DAZL mediates a broad translational program regulating expansion and differentiation of spermatogonial progenitors
Figures
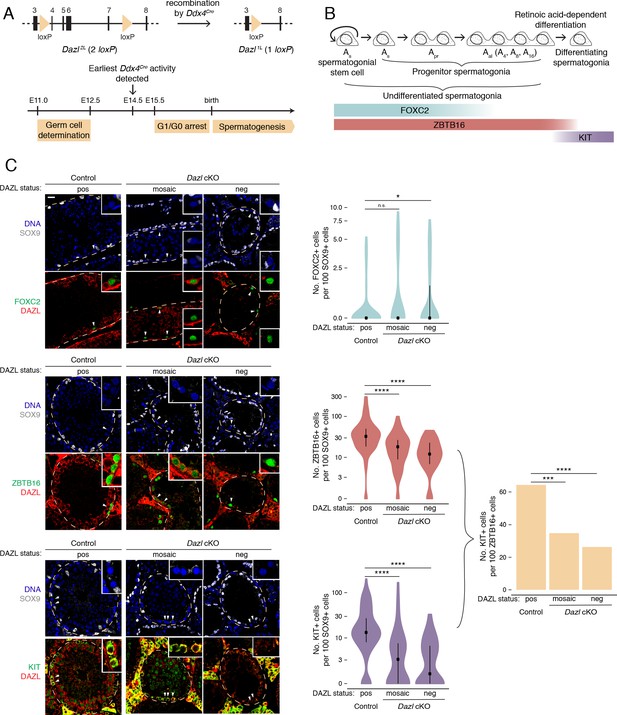
DAZL promotes spermatogonial expansion and differentiation.
(A) Schematic of Dazl2L (two loxP) and Dazl1L (one loxP) alleles. Recombination of Dazl2L allele via Ddx4Cre allele yields Dazl1L allele in germ cells. (B) Schematic of spermatogenesis from Asingle (As) spermatogonial stem cells to progenitor spermatogonia to differentiating spermatogonia, with expression of spermatogonial markers. As spermatogonial stem cells self-renew while also giving rise to As progenitor spermatogonia, which differentiate without self-renewal. Subsequent divisions with incomplete cytokinesis produce chains of two (Apaired, Apr) as well as chains of four, eight, and sixteen (Aaligned, Aal) progenitor spermatogonia. Progenitors form differentiating spermatogonia in response to retinoic acid. In addition, chains of Apr and Aal progenitors can fragment to form As spermatogonial stem cells, particularly under conditions of stress (not shown). (C) Quantification of FOXC2, ZBTB16, and KIT-positive spermatogonia (green) in histological sections of control and Dazl cKO adult testes. SOX9 marks Sertoli cells (gray), DAZL is in red, and DAPI marks DNA (blue). Select tubules are outlined via dotted orange line. Cells enlarged within insets are highlighted by arrowheads. Insets show spermatogonia that are DAZL-positive and FOXC2-positive (control and mosaic cKO panels); DAZL-negative and FOXC2-positive (mosaic and DAZL-negative cKO panels); DAZL-positive and ZBTB16-positive (control and mosaic cKO panels); DAZL-negative and ZBTB16-positive (DAZL-negative cKO panel); DAZL-positive and KIT-positive (control and mosaic cKO panels); and DAZL-negative and KIT-negative (DAZL-negative cKO panel). Scale bar = 20 μm. Populations were quantified from 50 tubules per animal, with two animals per genotype. Violin plots display medians with interquartile ranges. Difference between normalized FOXC2, ZBTB16, and KIT-positive populations was statistically assessed via two-sided Mann-Whitney U test. Difference between normalized KIT-positive populations over normalized ZBTB16-positive populations was statistically assessed via two-sided odds ratio. *, p<0.05; ***, p<0.001; ****, p<0.0001.
-
Figure 1—source data 1
RNA-seq analysis of Pou5f1:EGFP-positive spermatogonia from control and Dazl cKO testes.
- https://cdn.elifesciences.org/articles/56523/elife-56523-fig1-data1-v2.xlsx
-
Figure 1—source data 2
Quantification of spermatogonial subpopulations and tubule cross-section area in control and Dazl cKO testes.
- https://cdn.elifesciences.org/articles/56523/elife-56523-fig1-data2-v2.xlsx
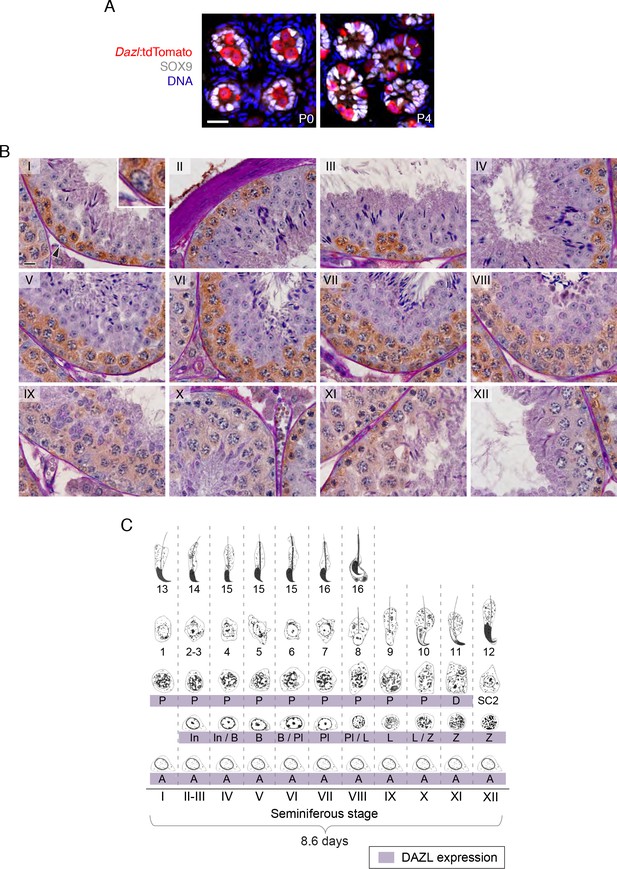
DAZL expression in postnatal gonocytes and during spermatogenesis.
(A) Male gonocytes at postnatal days (P) 0 and 4 express the Dazl:tdTomato reporter. SOX9 marks Sertoli cells. Scale bar = 20 μm. (B) DAZL immunohistochemistry in adult testis. DAZL localized to type A, intermediate, and type B spermatogonia as well as spermatocytes from the preleptotene through diplotene stages. Roman numeral designates stage of the primary tubule in each panel. Type A spermatogonium highlighted by arrowhead in stage I tubule is enlarged within inset. Scale bar = 10 μm. (C) Summary of DAZL expression by stage of spermatogenesis, based on immunohistochemistry in B. Abbreviations, in spermatogenic order: A, type A spermatogonium; In, intermediate spermatogonium; B, type B spermatogonium; Pl, preleptotene spermatocyte; L, leptotene spermatocyte; Z, zygotene spermatocyte; D, diplotene spermatocyte; SC2, secondary spermatocytes. The numbers indicate the ‘step’ staging designation of the corresponding spermatids.
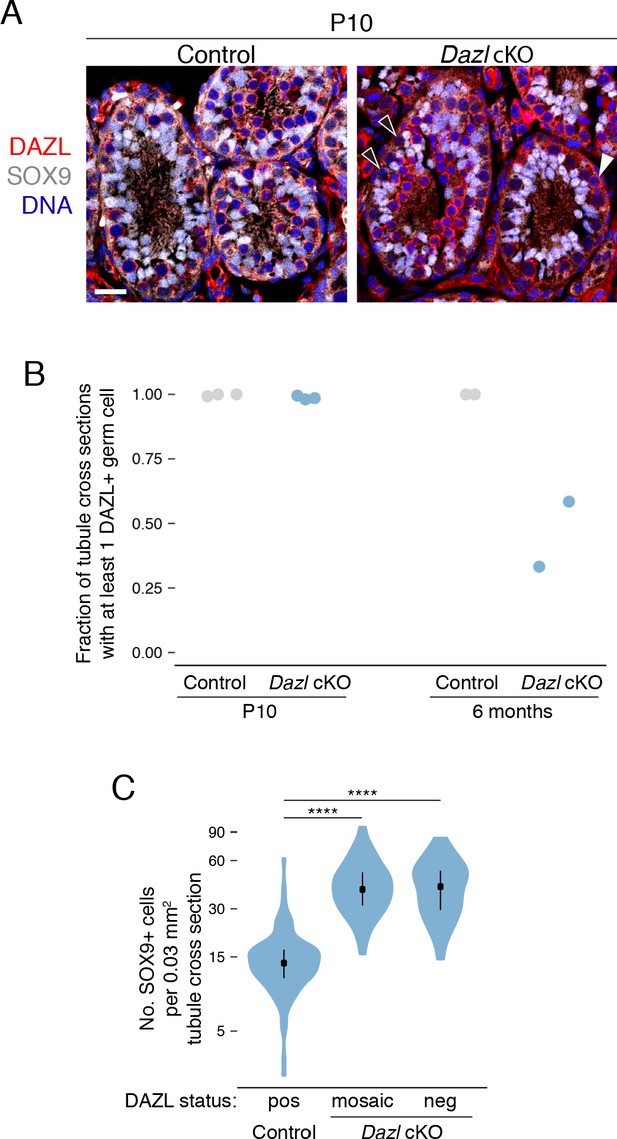
Conditional deletion of Dazl in spermatogonia.
(A) DAZL protein staining (red) in control and Dazl cKO P10 testes. SOX9 marks Sertoli cells (gray) and DAPI marks DNA (blue). DAZL-positive and -negative germ cells marked by white and black arrowheads, respectively. Scale bar = 20 μm. (B) Percentage of seminiferous tubule cross sections exhibiting at least one DAZL+ germ cell at postnatal day 10 (P10) and at 6 months. Each data point represents quantification from a single animal. At least 50 tubule cross sections were quantified per animal. (C) Quantification of SOX9-positive Sertoli cells per 0.03 mm2 area of seminiferous tubule cross section (two-sided Mann-Whitney U test). The area of the average tubule cross section analyzed in Figure 1C was 0.03 mm2. ****, p<0.0001.
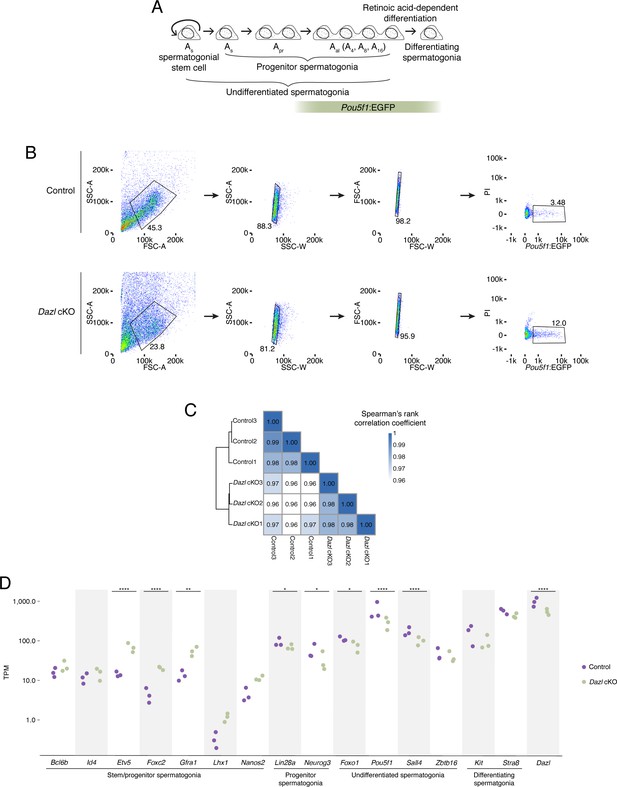
Characterization of Pou5f1:EGFP-positive spermatogonia in Dazl cKO.
(A) Schematic of spermatogenesis from undifferentiated spermatogonia through differentiating spermatogonia, with expression of Pou5f1:EGFP. (B) Schematic depicting the sorting strategy used to isolate Pou5f1:EGFP-positive spermatogonia from control and Dazl cKO adult testes. The GFP+ population (right panel) was collected for RNA-seq. (C) Heatmap of sample-to-sample distances among RNA-seq datasets of sorted Pou5f1:EGFP-positive spermatogonia from control and Dazl cKO testes. Three biological replicates were analyzed for each genotype. (D) Differential gene expression analysis of RNA-seq analysis of Pou5f1:EGFP-positive spermatogonia from adult testes, highlighting genes associated with spermatogonial stem cells and early progenitors (‘stem/progenitor spermatogonia’), progenitor spermatogonia, undifferentiated spermatogonia (i.e., spermatogonial stem cells as well as early and late progenitors), and differentiating spermatogonia. * adjusted p<0.05, ** adjusted p<0.01, *** adjusted p<0.001, **** adjusted p<0.0001.
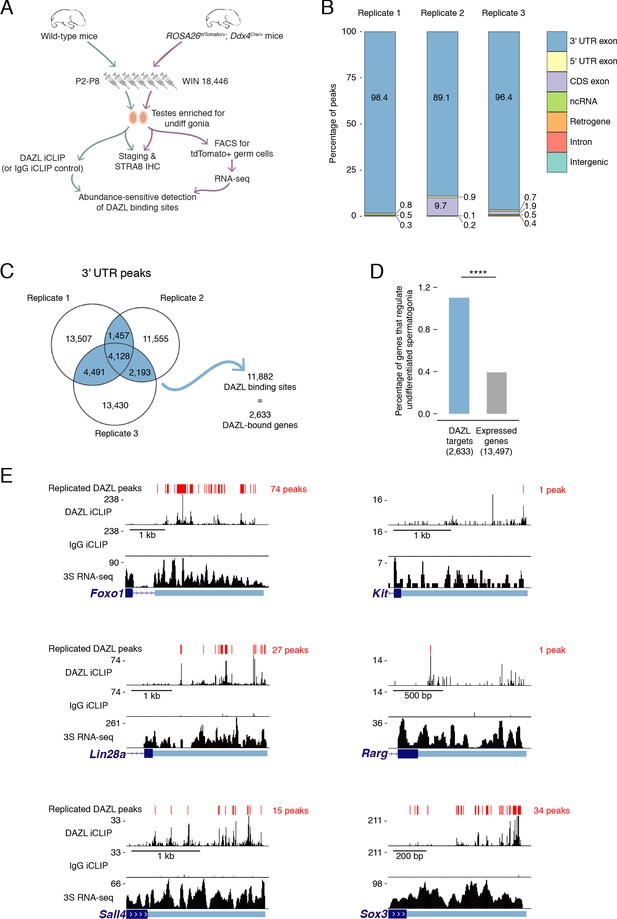
Identification of DAZL-bound transcripts in undifferentiated spermatogonia via iCLIP reveals DAZL’s regulation of spermatogonial factors.
(A) Schematic of the synchronization of spermatogenesis to obtain undifferentiated spermatogonia via the 2S method for iCLIP (green arrows) and via the 3S method for RNA-seq (purple arrows). WIN 18,446 was used to synchronize spermatogenesis by blocking spermatogonial differentiation, and samples were histologically staged to verify successful synchronization. For 3S samples, germ cells were sorted from synchronized testes. (B) Genomic distribution of DAZL iCLIP peaks identified in three biological replicates (TPM ≥1; FDR < 0.05). (C) Venn diagram showing overlap of DAZL iCLIP peaks in expressed 3' UTRs (TPM ≥1) among three biological replicates. Replicated peaks (i.e., present in at least two of three replicates) were identified. After merging replicated peaks that fell on consecutive nucleotides, 11,882 DAZL binding sites (present in at least two of three replicates; highlighted in blue) were identified. These binding sites correspond to 2,633 genes, which are designated as the DAZL-bound genes. (D) Enrichment of factors that regulate the development and differentiation of undifferentiated spermatogonia in DAZL targets compared with all genes expressed in undifferentiated spermatogonia (one-tailed hypergeometric test). Number of genes (n) in each group designated in parentheses in labels along x-axis.****, p<0.0001. (E) DAZL iCLIP, IgG iCLIP, and 3S RNA-seq gene tracks showing exemplary DAZL-bound genes that are required for spermatogonial proliferation and expansion (Lin28a and Sox3) or differentiation (Kit, Foxo1, Rarg, and Sall4). Each iCLIP track represents the crosslinked sites from the sum of unique reads from three biological replicates. The RNA-seq track represents the sum of two biological replicates. The scale of each gene track is marked on the left. 3' UTRs are in light blue.
-
Figure 2—source data 1
Genes that regulate development and differentiation in undifferentiated spermatogonia.
- https://cdn.elifesciences.org/articles/56523/elife-56523-fig2-data1-v2.xlsx
-
Figure 2—source data 2
Replicated DAZL iCLIP peaks and transcript expression levels in 3S undifferentiated spermatogonia.
- https://cdn.elifesciences.org/articles/56523/elife-56523-fig2-data2-v2.xlsx
-
Figure 2—source data 3
Comparison of 3S undifferentiated spermatogonia to previously published spermatogonial datasets.
- https://cdn.elifesciences.org/articles/56523/elife-56523-fig2-data3-v2.xlsx
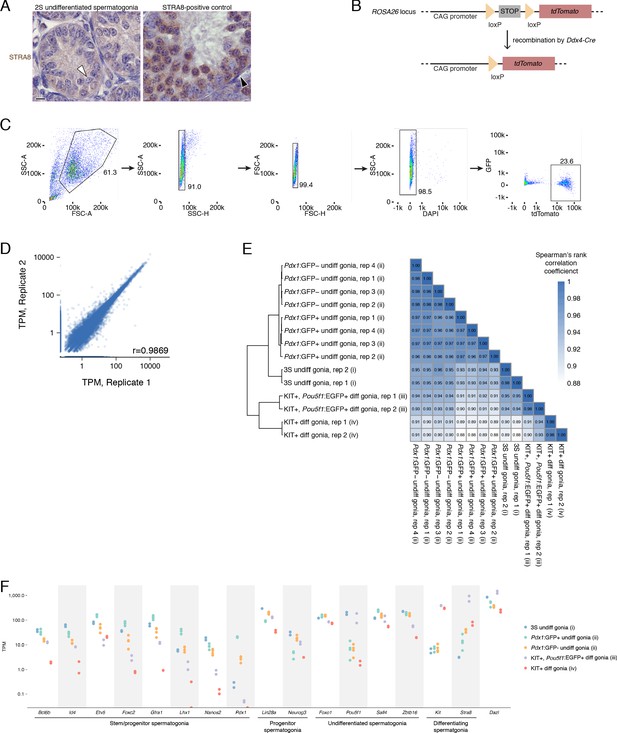
Isolation of undifferentiated spermatogonia via the 2S (synchronization and staging) and 3S (synchronization, staging, and sorting) strategies.
(A) The absence of STRA8 expression in WIN 18,446-synchronized testes immunohistologically confirms the accumulation of undifferentiated spermatogonia. White arrowhead highlights a STRA8-negative type A spermatogonium. Testes synchronized for preleptotene spermatocytes were used as a positive control for STRA8 immunohistology. Black arrowhead highlights a STRA8-positive differentiating spermatogonium; the majority of the STRA8-positive cells in this section are preleptotene spermatocytes. (B) Schematic of the Cre reporter tdTomato. Recombination of the tdTomato allele via the Ddx4Cre allele removes the floxed stop codon and activates expression of tdTomato protein specifically in germ cells. (C) Gating strategy for sorting undifferentiated spermatogonia after synchronization. Undifferentiated spermatogonia were sorted from 2S testes enriched for undifferentiated spermatogonia. The DAPI-negative population represents the live cell fraction, and the tdTomato-positive population represents the undifferentiated spermatogonia that were collected for RNA-seq. (D) Correlation between biological replicates of TPM expression values for protein-coding genes, noncoding RNA (excluding rRNA), and retrogenes with TPM ≥1. Pearson’s correlation coefficient shown. (E) Heatmap of sample-to-sample distances between RNA-seq datasets from (i) our 3S undifferentiated spermatogonia; (ii) Pdx1:GFP-positive undifferentiated spermatogonia and Pdx1:GFP-negative undifferentiated spermatogonia from 6 to 8 week old adult testes La et al., 2018a; (iii) KIT-positive, Pou5f1:EGFP-positive differentiating spermatogonia from P7 testes Kubo et al., 2015; (iv) KIT-positive differentiating spermatogonia from P7 testes (Maezawa et al., 2017). RNA-seq data for protein-coding genes were quantified, normalized and transformed via DESeq prior to calculating sample distances. (F) Expression of markers genes for spermatogonial stem cells and early progenitors (‘stem/progenitor spermatogonia’), progenitor spermatogonia, undifferentiated spermatogonia (i.e., spermatogonial stem cells as well as early and late progenitors), and differentiating spermatogonia in the RNA-seq datasets analyzed in E.
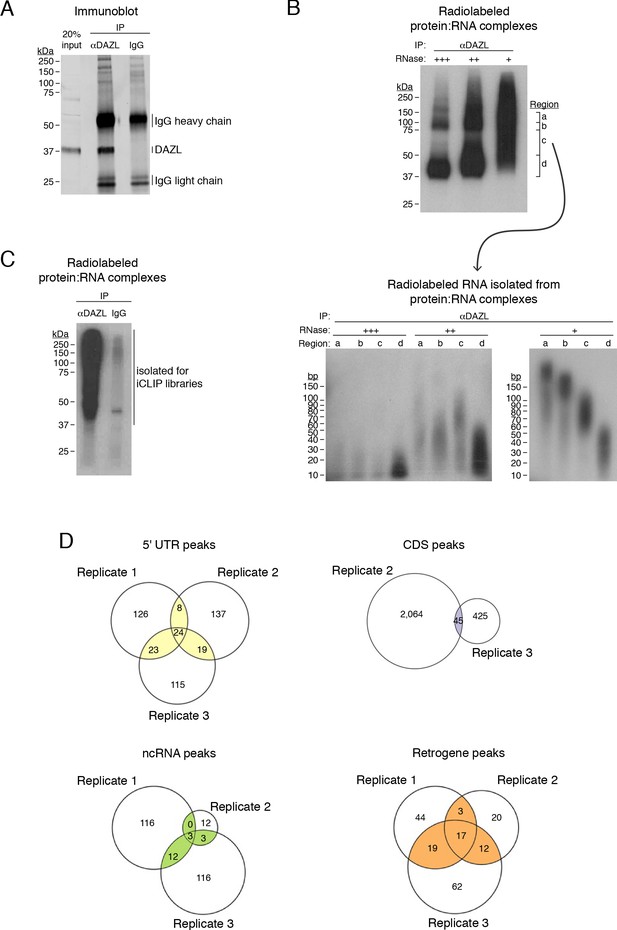
DAZL iCLIP.
(A) DAZL immunoblot from P20 testes. Immunoprecipitation (IP) and Western blotting identifies DAZL at ~37 kDa. (B) Radiolabeled DAZL:RNA complexes from P20 testes (upper blot) and radiolabeled RNA isolated from these DAZL:RNA complexes (lower blot). Prior to radiolabeling, DAZL:RNA complexes were subjected to digestion by RNAse I at high (+++), medium (++), and low (+) concentrations. High RNAse concentration identifies DAZL:RNA complexes just above 37 kDa, where DAZL protein alone is found, and at ~75 kDa, which is consistent with DAZL:RNA complexes containing two DAZL proteins bound to a single RNA fragment. To obtain RNA of a sufficient length for preparing cDNA libraries, the low concentration of RNAse I was used to prepare iCLIP libraries. (C) Radiolabeled DAZL:RNA complexes from postnatal testes synchronized for undifferentiated spermatogonia. One of three biological replicates used to prepare iCLIP libraries is shown. (D) Venn diagram showing overlap of DAZL iCLIP peaks in expressed transcripts (TPM ≥1) from each type of genomic region, other than 3' UTRs, among three biological replicates.
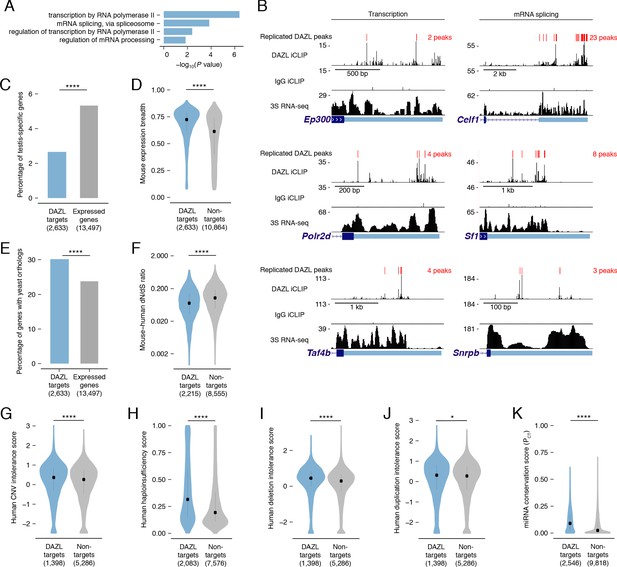
DAZL targets are enriched for broadly expressed, dosage-sensitive regulators of basic cellular processes.
(A) All statistically enriched GO biological processes, excluding parental nodes, for 2,633 DAZL-targeted genes compared with all genes expressed in undifferentiated spermatogonia (TPM ≥1) using the PANTHER GO Slim annotation set. P values are from binomial tests with Bonferroni correction. (B) DAZL iCLIP, IgG iCLIP, and 3S RNA-seq gene tracks showing exemplary DAZL-bound genes that are involved in transcription and RNA splicing. Gene tracks as described in Figure 2E. (C) DAZL targets are depleted of testis-specific factors compared with all genes expressed in undifferentiated spermatogonia (one-tailed hypergeometric test). (D) DAZL targets are more broadly expressed than nontargets within 12 adult mouse tissues (one-sided Mann-Whitney U test). (E) DAZL targets are more likely to have a yeast ortholog (one-tailed hypergeometric test). (F) DAZL targets have a reduced ratio of nonsynonymous substitutions per nonsynonymous site to synonymous substitutions per synonymous site (dN/dS) in alignments with human orthologs (one-sided Mann-Whitney U test). (G) Human orthologs of DAZL’s targets exhibit increased intolerance for copy number variation (CNV) (one-sided Mann-Whitney U test). (H) The human orthologs of DAZL’s targets exhibit increased probability of haploinsufficiency (one-sided Mann-Whitney U test). (I) The human orthologs of DAZL’s targets exhibit increased intolerance for deletions (one-sided Mann-Whitney U test). (J) The human orthologs of DAZL’s targets exhibit increased intolerance for duplications (one-sided Mann-Whitney U test). (K) DAZL targets exhibit higher miRNA conservation scores (PCT) (one-sided Mann-Whitney U test). Violin plots display medians with interquartile ranges; number of genes (n) in each group designated in parentheses in labels along x-axis. *, p<0.05; ****, p<0.0001.
-
Figure 3—source data 1
GO analysis of DAZL targets.
- https://cdn.elifesciences.org/articles/56523/elife-56523-fig3-data1-v2.xlsx
-
Figure 3—source data 2
Testis-specific genes and expression breadth for genes expressed in undifferentiated spermatogonia.
- https://cdn.elifesciences.org/articles/56523/elife-56523-fig3-data2-v2.xlsx
-
Figure 3—source data 3
Mouse:yeast orthology status for genes expressed in undifferentiated spermatogonia.
- https://cdn.elifesciences.org/articles/56523/elife-56523-fig3-data3-v2.xlsx
-
Figure 3—source data 4
dN/dS ratios of mouse:human orthologs for genes expressed in undifferentiated spermatogonia.
- https://cdn.elifesciences.org/articles/56523/elife-56523-fig3-data4-v2.xlsx
-
Figure 3—source data 5
Haploinsufficiency scores, ExAC data, and mean miRNA PCT scores for genes expressed in undifferentiated spermatogonia.
- https://cdn.elifesciences.org/articles/56523/elife-56523-fig3-data5-v2.xlsx
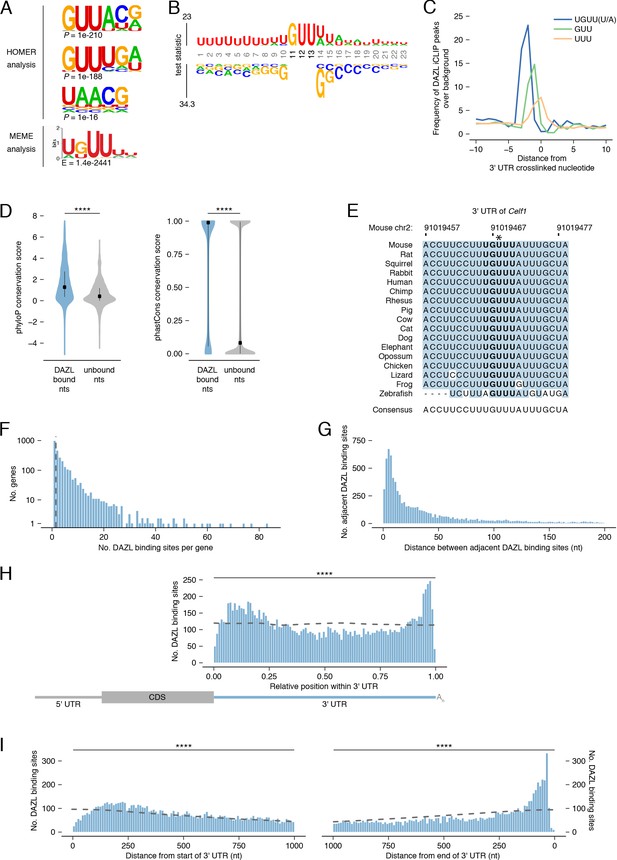
DAZL binds a UGUU(U/A) motif within 3' UTRs.
(A) De novo motif discovery from replicated DAZL peaks in 3' UTR exons. Motif analyses were carried out with HOMER and MEME tools using crosslinked peaks ± 10 nucleotides, with all expressed 3' UTRs (TPM ≥1) as background. The top three ranked motifs identified via HOMER are shown. One statistically significant motif was identified via MEME. (B) GUU-centered motif analysis of replicated peaks in 3' UTRs via kpLogo. For each crosslinked peak ±10 nucleotides, the closest GUU was identified, and all sequences were aligned along the GUU. Background was a subset of unbound GUUs randomly selected sequences from the full-length 3' UTRs that contain DAZL peaks. As P values are extremely small (<1×10−308), residues are scaled by test statistics. (C) Position of all UGUU(U/A), GUU, and UUU motifs relative to crosslinked nucleotides from replicated peaks in 3' UTRs. 0 represents the crosslinked nucleotide. Enrichment was identified relative to randomly selected sequences from the full-length 3' UTRs that contain DAZL peaks. (D) Conservation of DAZL binding sites across vertebrates based on phyloP and phastCons scores. DAZL-bound nucleotides identified via iCLIP were compared with unbound nucleotides from the same 3' UTRs (two-sided Mann-Whitney U test). (E) DAZL’s 3' UTR binding site in Celf1 is conserved among vertebrates. Blue shading highlights nucleotides that reflect the consensus. Bold designates DAZL’s UGUU(U/A) motif. Asterisk marks crosslinked nucleotide in DAZL iCLIP data. Sequence shown is absent from coelacanth. (F) Frequency of DAZL binding sites per DAZL-bound transcript. The majority of DAZL targets have more than one DAZL binding site (those targets to the right of the vertical dashed line). (G) Distance between adjacent DAZL binding sites in 1,649 DAZL-bound transcripts with more than one DAZL binding site. (H) Relative position of DAZL binding sites along the 3' UTR. The start and end of the 3' UTR were designated as 0 and 1, respectively. DAZL binding sites are enriched at the end and, to a lesser extent, at the start, relative to randomly selected sites in the same 3' UTRs (dashed line) (two-sided Kolmogorov-Smirnov test). (I) Absolute position of DAZL binding sites along the 3' UTR. DAZL binding sites exhibit a sharp accumulation 20–100 nucleotides from the end of the 3' UTR and a broader accumulation 100–240 nucleotides from the start of the 3' UTR relative to randomly selected positions within the same 3' UTRs (dashed line) (two-sided Kolmogorov-Smirnov tests). ****, p<0.0001.
-
Figure 4—source data 1
Characterization of DAZL binding within 3' UTR.
- https://cdn.elifesciences.org/articles/56523/elife-56523-fig4-data1-v2.xlsx
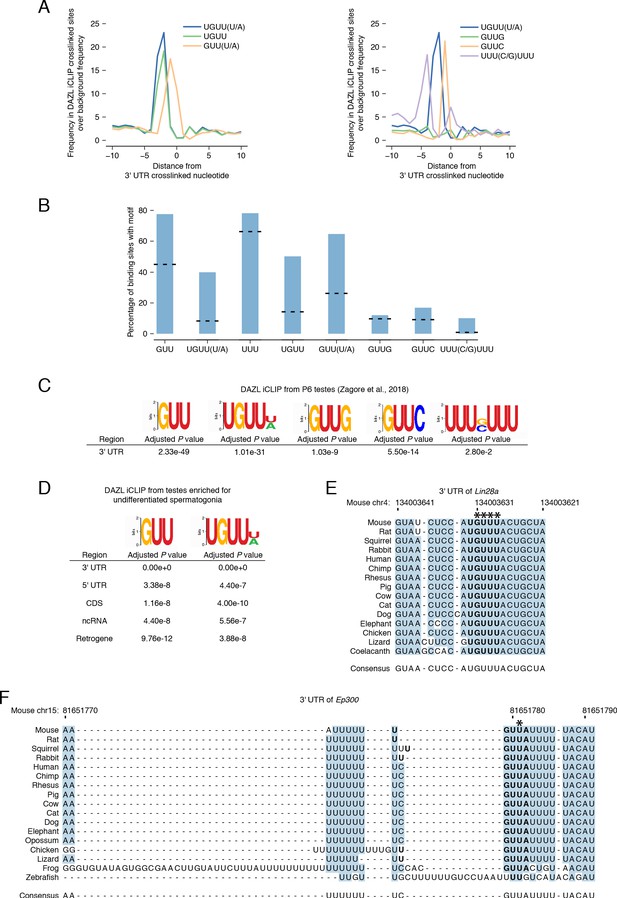
DAZL iCLIP motif enrichment and conservation.
(A) Position of UGUU(U/A) and other motifs relative to crosslinked nucleotides from replicated peaks in 3' UTRs. 0 represents the crosslinked nucleotide. Enrichment was identified relative to randomly selected sequences from the full-length 3' UTRs bound by DAZL. Left panel: UGUU and GUU(U/A) represent truncations of the UGUU(U/A) motif. UGUU was also previously identified as a DAZL motif in one study (Li et al., 2019). Right panel: previously reported DAZL motifs GUUG (Zagore et al., 2018), GUUC (Maegawa et al., 2002; Reynolds et al., 2005), and UUU(C/G)UUU (Chen et al., 2011). (B) Percentage of DAZL binding sites with GUU, UGUU(U/A) and other motifs. Dashed line for each motif represents expected percentage calculated using the nucleotide frequency within 3' UTRs of DAZL-bound transcripts. (C) Enrichment of specific motifs at replicated 3' UTR peaks from an independent DAZL iCLIP dataset from P6 testes (Zagore et al., 2018). Dataset was reanalyzed using our computational pipeline. AME from the MEME Suite (McLeay and Bailey, 2010) was used to identify the enrichment of each motif at crosslinked nucleotides in replicated peaks relative to shuffled control sequences. P value was adjusted with Bonferroni correction. (D) GUU and UGUU(U/A) motif enrichment at replicated peaks from expressed transcripts (TPM ≥1) from each type of genomic region. Analysis was carried out as described in C. P value was adjusted with Bonferroni correction to account for multiple testing. (E) DAZL’s 3' UTR binding sites in Lin28a are conserved among vertebrates. Blue highlights nucleotides conserved across all sequences. Bold designates DAZL’s UGUU(U/A) motif. Asterisks mark crosslinked nucleotides in DAZL iCLIP data. Lin28a sequence is absent from opossum, frog, and zebrafish. (F) DAZL’s 3' UTR binding sites in Ep300 are conserved among vertebrates. Formatting as described in D. Ep300 sequence is absent from coelacanth.
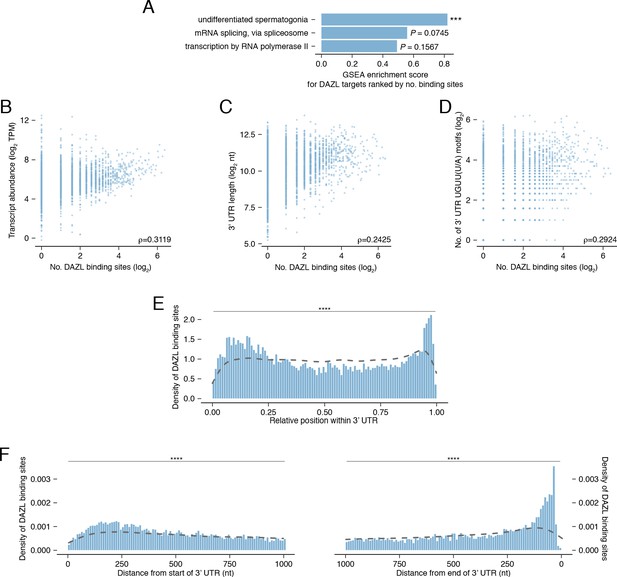
DAZL binding along the 3' UTR.
(A) Gene Set Enrichment Analysis (GSEA) enrichment scores showing the degree to which gene sets are overrepresented among DAZL targets with more binding sites, relative to all targets. The gene set for ‘undifferentiated spermatogonia’ is listed in Figure 2—source data 1. The gene sets ‘mRNA splicing, via spliceosome’ and ‘transcription by RNA polymerase II’ are from Gene Ontology (GO) terms. Adjusted P values shown. (B) Relationship between number of DAZL binding sites and transcript abundance. (C) Relationship between number of DAZL binding sites and 3' UTR length. (D) Relationship between number of DAZL binding sites and number of UGUU(U/A) motifs in the 3' UTR. (E) Relative positions of DAZL binding site density along the 3' UTR. The density of DAZL binding sites was statistically distinct from the density of all UGUU(U/A) motifs within DAZL-bound 3' UTRs (dashed line) (two-sided Kolmogorov-Smirnov test). (F) Absolute position of DAZL binding site density along the 3' UTR. The densities of DAZL binding sites were statistically distinct from the densities of all UGUU(U/A) motifs within DAZL-bound 3' UTRs (dashed lines) (two-sided Kolmogorov-Smirnov tests). ***, p<0.001, ****, p<0.0001.
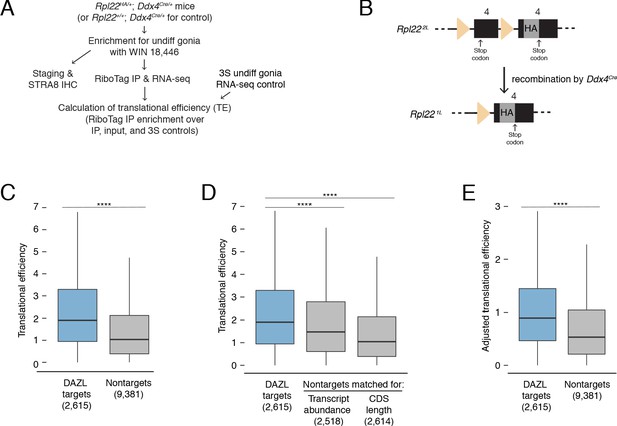
DAZL enhances translation of its targets in undifferentiated spermatogonia.
(A) Schematic of synchronization of spermatogenesis by WIN 18,446 to enrich for undifferentiated spermatogonia for RiboTag IP and sequencing experiments. (B) Schematic of the RiboTag allele. The Rpl22 locus carries a floxed exon 4, which is expressed in the absence of recombination, followed by an engineered exon four that encodes an HA tag before the stop codon. Recombination via the Ddx4-Cre allele removes the floxed exon four and allows for expression of the exon four that encodes the HA tag specifically in germ cells. The germ cells’ ribosomes can then be immunoprecipitated via the HA tag. (C) Translational efficiency of DAZL targets compared with all nontargets from undifferentiated spermatogonia (two-sided Mann-Whitney U test). (D) Translational efficiency of DAZL targets compared with nontarget subset datasets that were sampled to match transcript abundance (TPM) and CDS length in undifferentiated spermatogonia (two-sided Mann-Whitney U test). (E) Adjusted translational efficiency in undifferentiated spermatogonia (two-sided Mann-Whitney U test), calculated from a multiple log-linear regression model that included transcript abundance (TPM), CDS length, 3' UTR length, codon usage (Codon Adaptiveness Index, CAI), and DAZL binding as variables. Adjusted translational efficiency was calculated by subtracting the contributions (as calculated by the model) of transcript abundance, CDS length, 3' UTR length, and codon usage from biochemically measured translational efficiency values. ****, p<0.0001.
-
Figure 5—source data 1
Translational efficiencies for genes expressed in undifferentiated spermatogonia.
- https://cdn.elifesciences.org/articles/56523/elife-56523-fig5-data1-v2.xlsx
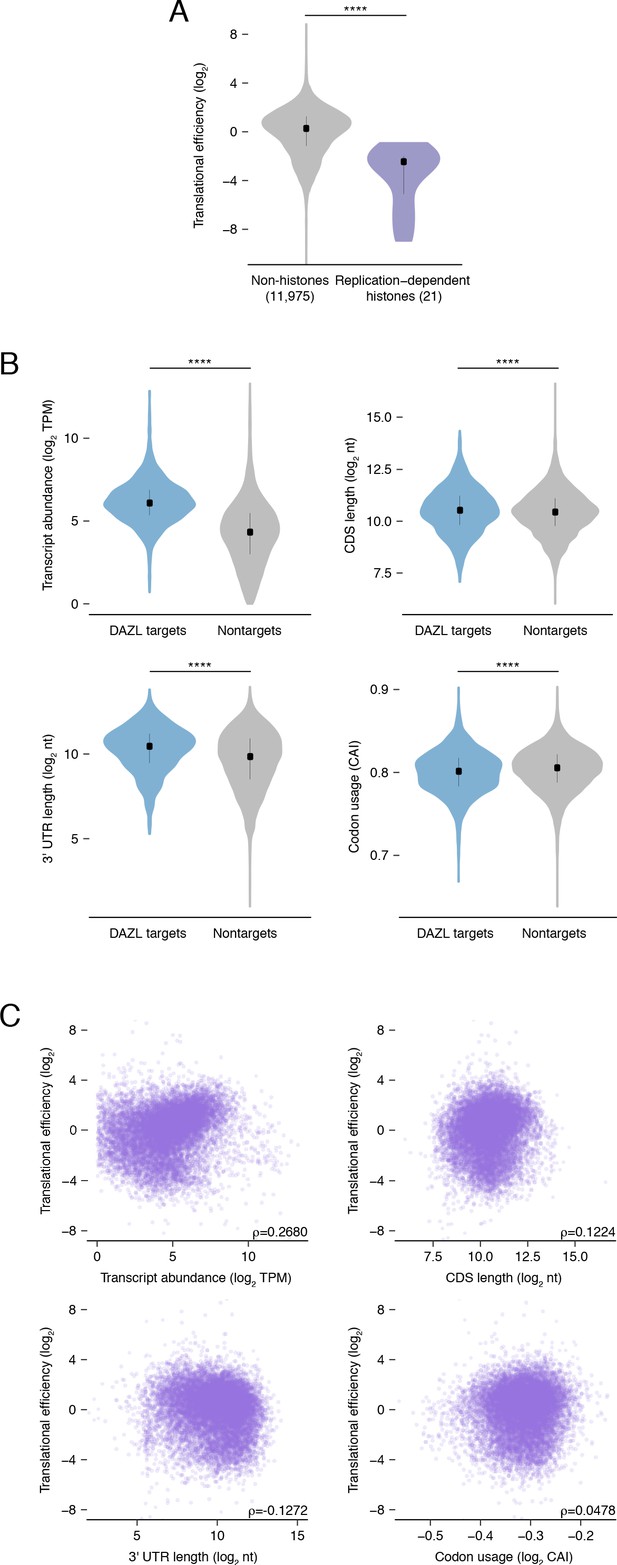
Translational efficiency in undifferentiated spermatogonia.
(A) Replication-dependent histones show reduced translational efficiency compared with the average transcript in undifferentiated spermatogonia. This is expected, as the undifferentiated spermatogonia here were not synchronized for S phase, when these histones are robustly translated (two-sided Mann-Whitney U test). (B) DAZL targets are distinct from nontargets in variables known to correlate with translational efficiency: transcript abundance, CDS length, 3' UTR length, and codon usage (CAI) (two-sided Kolmogorov-Smirnov tests). (C) Relationships between translational efficiency and variables known to correlate with translation efficiency: transcript abundance, CDS length, 3' UTR length, and codon usage. ****, p<0.0001.
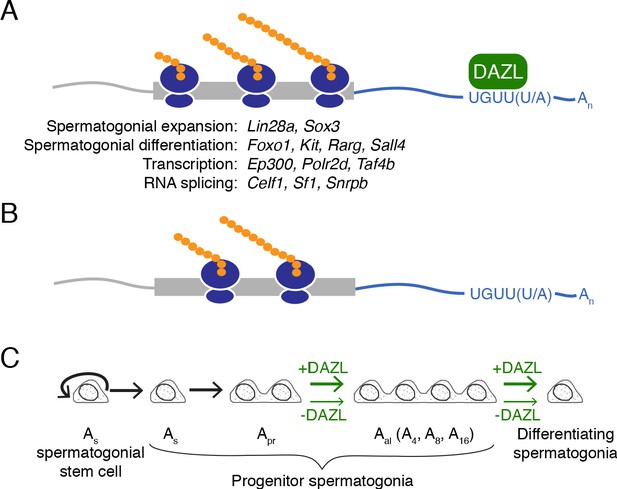
Model of DAZL’s regulation of a broad translation program in undifferentiated spermatogonia.
(A) DAZL promotes robust translation of a broad set of transcripts, ranging from those required for spermatogonial proliferation and differentiation to those that regulate fundamental cellular processes like transcription and RNA splicing. (B) In the absence of DAZL, transcripts normally bound by DAZL are translated less efficiently. (C) DAZL’s broad translational program promotes the expansion of late progenitor (Aal) undifferentiated spermatogonia and subsequent spermatogonial differentiation (‘+DAZL’ arrows). In the absence of DAZL (‘-DAZL’ arrows), both expansion and differentiation occur at reduced rates.
Tables
Reagent type (species) or resource | Designation | Source or reference | Identifiers | Additional information |
---|---|---|---|---|
Gene (Mus musculus) | deleted in azoospermia-like (Dazl) | Mouse Genome Informatics | MGI: 1342328 | |
Strain, strain background (Mus musculus) | B6 | Taconic | TAC: B6-F TAC: B6-M | C57BL/6NTac |
Genetic reagent (Mus musculus) | Dazl:tdTomato | Nicholls et al., 2019b | B6D2-Dazlem1(tdTomato)Huyc | |
Genetic reagent (Mus musculus) | Ddx4Cre | Hu et al., 2013 | MGI:5554579 | Ddx4tm1.1(cre/mOrange)Dcp |
Genetic reagent (Mus musculus) | Dazl2L | Nicholls et al., 2019b | B6N-Dazlem1Dcp | |
Genetic reagent (Mus musculus) | Dazl- | Ruggiu et al., 1997 | RRID:IMSR_JAX:023802 | 129P2-Dazltm1Hjc |
Genetic reagent (Mus musculus) | Dazl1L | Nicholls et al., 2019b | B6N-Dazlem1.1Dcp | |
Genetic reagent (Mus musculus) | Pou5f1:EGFP | Szabó et al., 2002 | RRID:IMSR_JAX:004654 | Tg(Pou5f1-EGFP)2Mnn |
Genetic reagent (Mus musculus) | ROSA26tdTomato | Madisen et al., 2010 | RRID:IMSR_JAX:007909 | Gt(ROSA)26Sortm9(CAG-tdTomato)Hze |
Genetic reagent (Mus musculus) | Rpl22HA | Sanz et al., 2009 | RRID:IMSR_JAX:011029 | RiboTag allele; B6N.129-Rpl22tm1.1Psam/J |
Antibody | anti-DAZL (rabbit polyclonal) | Abcam | Abcam ab34139; RRID:AB_731849 | IHC 1:200; iCLIP 10 μg antibody per 100 μl Dynabeads and 500 μl lysate |
Antibody | anti-DAZL (mouse monoclonal IgG1) | BioRad | BioRad MCA2336; RRID:AB_2292585 | IF 1:100 |
Antibody | anti-FOXC2 (sheep polyclonal) | R&D Systems | R&D Systems AF6989; RRID:AB_10973139 | IF 1:250 |
Antibody | anti-HA (mouse monoclonal IgG1) | BioLegend | BioLegend #901513; previously Covance #MMS-101R; RRID:AB_2565335 | IP 2.5 μl antibody per 400 μl lysate and 200 μl Dynabeads |
Antibody | anti-KIT (goat polyclonal) | R&D Systems | R&D Systems AF1356; RRID:AB_354750 | IF 1:250 |
Antibody | anti-mCherry (goat polyclonal) | SICGEN | SICGEN AB0040-200; RRID:AB_2333092 | IF 1:300 |
Antibody | anti-SOX9 (rabbit polyclona) | EMD Millipore | EMD Millipore AB5535; RRID:AB_2239761 | IF 1:300 for postnatal testis sections; 1:250 for adult testis sections |
Antibody | anti-STRA8 (rabbit polyclonal) | Abcam | Abcam ab49405; RRID:AB_945677 | IHC 1:500 |
Antibody | anti-ZBTB16 (goat polyclonal) | R&D Systems | R&D Systems AF2944; RRID:AB_2218943 | IF 1:250 |
Antibody | IgG control, anti-rabbit polyclonal | Santa Cruz Biotechnologies | Santa Cruz Biotechnologies sc-2027; RRID:AB_737197 | iCLIP 10 μg antibody per 100 μl Dynabeads and 500 μl lysate |
Commercial assay or kit | ImmPACT DAB Peroxidase Substrate | Vector Laboratories | Vector Laboratories SK-4105 | |
Commercial assay or kit | ImmPRESS HRP anti-Rabbit Detection Kit | Vector Laboratories | Vector Laboratories MP-7401–50 | |
Commercial assay or kit | TruSeq Stranded mRNA kit | Illumina | Illumina 20020594 | |
Commercial assay or kit | SMART-Seq v4 Ultra Low Input RNA Kit | Takara Bio | Takara Bio 634888 | |
Commercial assay or kit | SMARTer Stranded Total RNA-Seq Kit v2 – Pico Input | Takara Bio | Takara Bio 634411 | |
Chemical compound, drug | N,N′-Octamethylenebis(2,2-dichloroacetamide) [Win18,446] | Santa Cruz Biotechnology | Santa Cruz Biotechnologies sc-295819 | Used at 0.1 mg/gram body weight |
Software, algorithm | ASPeak v.2.0.0 | Kucukural et al., 2013 | RRID:SCR_000380 | https://sourceforge.net/projects/as-peak |
Software, algorithm | CellProfiler v3.1.8 | Kamentsky et al., 2011 | RRID:SCR_007358 | https://cellprofiler.org |
Software, algorithm | coRdon v1.2.0 | https://github.com/BioinfoHR/coRdon | ||
Software, algorithm | Cutadapt v.1.8 | Martin, 2011 | RRID:SCR_011841 | https://cutadapt.readthedocs.io/en/stable/ |
Software, algorithm | DESeq2 v1.26.0 | Love et al., 2014 | RRID:SCR_015687 | http://bioconductor.org/packages/release/bioc/html/DESeq2.html |
Software, algorithm | FASTX-Toolkit v.0.0.14 | RRID:SCR_005534 | http://hannonlab.cshl.edu/fastx_toolkit/index.html | |
Software, algorithm | HOMER v4.9.1 | Heinz et al., 2010 | RRID:SCR_010881 | http://homer.ucsd.edu/homer/ |
Software, algorithm | GSEA v4.0.3 | Mootha et al., 2003; Subramanian et al., 2005 | RRID:SCR_003199 | https://www.gsea-msigdb.org/gsea/index.jsp |
Software, algorithm | kallisto v0.44.0 | Bray et al., 2016 | RRID:SCR_016582 | https://pachterlab.github.io/kallisto/ |
Software, algorithm | kpLogo v1.0 | Wu and Bartel, 2017 | http://kplogo.wi.mit.edu | |
Software, algorithm | MEME Suite v4.11.2 | Bailey and Elkan, 1994; McLeay and Bailey, 2010 | RRID:SCR_001783 | http://meme-suite.org/ |
Software, algorithm | MetaPlotR | Olarerin-George and Jaffrey, 2017 | https://github.com/olarerin/metaPlotR | |
Software, algorithm | PANTHER v.13.1 | Mi et al., 2017 | RRID:SCR_004869 | http://www.pantherdb.org |
Software, algorithm | STAR v.2.5.4b | Dobin et al., 2013 | RRID:SCR_015899 | https://github.com/alexdobin/STAR |
Software, algorithm | TargetScanMouse v7.1 | Agarwal et al., 2015 | RRID:SCR_010845 | http://www.targetscan.org/mmu_72/ |
Other | Collagenase, Type I | Worthington Biochemical | Worthington Biochemical LS004196 | |
Other | Dynabeads Protein G | Thermo Fisher Scientific | Thermo Fisher Scientific 10003D | |
Other | GlycoBlue Coprecipitant | Thermo Fisher Scientific | Thermo Fisher Scientific AM9515 | |
Other | Novex TBE-Urea gel, 6% | Thermo Fisher Scientific | Thermo Fisher Scientific EC6865BOX | |
Other | Protease inhibitor, EDTA Free | MilliporeSigma | MilliporeSigma 11836170001 | |
Other | Proteinase K, RNA grade | Thermo Fisher Scientific | Thermo Fisher Scientific 25530049 | |
Other | RNase I | Thermo Fisher Scientific | Thermo Fisher Scientific AM2295 | For DAZL iCLIP libraries, lysates were digested with 0.005 U/μg RNase I for 3 min at 37 °C and 1100 rpm on a Thermomixer R (Eppendorf) |
Other | TURBO DNAse | Thermo Fisher Scientific | Thermo Fisher Scientific AM2238 |
Additional files
-
Supplementary file 1
Information on statistical tests, alternative hypotheses, and P values used.
- https://cdn.elifesciences.org/articles/56523/elife-56523-supp1-v2.xlsx
-
Transparent reporting form
- https://cdn.elifesciences.org/articles/56523/elife-56523-transrepform-v2.docx