Antagonistic roles for Ataxin-2 structured and disordered domains in RNP condensation
Figures
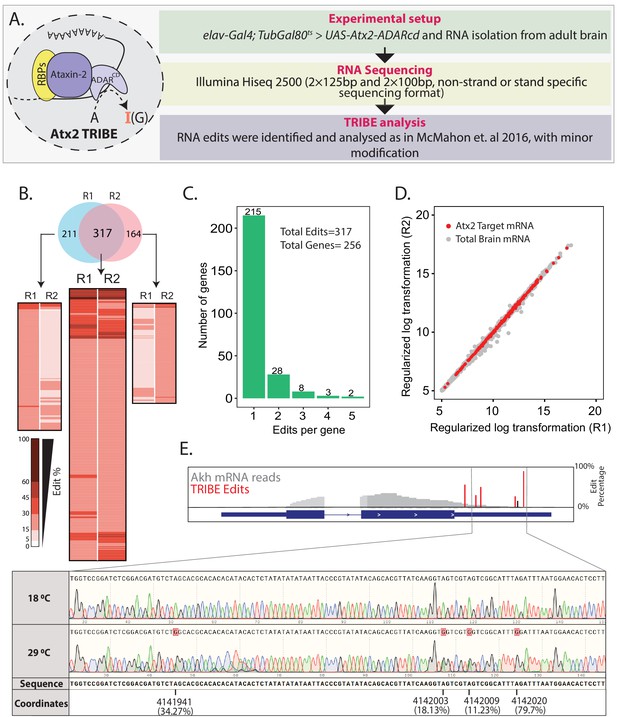
Using Targets of RNA-Binding Proteins Identified by Editing (TRIBE) to identify Atx2-interacting mRNAs in the adult fly brain.
(A) Schematic and flow chart for TRIBE analysis: the Atx2-ADARcd fusion protein is expressed in the adult fly brain, total brain mRNA isolated, sequenced, and analyzed using a published TRIBE pipeline. (B) Heatmaps show edit percentages of individual transcript coordinates. Replicate experiments R1 and R2 identify largely overlapping edit sites and edited mRNAs. The common targets (intersect between R1 and R2) show almost reproducible edit levels. The inset (heatmaps on either sides of the common intersected list) indicates that several mRNAs are identified as ‘non-replicates’ between R1 or R2 because they do not cross quality control thresholds (edit percentages or read counts) and not because of robust differences between replicates. (C) Bar plot showing the number of edits with a significant number of genes edited at a single site. (D) The mRNA edits are independent of expression levels. A scatter plot shows expression differences of all the mRNAs expressed in fly brain. Red dots represent the edited mRNAs while gray dots represent the mRNAs of the brain transcriptome that are not edited. (E) Sanger sequencing confirms editing site identified by TRIBE analysis. Data shown for one target mRNA (Akh). Red bars show the edit percentages at the different modified nucleotides with respect to the total Akh mRNA shown in gray. The black bar indicates identified edits that are below 15% threshold (see also Figure 1—figure supplement 4).
-
Figure 1—source data 1
Data related to Figure 1B.
Percentage edits for Atx2-target mRNAs in RNAseq replicates.
- https://cdn.elifesciences.org/articles/60326/elife-60326-fig1-data1-v1.xlsx
-
Figure 1—source data 2
Data related to Figure 1C.
Atx2-target mRNAs along with the number of edits.
- https://cdn.elifesciences.org/articles/60326/elife-60326-fig1-data2-v1.xlsx
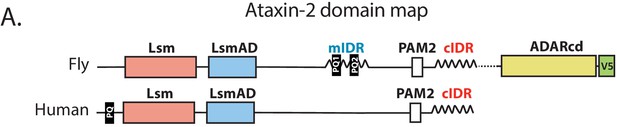
Atx2-ADARcd fusion protein constructs.
Schematic showing the Atx2-ADARcd construct.
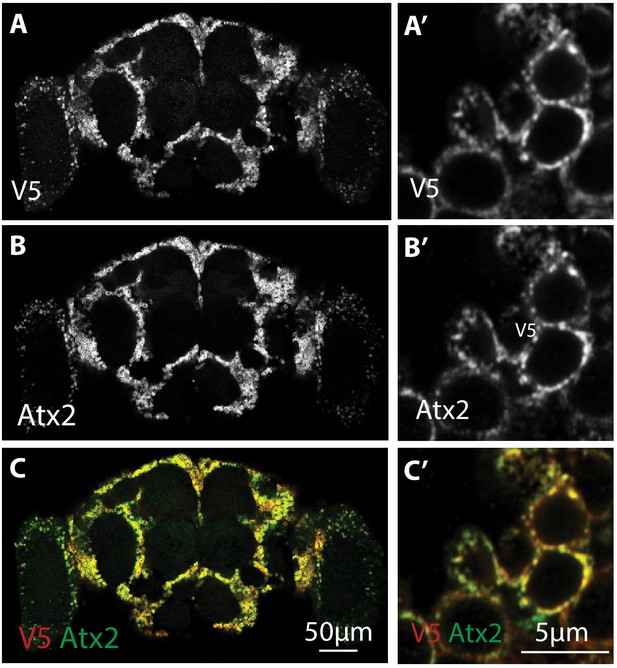
UAS-Atx2-ADARcd expression was driven using elav-Gal4;tub-Gal80ts in adult fly brain for 5 days.
Brains dissected from flies maintained at 29℃ for 5 days were stained using anti-V5 (A, A') and anti-Atx2 (B, B') antibodies. (A-C') Confocal images of Oblique frontal view of the Drosophila brain. (A,B,C) (dorsal is more anterior) of a complete. (A',B'C') higher magnification of the respective overview images.
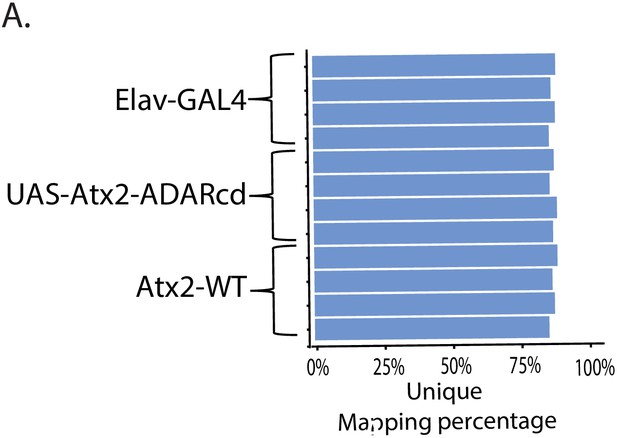
Unique mapping percentage for various RNAseq replicates.
Total RNA was isolated from 10 to 12 brains for each replicate. Poly(A)-enriched mRNA was sequenced using paired-end libraries on Illumina 2500. The reads obtained were of high quality, and mapping to Drosophila melanogaster genome was >80% for all the samples.
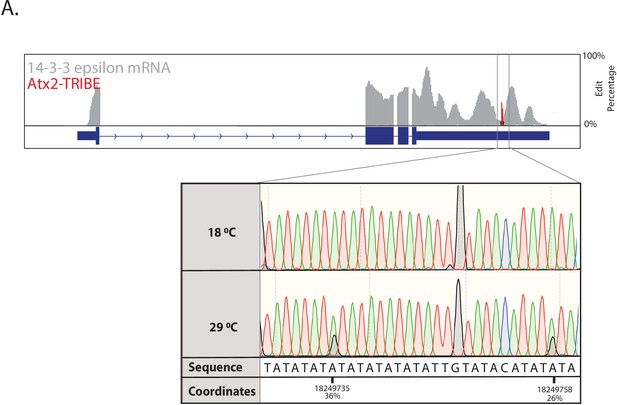
An example of a gene, 14-3-3 epsilon, showing multiple edits specifically in the exons.
Red lines show the edit percentages at the different modified nucleotides with respect to the total mRNA present at a given moment of time.
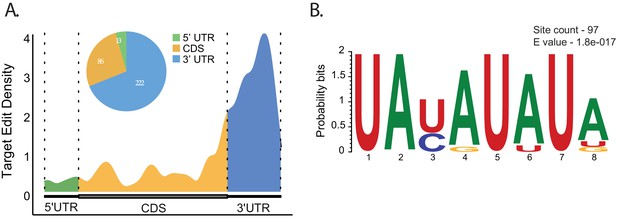
Atx2 preferentially edits AU-rich sequences in the 3′UTRs of the target mRNAs.
(A) Metaplot analysis showing Atx2 preferentially associates with 3′UTRs of the target mRNA. (B) Motif analyses involving ±100 bases around the edit site using MEME identifies AU-rich element sequences in the 3′UTRs of the target mRNAs.
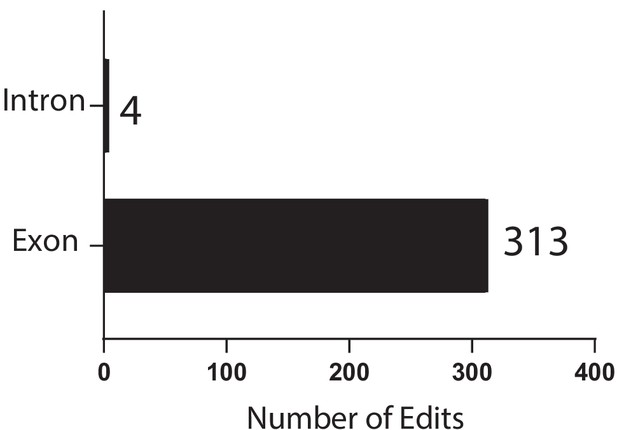
Exon specificity of Atx2 mRNA interactions and sequence selectivity of observed Atx2–coding sequence (CDS) interactions.
Atx2-ADARcd fusion protein exclusively edits exons, indicating cytoplasmic binding to mature mRNAs.
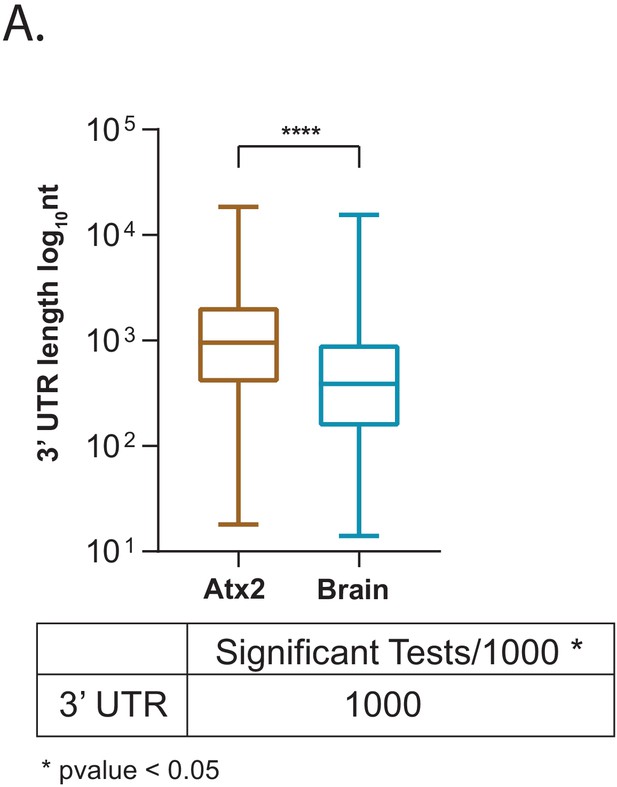
Atx2-target mRNAs have longer-than-average 3′UTRs.
Mann–Whitney U test was used to determine the difference in the 3′UTR length between Atx2 targets and brain transcriptome. p-Value is <0.0001, and error bars represent SEM. Bootstraping was performed 1000 times with replacement. Statistics was performed using chi-square test with cutoff (p-value <0.05). Fisher's test was used to combine the p-values.
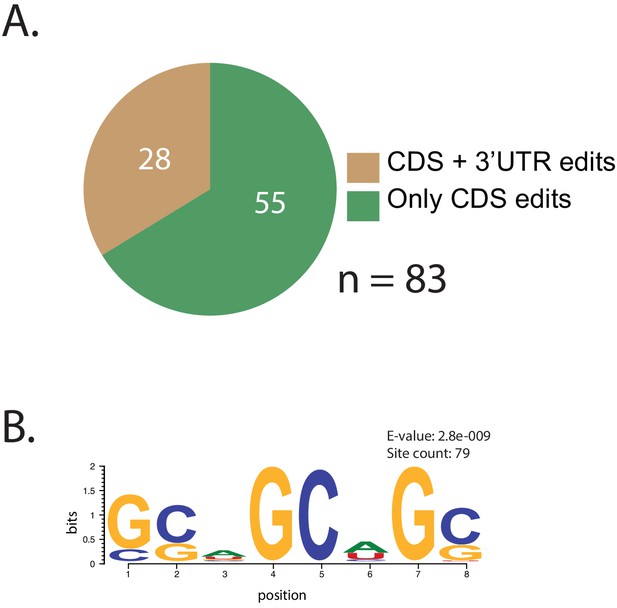
Coding sequence edits were co-existent with 3'UTR edits.
(A) Coding sequence edits often occurred together with a 3′UTR edit on the same mRNA consistent with an initial Atx2–3′UTR interaction enabling edits to a proximal coding sequence as well. (B) A GC-rich motif for Atx2–CDS association indicated by analyses of sequences flanking CDS edit sites.
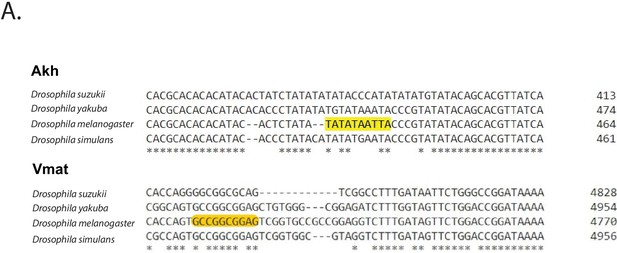
Multiple-sequence alignment was performed for regions around Atx2 binding motifs in Akh and Vmat genes between Drosophila melanogaster and closely related Drosophila species.
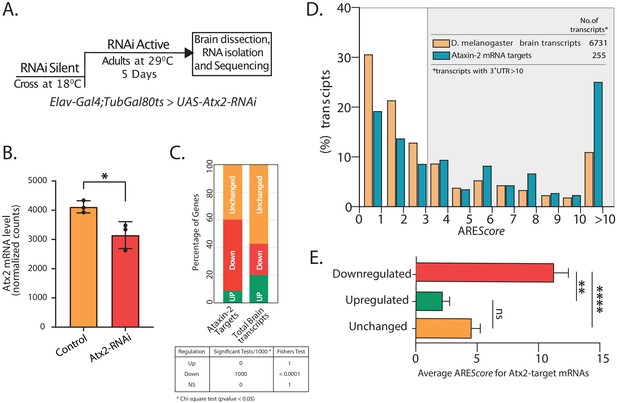
Atx2–ARE interactions modulate mRNA stability.
(A) Schematic of strategy to induce RNAi expression specifically in adult fly brain. Total RNA extracted from brain post 5 days at 29°C was sequenced using Illumina 2500 and differential expression analyzed using DESeq2. (B) Normalized mRNA read counts showing Atx2 mRNA levels to be significantly reduced following RNAi expression compared to Gal4 control with a p-value of <0.0298 using Student’s t-test. (C) Effect on Atx2-target mRNAs following Atx2 knockdown: the majority are reduced in level, indicating a role for Atx2 is target stabilization. Bootstraping was performed 1000 times with replacement. Statistics was performed using chi-square test with cutoff (p-value <0.05). Fisher’s test was used to combine the p-values. (D) AREScore analysis showing AU-rich elements (AREs) to be enriched in Atx2-target mRNAs compared to the brain transcriptome. (E) Among Atx2-target mRNAs, higher ARE scores are seen in mRNAs whose levels are reduced following Atx2 knockdown.
-
Figure 3—source data 1
Data related to Figure 3C.
Fold changes in Atx2-target mRNAs upon Atx2 RNAi.
- https://cdn.elifesciences.org/articles/60326/elife-60326-fig3-data1-v1.xlsx
-
Figure 3—source data 2
Data related to Figure 3E.
Correlation of AREScore and target mRNA levels.
- https://cdn.elifesciences.org/articles/60326/elife-60326-fig3-data2-v1.xlsx
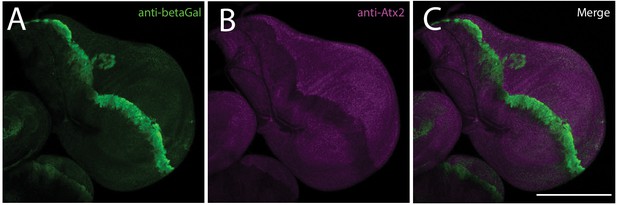
Atx2 RNAi knockdown reduces Atx2 expressions.
Ptc-Gal4 was used to drive Atx2 RNAi in Drosophila wing disc and immunostained with anti-beta-Gal (A) and anti-Atx2 (B) antibodies. Atx2 levels are reduced in the Gal4-expressing cells (C).
-
Figure 3—figure supplement 1—source data 1
Data related to RNAseq mapping percentage from Gal4 and Atx2 RNAi brains.
- https://cdn.elifesciences.org/articles/60326/elife-60326-fig3-figsupp1-data1-v1.xlsx
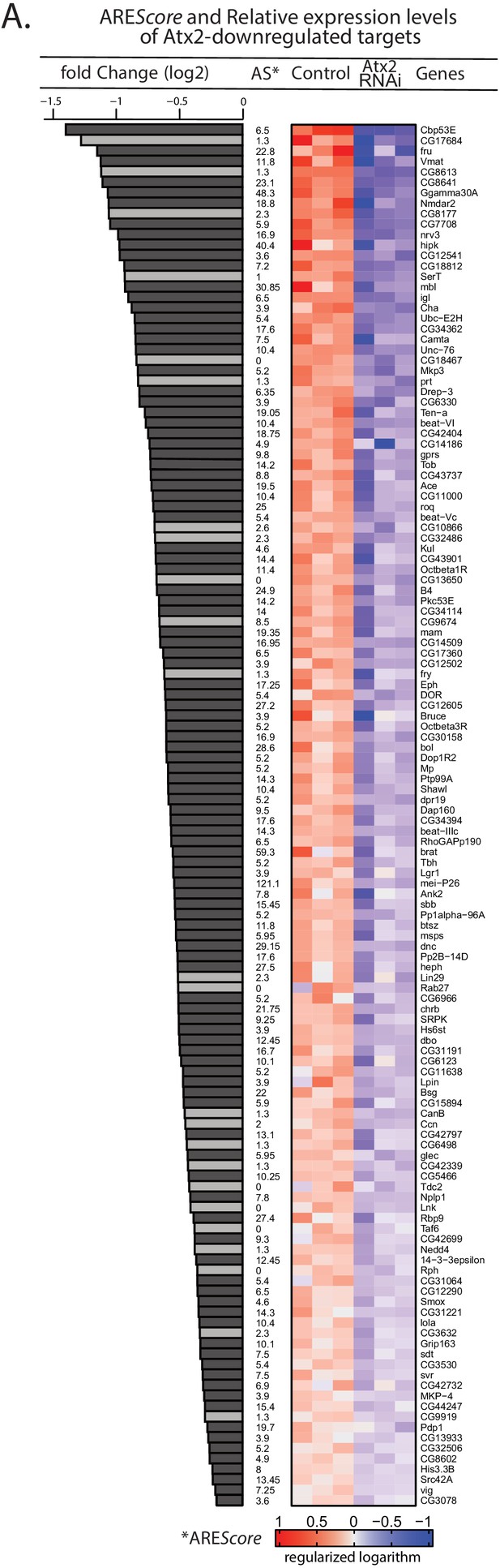
Correlation between AREScore and relative expression levels of Atx2-downregulated targets for three biological replicates (selected based on a p-value<0.05).
Heatmap indicates relative expression levels for each mRNA in control and experimental brain samples. Light gray bars represent Atx2-target mRNAs without significant AREScore.
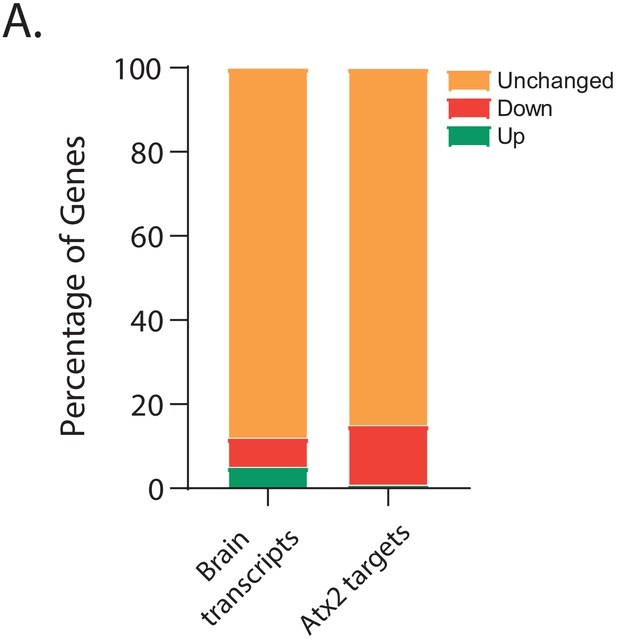
Differential expression analysis of intron reads from control and Atx2 RNAi show no major reduction in levels of Atx2 Targets of RNA-Binding Proteins Identified by Editing nascent mRNA targets.
-
Figure 3—figure supplement 3—source data 1
Fold changes in the introns of Atx2-target mRNAs upon Atx2 RNAi.
- https://cdn.elifesciences.org/articles/60326/elife-60326-fig3-figsupp3-data1-v1.xlsx
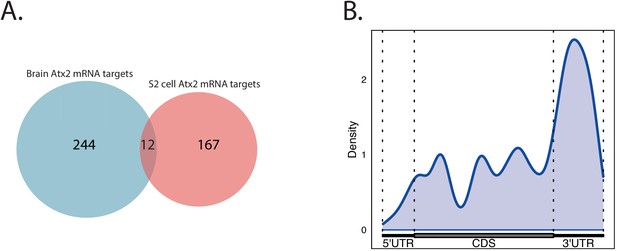
Comparative analyses of Atx2 targets in Drosophila brain and S2 cells.
(A) Comparison of Atx2 S2 cell Targets of RNA-Binding Proteins Identified by Editing (TRIBE) targets with Drosophila brain TRIBE targets. (B) Metaplot showing Atx2 preferentially associates with 3′UTRs of target mRNAs in S2 cells.
-
Figure 3—figure supplement 4—source data 1
Percentage edits of Atx2-target mRNAs in S2 cells among RNAseq replicates.
- https://cdn.elifesciences.org/articles/60326/elife-60326-fig3-figsupp4-data1-v1.xlsx
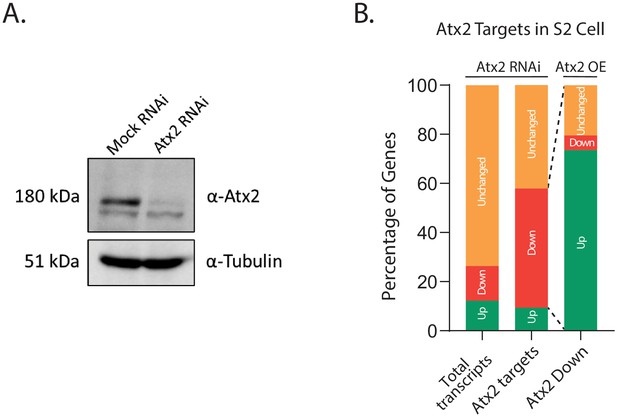
Altering levels of Atx2 in S2 cells affects the levels of target mRNAs.
(A) Knockdown of Atx2 using dsRNA in S2 cells. Western blots with anti-Atx2 antibody and anti-tubulin show significant reduction in Atx2 levels. (B) Knockdown of Atx2 in S2 cells destabilizes majority of its Targets of RNA-Binding Proteins Identified by Editing targets, which are stabilized in overexpression, indicating a role for Atx2 is target stabilization.
-
Figure 3—figure supplement 5—source data 1
Fold changes in Atx2-target mRNAs when Atx2 is overexpressed or knocked down in S2 cells.
- https://cdn.elifesciences.org/articles/60326/elife-60326-fig3-figsupp5-data1-v1.xlsx
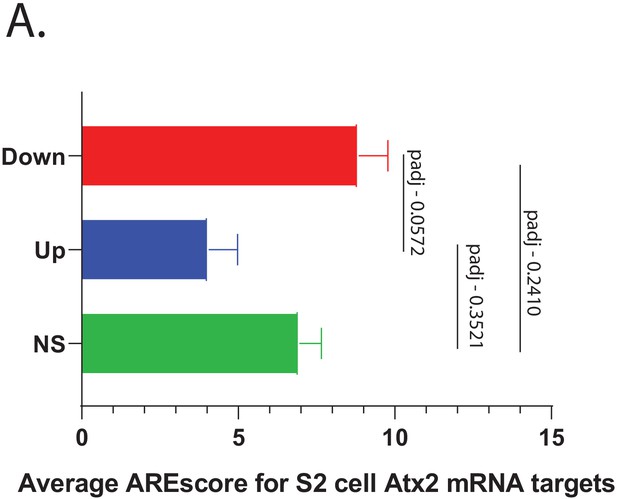
AREScore correlate directly with the stability of Atx2-target mRNAs in S2 cells.
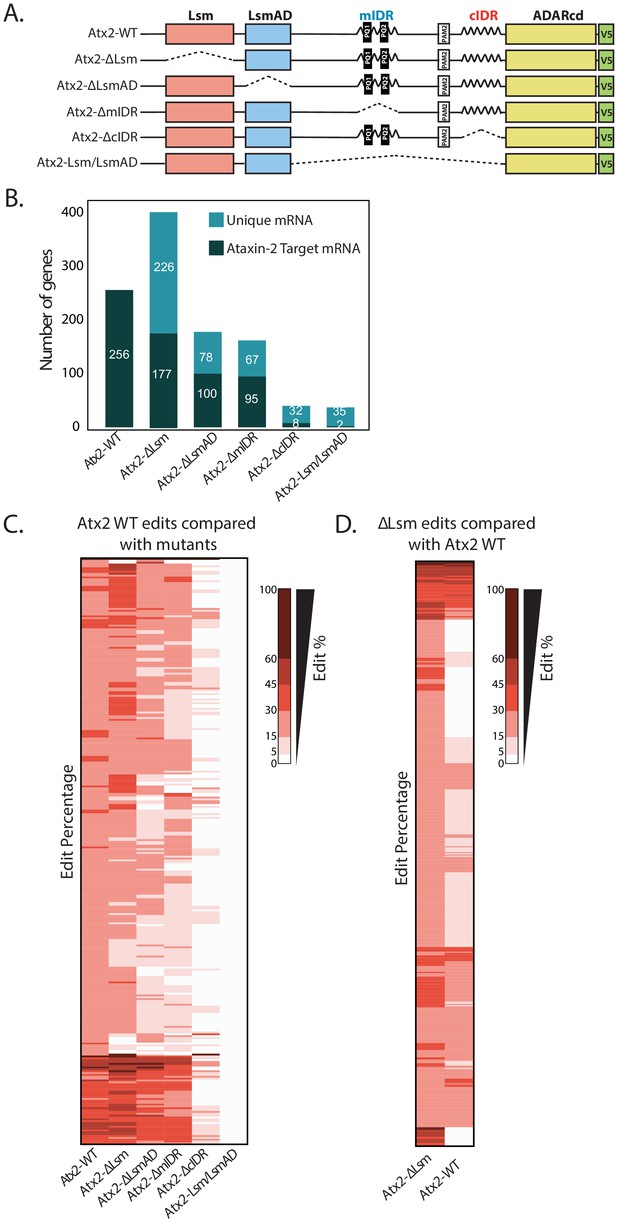
Atx2 requires its cIDR domain for interaction with its target mRNA.
(A) An illustration of domains of Atx2. The structured (Like-Sm [Lsm] and Lsm-associated [LsmAD]) and disordered domains (mIDR and cIDR) of Atx2 protein were deleted one at a time to understand the domains necessary for its interaction with target RNA. The wild-type Atx2 and the deletions contained a c-terminal ADARcd and V5 tag. The deletions are shown by the dotted lines. (B) Deletion of LsmAD or mIDR domain reduced the ability of Atx2 to interact with its targets, while cIDR deletion almost entirely prevented Atx2 mRNA interactions. The Lsm-domain deletion showed an overall increase in mRNA edits. (C) More detailed heatmap view of individual target mRNAs showing target edit percentages in flies expressing control or domain-deleted forms Atx2 fused to ADARcd. (D) Most of the apparently new targets identified in Atx2∆Lsm Targets of RNA-Binding Proteins Identified by Editing analyses are also edited, albeit at a lower efficiency, in flies expressing full-length Atx2-ADAR fusions. This suggests that deletion of the Lsm domain increases the interaction of Atx2 with its native target mRNAs. (For C and D, 15% threshold edits identified for samples in column 1 [Atx2 WT in C and Atx2∆Lsm in D] was used to compare with all the edits of the rest of the columns to generate a heatmap.)
-
Figure 4—source data 1
Data related to Figure 4B.
mRNA targets identified for different Atx2 domain deletions.
- https://cdn.elifesciences.org/articles/60326/elife-60326-fig4-data1-v1.xlsx
-
Figure 4—source data 2
Data related to Figure 4C, D.
Edit percentages for Atx2-target mRNAs identified using different domain deletions.
- https://cdn.elifesciences.org/articles/60326/elife-60326-fig4-data2-v1.xlsx
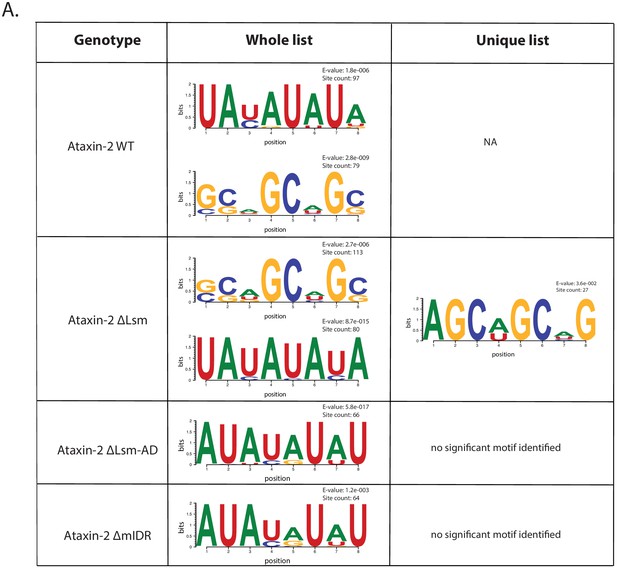
Atx2 Like-Sm (Lsm), Lsm-associated (LsmAD), or mIDR domains are not essential for AU-rich motif preference.
MEME analysis showing Atx2 preferentially associates with AU-rich motif on target mRNA. Deletion of Lsm, LsmAD, or mIDR domains does not block the Atx2 AU-rich motif preference. Unique targets found in Lsm domain deletion have a GC-rich motif preference, which is also identified as the second most predominant motif that Atx2 WT bind.
-
Figure 4—figure supplement 1—source data 1
Mapping percentage for different Atx2 deletions.
- https://cdn.elifesciences.org/articles/60326/elife-60326-fig4-figsupp1-data1-v1.xlsx
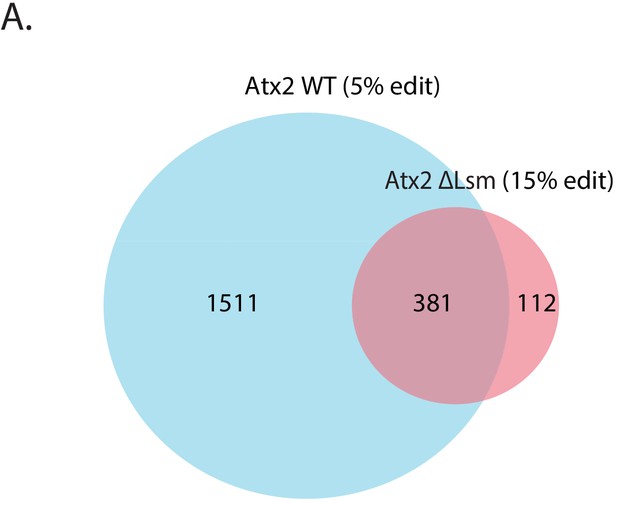
Atx2∆Lsm unique targets were present at a reduced edit threshold in Atx2 WT.
Several of the Atx2∆Lsm edits found at 15% threshold were found in Atx2 WT edits at 5% threshold.
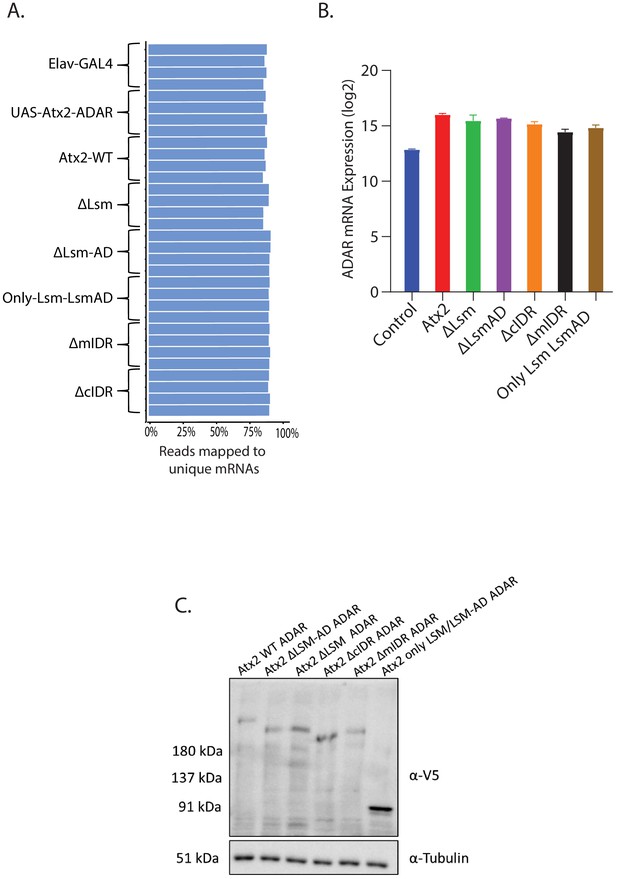
Equivalent read quality and ADAR expression across Atx2 deletion analyses.
Poly(A)-enriched mRNA from 10 to 12 brains was sequenced using paired-end libraries on Illumina 2500. (A) The reads obtained were comparable between genotypes and of high quality, and mapping to the Drosophila genome was >80% for all the samples (Figure 4—figure supplement 1—source data 1). (B) Quantification for ADAR mRNA as a read out for transgene expression showed expression above the endogenous control and comparable between Atx2 WT and the various mutant transgenic lines. (C) Western blots with anti-V5 antibody show levels of Atx2 transgene expression in Drosophila brain among transgenic lines.
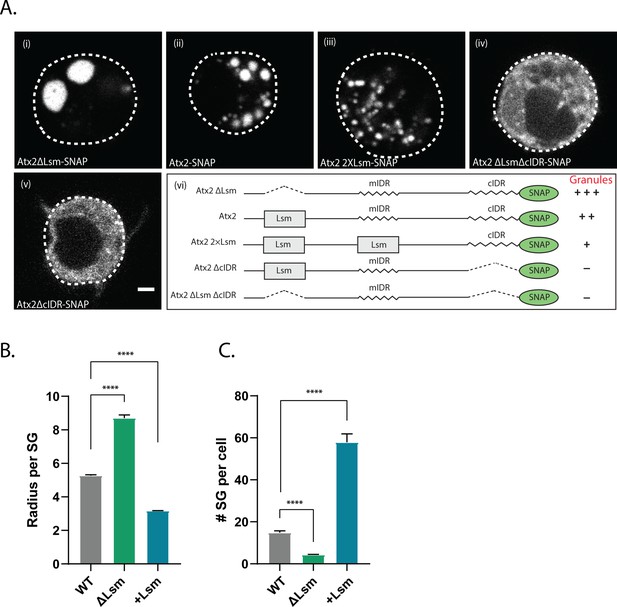
Atx2 Like-Sm (Lsm) domain alters granule dynamics.
(A) Drosophila S2 cells expressing Atx2 protein with a C-terminal SNAP tag. Atx2∆Lsm form large cytoplasmic granules (i) that are much larger than WT Atx2 granules (ii). Atx2 with an additional Lsm domain in place of mIDR to create 2XLsm forms smaller granules compared to WT Atx2 in S2 cells (iii). Deletion of cIDR domain blocks Atx2 granule formation (iv). In the absence of cIDR, Lsm deletion does not rescue Atx2 granules (v). Domain map along with the granule phenotype is shown in (vi). The scale bar corresponds to 2 µm. (B) Radius of the stress granule (size) and (C) number of stress granules per cell were quantified and plotted. More than 80 cells per genotype were used for granule quantification.
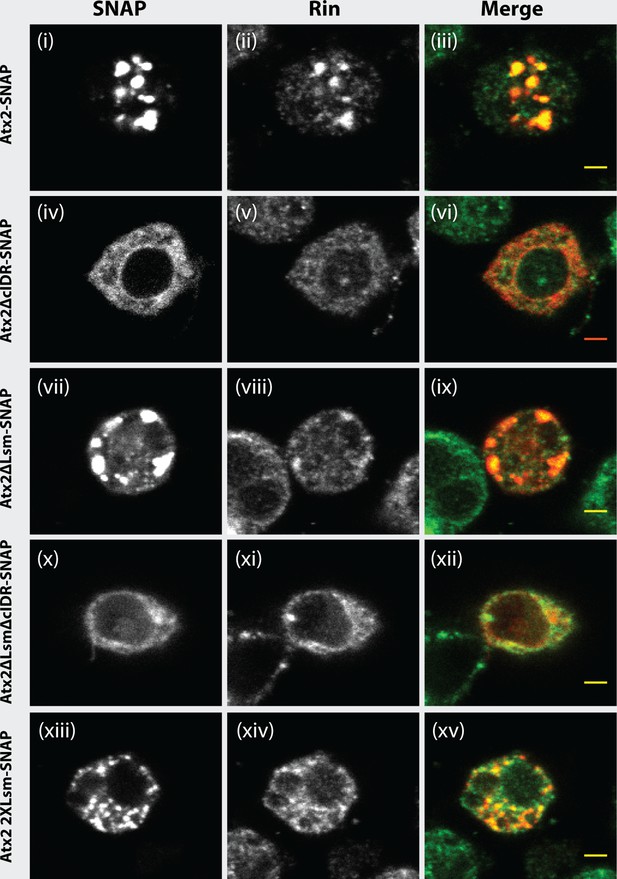
Granules formed by overexpression of Atx2 and ∆Lsm resemble stress granules (SGs) that also contain SG protein Rasputin (Rin).
Atx2 WT forms cytoplasmic granules (i) that contain Rin (ii and iii). The deletion of cIDR blocks granule formation (iv, v, and vi). Atx2 granules formed upon Like-Sm (Lsm) domain deletion also resemble Atx2 WT granules and contain SG protein Rin (vii, viii, and ix). The deletion of both Lsm and cIDR domains blocks the ability of Atx2 to form granules (x, xi, and xii). Atx2-2XLsm, where mIDR was replaced with a second Lsm domain, reduces Atx2 granule size but resembles WT granules and contains Rin (xiii, xiv, and xv). The scale bars correspond to 2 µm.
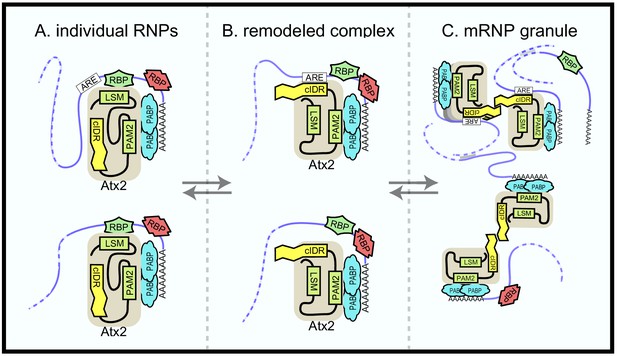
Ataxin-2 domains in mRNA target regulation.
A model to explain the current and previous observations on Ataxin-2 as well as its function in mRNA regulation. (A) Ataxin-2 does not make direct contact with mRNAs in soluble mRNPs. Instead, it is recruited to RNA by other RBPs that bind to structured Like-Sm (Lsm) (or Lsm-associated [LsmAD]) domains. In these soluble mRNPs, the cIDR is buried and inaccessible. One class of mRNP (above) contains AU-rich elements (AREs); the second class below (e.g., per in Drosophila) does not. (B) Under specific signaling conditions (e.g., stress), RBP–Atx2 interactions are prevented. In these ‘remodeled’ mRNPs, the Atx2-cIDR is exposed. We speculate that a segment of this intrinsically disordered domain (IDR) directly binds to nearby AREs (or to ARE-binding proteins), while other segments of the IDR mediate multivalent interactions that contribute to mRNP condensation. (C) Ataxin-2 cIDR interactions enable mRNP assembly into granules, facilitated by RNA–RNA crosslinks and interactions mediated by other RBPs (e.g., G3BP/Rin). These RNP granules may include both ARE-containing mRNAs (edited) and mRNAs that do not. Note that additional RBPs (red) could determine not only Atx2 proximity to specific mRNAs (leading to editing) but also the effect of Atx2 on mRNA translation and/or stability. These and alternative models remain to be tested.
Tables
Gene Ontology (GO) analysis of Atx2 brain targets.
GO analysis using PANTHER shows a large enrichment of neuronal mRNAs coding for neuropeptides and proteins involved in neuronal signaling pathways. FDR: false discovery rate.
Molecular function | Fold enrichment | Probability (FDR) | Targets (%) | Genome (%) | GO term |
---|---|---|---|---|---|
Signaling receptor binding | 7.75 | 4.47E-11 | 14.46 | 1.87 | GO:0005102 |
Neuropeptide hormone activity | 27.67 | 2.52E-09 | 6.63 | 0.24 | GO:0005184 |
Hormone activity | 18.64 | 5.74E-08 | 6.63 | 0.36 | GO:0005179 |
Neuropeptide receptor binding | 38.74 | 2.08E-06 | 4.22 | 0.11 | GO:0071855 |
G protein-coupled receptor binding | 13.84 | 3.79E-06 | 6.03 | 0.44 | GO:0001664 |
Receptor regulator activity | 9.58 | 4.55E-06 | 7.23 | 0.76 | GO:0030545 |
Receptor ligand activity | 9.41 | 1.96E-05 | 6.63 | 0.71 | GO:0048018 |
Signaling receptor activator activity | 9.04 | 2.58E-05 | 6.63 | 0.74 | GO:0030546 |
Organic hydroxy compound transmembrane transporter activity | 83.02 | 3.38E-04 | 2.41 | 0.03 | GO:1901618 |
Monoamine transmembrane transporter activity | 66.41 | 5.52E-04 | 2.41 | 0.04 | GO:0008504 |
Phosphoric ester hydrolase activity | 4.93 | 6.43E-04 | 7.84 | 1.59 | GO:0042578 |
Anion:sodium symporter activity | 55.35 | 6.44E-04 | 2.41 | 0.05 | GO:0015373 |
Sodium:chloride symporter activity | 55.35 | 6.84E-04 | 2.41 | 0.05 | GO:0015378 |
Phosphoprotein phosphatase activity | 7.95 | 7.06E-04 | 5.43 | 0.69 | GO:0004721 |
Anion:cation symporter activity | 36.9 | 1.81E-03 | 2.41 | 0.07 | GO:0015296 |
Cation:chloride symporter activity | 36.9 | 1.91E-03 | 2.41 | 0.07 | GO:0015377 |
Phosphatase activity | 5.05 | 2.53E-03 | 6.63 | 1.32 | GO:0016791 |
Serotonin:sodium symporter activity | 83.02 | 4.17E-03 | 1.81 | 0.03 | GO:0005335 |
Neurotransmitter:sodium symporter activity | 14.82 | 5.04E-03 | 3.02 | 0.21 | GO:0005328 |
Protein tyrosine/serine/threonine phosphatase activity | 23.72 | 6.17E-03 | 2.41 | 0.11 | GO:0008138 |
Solute:sodium symporter activity | 11.53 | 1.26E-02 | 3.02 | 0.27 | GO:0015370 |
mRNA 3'UTR binding | 11.22 | 1.36E-02 | 3.02 | 0.27 | GO:0003730 |
Protein tyrosine phosphatase activity | 10.64 | 1.59E-02 | 3.02 | 0.29 | GO:0004725 |
Neurotransmitter transmembrane transporter activity | 10.64 | 1.65E-02 | 3.02 | 0.29 | GO:0005326 |
Adrenergic receptor activity | 24.91 | 3.98E-02 | 1.81 | 0.08 | GO:0004935 |
-
Table 1—source data 1
Gene Ontology (GO) terms for Atx2 brain targets.
- https://cdn.elifesciences.org/articles/60326/elife-60326-table1-data1-v1.xlsx
Reagent type (species) or resource | Designation | Source or reference | Identifiers | Additional information |
---|---|---|---|---|
Genetic reagent (Drosophila melanogaster) | UAS-Atx2-WT-ADARcd | This paper | N/A | Related to Figures 1, 2 and 4 |
Genetic reagent (Drosophila melanogaster) | UAS-Atx2-ΔLsm-ADARcd | This paper | N/A | Related to Figure 4 |
Genetic reagent (Drosophila melanogaster) | UAS-Atx2- ΔLsm-AD-ADARcd | This paper | N/A | Related to Figure 4 |
Genetic reagent (Drosophila melanogaster) | (UAS-Atx2- ΔmIDR-ADARcd) | This paper | N/A | Related to Figure 4 |
Genetic reagent (Drosophila melanogaster) | UAS-Atx2- ΔcIDR-ADARcd | This paper | N/A | Related to Figure 4 |
Genetic reagent (Drosophila melanogaster) | UAS-Atx2-only Lsm/Lsm-AD -ADARcd | This paper | N/A | Related to Figure 4 |
Genetic reagent (Drosophila melanogaster) | Elav-Gal4; Tub-Gal80ts | Bloomington Drosophila Stock center | Related to Figure 3 | |
Genetic reagent (Drosophila melanogaster) | UAS-Atx2 RNAi | Vienna Drosophila RNAi Center stock collection | 34955 | Related to Figure 3 |
Cell line (Drosophila melanogaster) | S2R+ cells | DGRC | RRID:CVCL_Z831 | |
Recombinant DNA reagent | pJFRC7-20XUAS-IVS-8_Atx2-ADARcd (plasmid) | This paper | N/A | Construct to express WT Atx2-ADAR fusion protein |
Recombinant DNA reagent | pJFRC7-20XUAS- IVS-8_Atx2ΔmIDR -ADARcd (plasmid) | This paper | N/A | Construct to express Atx2∆mIDR-ADAR fusion protein |
Recombinant DNA reagent | pJFRC7-20XUAS-IVS-8_Atx2ΔcIDR -ADARcd (plasmid) | This paper | N/A | Construct to express Atx2∆cIDR-ADAR fusion protein |
Recombinant DNA reagent | pJFRC7-20XUAS-IVS-8_Atx2ΔLsm -ADARcd (plasmid) | This paper | N/A | Construct to express Atx2∆Lsm-ADAR fusion protein |
Recombinant DNA reagent | pJFRC7-20XUAS-IVS-8_Atx2ΔLsmAD-ADARcd (plasmid) | This paper | N/A | Construct to express Atx2∆LsmAD-ADAR fusion protein |
Recombinant DNA reagent | pJFRC7-20XUAS-IVS-8_Atx2only-Lsm-LsmAD-ADARcd (plasmid) | This paper | N/A | Construct to express only Lsm-LsmAD-ADAR fusion protein |
Antibody | Anti-Atx2 (chicken polyclonal) | Bakthavachalu et al., 2018 | IF (1:500) WB (1:1000) | |
Antibody | Anti-Rasputin (rabbit polyclonal) | Aguilera-Gomez et al., 2017 | IF (1:500) | |
Antibody | Anti-GFP (rabbit polyclonal) | Molecular probes | Cat# A11122 | IF (1:500) |
Antibody | Anti-GFP (chicken polyclonal) | Abcam | Cat# mAb 13970 | IF (1:500) |
Antibody | Anti-V5 (rabbit polyclonal) | Santa Cruz Biotechnology | Cat# sc83849-R | IF (1:500) WB (1:1000) |
Antibody | Anti-nc82 (mouse monoclonal) | Kittel, 2006 | IF (1:500) | |
Antibody | Anti-tubulin (mouse monoclonal) | DSHB | Cat# E7C | WB (1:2000) |
Antibody | Alexa Fluor 555 (polyclonal goat anti-chicken IgG) | Invitrogen | Cat# A21437 | IF (1:1000) |
Antibody | Alexa Fluor 488 (polyclonal goat anti-chicken IgG) | Invitrogen | Cat# A11039 | IF (1:1000) |
Antibody | Alexa Fluor 647 (polyclonal goat anti-chicken IgG) | Invitrogen | Cat# A21449 | IF (1:1000) |
Antibody | Alexa Fluor 555 (polyclonal goat anti-rabbit IgG) | Invitrogen | Cat# A21428 | IF (1:1000) |
Antibody | Alexa Fluor 488 (polyclonal goat anti-rabbit IgG) | Invitrogen | Cat# A11078 | IF (1:1000) |
Antibody | Alexa Fluor 647 (polyclonal goat anti-rabbit IgG) | Invitrogen | Cat# A21244 | IF (1:1000) |
Antibody | Alexa Fluor 555 (polyclonal goat anti-mouse IgG) | Invitrogen | Cat# A21422 | IF (1:1000) |
Antibody | Alexa Fluor 488 (polyclonal goat anti-mouse IgG) | Invitrogen | Cat# A21121 | IF (1:1000) |
Antibody | Alexa Fluor 647 (polyclonal goat anti-mouse IgG) | Invitrogen | Cat# A21235 | IF (1:1000) |
Chemical compound | Vectashield Mounting Medium | Vector Laboratories | Cat# H-1000 | |
Chemical compound | SNAP-Surface 549 | New England Biolabs | Cat# S9112S | IF (1:500) |
Software, algorithm | TRIBE | McMahon et al., 2016 | https://github.com/rosbashlab/TRIBE | |
Software, algorithm | STAR v2.5.3 | Dobin et al., 2013 | https://github.com/alexdobin/STAR | |
Software, algorithm | HTSeq v0.11.2 | Anders et al., 2015 | https://github.com/htseq/htseq | |
Software, algorithm | DESeq2 | Love et al., 2014 | https://bioconductor.org/packages/release/bioc/html/DESeq2.html | |
Software, algorithm | AREScore | Spasic et al., 2012 | http://arescore.dkfz.de/arescore.pl | |
Software, algorithm | Guitar | Cui et al., 2016 | https://bioconductor.org/packages/release/bioc/html/Guitar.html | |
Software, algorithm | Bedtools | Quinlan and Hall, 2010 | https://github.com/arq5x/bedtools2 | |
Software, algorithm | twoBitToFa | - | https://genome.ucsc.edu/goldenPath/help/twoBit.html | |
Software, algorithm | MEME suite | Bailey et al., 2009 | http://meme-suite.org/tools/meme | |
Software, algorithm | Cellprofiler | McQuin et al., 2018 | https://cellprofiler.org | |
Software, algorithm | ImageJ | Schneider et al., 2012 | https://imagej.nih.gov/ij/ | |
Software, algorithm | Ggplot2 | Wilkinson, 2011 | https://github.com/tidyverse/ggplot2 | |
Software, algorithm | Pheatmap | https://cran.r-project.org/web/packages/pheatmap/index.html | ||
Software, algorithm | SnapDragon | https://www.flyrnai.org/snapdragon |
Additional files
-
Supplementary file 1
Details of all the primers used in the study.
- https://cdn.elifesciences.org/articles/60326/elife-60326-supp1-v1.docx
-
Supplementary file 2
All the edits identified (without thresholding) in Targets of RNA-Binding Proteins Identified by Editing experiments are consolidated as a single spreadsheet file.
Each genotype is presented as a sheet within this file, and the raw and processed sequencing data is available at GEO accession number GSE153985.
- https://cdn.elifesciences.org/articles/60326/elife-60326-supp2-v1.xlsx
-
Transparent reporting form
- https://cdn.elifesciences.org/articles/60326/elife-60326-transrepform-v1.docx