟨γ⟩ are given as the median of the distribution from all scans; the errors denote half the width of the second and third quartile of the distribution.
-
Table 2—source data 1
Source Data to Table 2.
- https://cdn.elifesciences.org/articles/55286/elife-55286-table2-data1-v2.docx