Inhibition of SARS-CoV-2 viral entry upon blocking N- and O-glycan elaboration
Figures
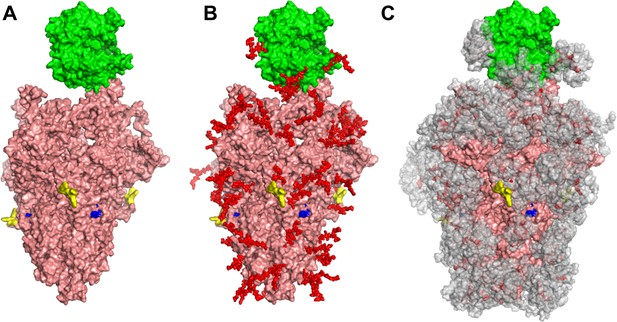
Glycan coverage of Spike-ACE2 co-complex.
SARS-CoV-2 Spike protein trimer (pink) bound to ACE2 (green). (A) Without glycans. (B) With N-glycans (red) identified using LC-MS on Spike and ACE2. (C) Molecular dynamics simulation analyzed the range of movement of each glycan. The space sampled by glycans is represented by a gray cloud. Glycans cover the Spike-ACE2 interface. They also surround the putative proteolysis site of furin (‘S1-S2’, yellow) and S2’ (blue).
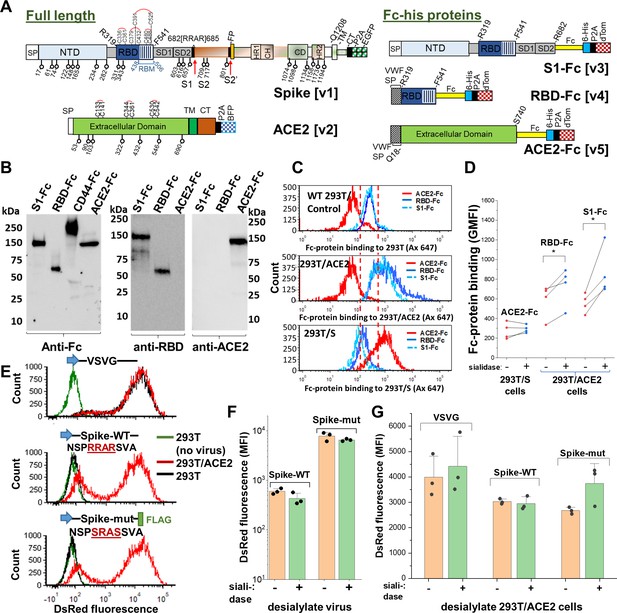
Sialic acid has modest effect on Spike binding and viral entry.
(A) Full-length proteins expressed on cells include wild-type Spike-protein [v1] and human ACE2 [v2]. N-glycosylation sites are indicated by lollipop. Fc-his soluble proteins encode for S1-subunit [v3], RBD [v4] and soluble ACE2 [v5]. All constructs were co-expressed with fluorescent reporters separated by P2A. Note that the Fc-section also contains one N-glycosylation site. (B) Western blot for purified Fc-proteins from HEK293T probed with anti-Fc, anti-RBD or anti-ACE2 Ab. CD44-Fc is positive control. (C) Flow cytometry data showing S1-Fc (1.7 µg/mL) and RBD-Fc (0.35 µg/mL) binding to ACE2 expressed on HEK293T (middle panel). Spike expression enhances ACE2-Fc (1.4 µg/mL) binding (bottom). (D) Desialylation of Spike-protein expressed on 293 T/S had minimal effect on ACE2-Fc (0.7 µg/mL) binding. ACE2 desialylation on 293T/ACE2 increased binding of RBD-Fc (0.2 µg/mL) and S1-Fc (1.7 µg/mL) by 26–56% (paired experiments, *p<0.05). (E) Pseudovirus with DsRed-reporter were developed with three different envelope proteins. VSVG pseudotyped virus infected both HEK293T (black line) and stable 293T/ACE2 (red line) cells. Virus with Spike-WT and Spike-mutant entered 293T/ACE2 only. (F) Same titer of virus (0.3 µg/mL p24-equivalent) were treated with or without sialidase, prior to addition to stable 293T/ACE2 cells. Infection using Spike-mutant was higher compared to Spike-WT. Sialidase treatment of virus had no effect. (G) 293T/ACE2 cells were sialidase treated prior to addition of VSVG (0.3 µg/mL p24-equiv.), Spike-WT (1.5 µg/mL p24-equiv.) or Spike-mutant (0.2 µg/mL p24-equiv.) pseudovirus. Sialidase treatment did not affect viral entry. Abbreviations: Spike signal peptide (SP), N-terminal domain (NTD), receptor-binding domain (RBD), receptor-binding motif (RBM), subdomain 1 (SD1), subdomain 2 (SD2), fusion peptide (FP), heptad repeat 1 (HR1), central helix (CH), connector domain (CD), heptad repeat 2 (HR2) transmembrane section (TM), cytoplasmic tail (CT), ACE2: Angiotensin-converting enzyme-2; VSVG: Vesicular stomatitis virus G-protein; WT: wild-type; mut: mutant.
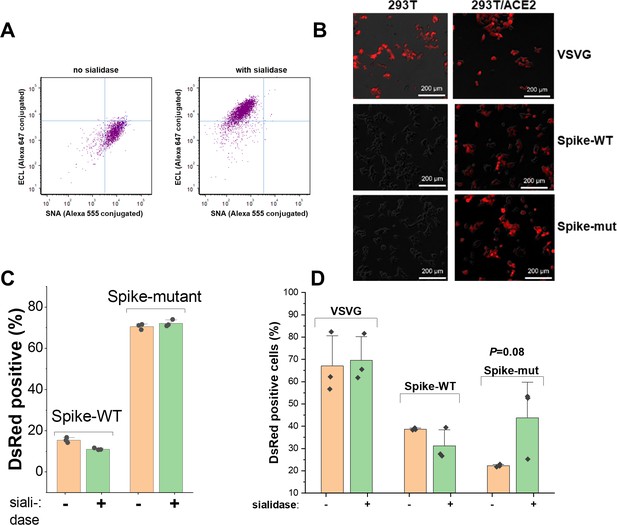
Sialidase treatment studies.
(A) Sialidase protocol validation. All lectins were directly conjugated with Alexa dyes. They were incubated with cells at 1–5 µg/mL for 15 min before a quick wash and cytometry measurement. Compared to untreated control (left), sialidase treatment (right) decreased SNA lectin binding to α2,6 sialylated structures by 15-fold and increased ECL binding to desialylated lactosamine chains (Galβ1,4GlcNAcβ) by an order of magnitude. (B) Pseudovirus assay. DsRed fluorescence in HEK293T and stable 293T/ACE2 cells upon addition of VSVG, Spike-WT and Spike-mutant pseudotyped virus. (C) Sialidase treatment of pseudovirus. % DsRed positive cell data are shown for study in Figure 2F (main manuscript). Viral entry was sialidase independent. (D) Sialidase treatment of HEK/ACE2 cells. Pseudovirus expressing VSVG, Spike-WT and Spike-mutant were added to cells under conditions described in Figure 2G (main manuscript). All error bars are standard deviations. Data are representative of 3 independent runs.
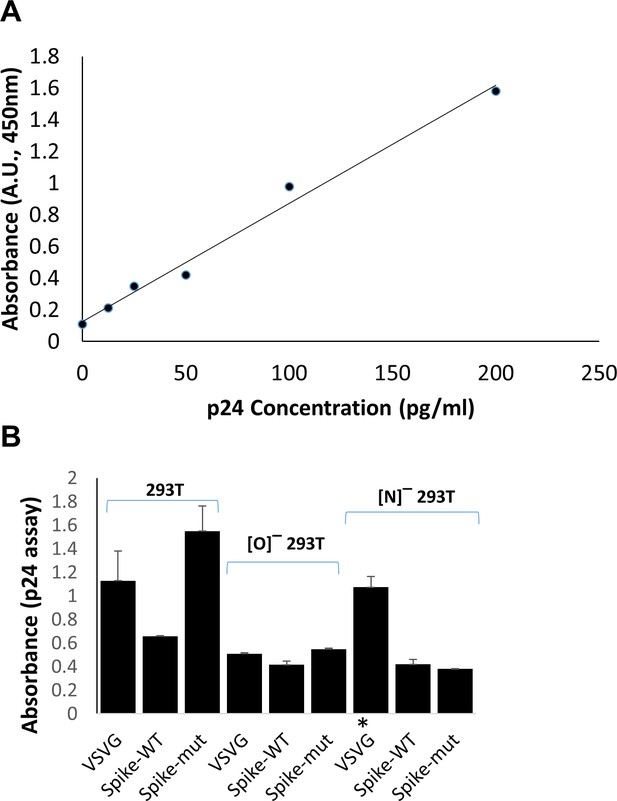
p24 assay to determine viral titer.
(A) Representative calibration curve for p24 standard. (B) Absorbance value for viral stock in representative ELISA run. Nine different viruses were prepared and used in Figure 4 (main manuscript). All viruses were diluted 1:500,000 fold before ELISA measurement, except for VSVG virus made in [N]¯ 293 T cells (indicated by asterisk) which were diluted 1:50,000 fold, in this instance, due to lower titer.
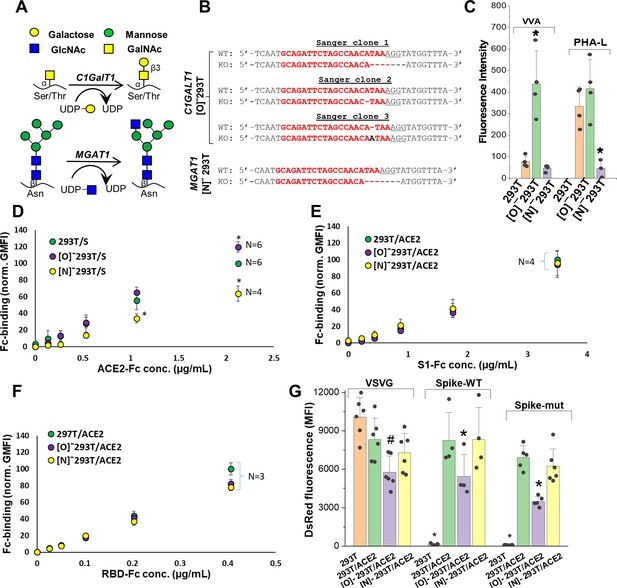
ACE2 glycosylation does not affect viral entry.
(A) Knocking out C1GALT1 and MGAT1 using CRISPR-Cas9 inhibits O- and N-glycan biosynthesis in HEK293Ts. (B) Sanger sequencing results of isogenic 293T clones shows indels on all 3 alleles of C1GALT1 (‘[O]-293T’) and single allele of MGAT1 (‘[N]-293T’) knockout cells. Wild-type (WT) sequence is on the first line. Lower line shows base deletions (hyphen) and insertions (black fonts) for individual KOs. sgRNA target sequence is in red and protospacer adjacent motif is underlined. (C) Increased VVA and reduced PHA-L binding confirm loss of O-linked glycans in [O]-293Ts and N-glycans in [N]-293Ts, respectively. (D) Knocking out N-glycans on Spike protein reduced ACE2-Fc binding in cytometry based binding studies. Knocking out Spike O-glycans increased ACE-2 binding. (E–F) Truncation of ACE2 N- and O-glycans did not affect either S1-Fc (panel E) or RBD-Fc (panel F) binding. (G) ACE2 was transiently expressed on 293T, [O]-293T and [N]-293 T cells. All pseudotyped virus efficiently entered ACE2 expressing cells. Virus was not titered for these runs, and thus comparison between viruses is not possible. *p<0.05 with respect to all other treatments. #p<0.05 with respect to 293T and [N]-293T/ACE2 in panel G.
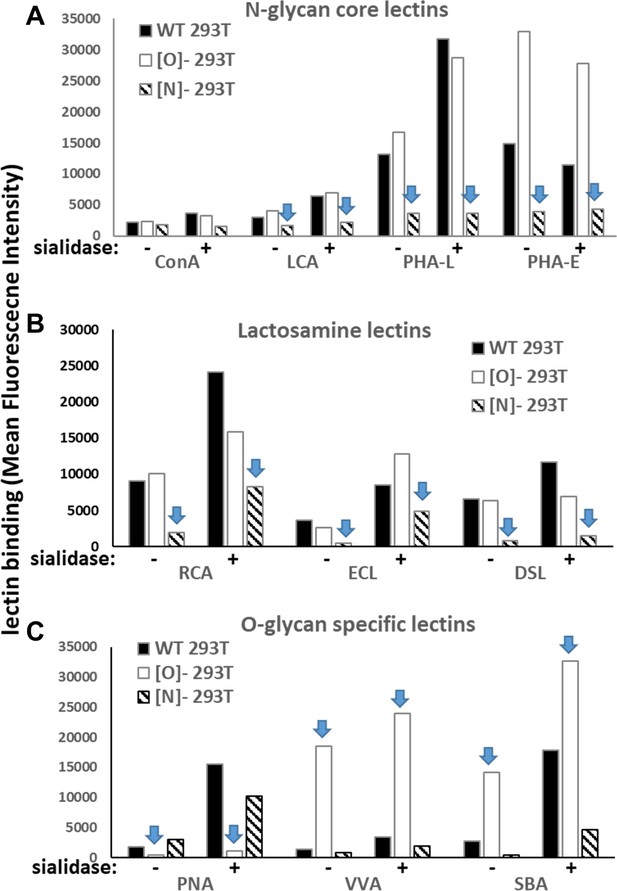
Lectin binding to wild-type and glycogene-KO 293 T cells.
A panel of lectins (from Vector Labs) was conjugated with Alexa dyes, either Alexa 405, 488 or 647. The binding of these fluorescent reagents to wild-type 293T, [N]-293T and [O]-293 T cells was measured using flow cytometry. The lectins bound: (A) N-glycan high-mannose and complex structures [ConA and LCA bind αMan in high-mannose glycans; PHA-L and PHA-E bind complex glycans], (B) lactosamine chains primarily on N-linked glycans [RCA, ECL bind terminal Gal or lactose; DSL bind β1,4GlcNAc], and (C) O-glycan related structures [PNA binds Galβ1,GalNAc; VVA and SBA bind GalNAcα]. Measurements were made with either untreated or sialidase treated 293 T cells. As seen: i. Knocking out MGAT1 in [N]-293T reduces lectin binding in panels A and B (see arrow). ii. Knocking out C1GalT1 results in a dramatic decrease in PNA binding and increase in VVA and SBA binding, These data are consistent with the expected changes in lectin profile upon knocking out these N- and O-glycan-specific enzymes.
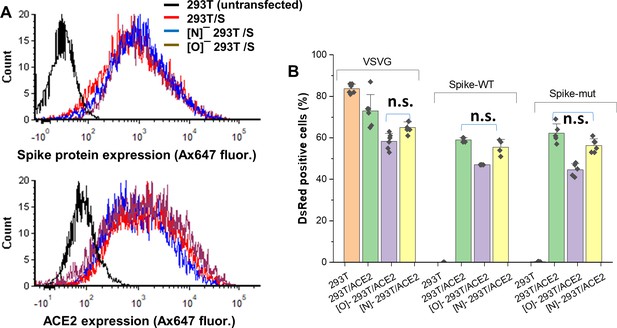
Effect of ACE2 glycosylation on viral entry.
(A) Surface expression of Spike-protein and ACE2. Full-length Spike (top) and human ACE2 (bottom) were expressed in HEK 293T, [N]-293T and [O]-293T. Protein expression was measured in EGFP+ cells in the case of Spike (using anti-RBD), and on BFP+ cells in the case of ACE2 (using anti-ACE2), as these fluorescent reporters are co-expressed with surface proteins. Protein expression was comparable in all cells. Untransfected 293Ts serve as negative control. (B) Viral entry assay. Pseudovirus expressing VSVG envelope protein, Spike-WT or Spike-mutant were added to HEK 293 T cells transiently transfected to overexpress ACE2 (both wild-type 293T and glycosylation mutants). An additional control included 293 T cells not expressing ACE2, which only allowed entry of VSVG pseudotyped viral particles, but not Spike bearing virus. % cells that were DsRed (reporter) positive is shown at 72 hr. All treatments were statistically different except as indicated by n.s. (‘not significant’). All data are from of N > 3 repeats.
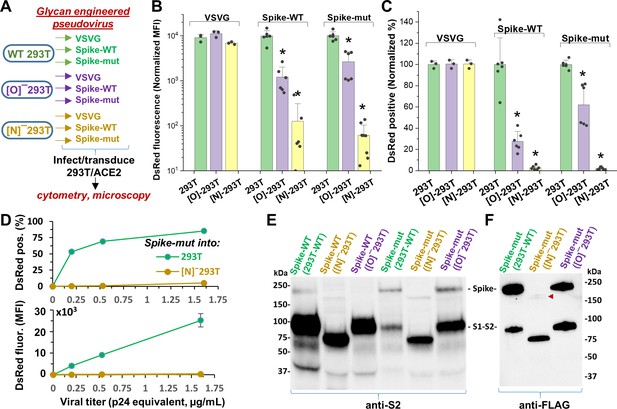
N-glycan modification of SARS-CoV-2 pseudovirus abolishes entry into 293T/ACE2 cells.
(A) Pseudovirus expressing VSVG envelope protein, Spike-WT and Spike-mutant were produced in wild-type, [O]- and [N]-293 T cells. All nine viruses were applied at equal titer to stable 293T/ACE2. (B–C) O-glycan truncation of Spike partially reduced viral entry. N-glycan truncation abolished viral entry. In order to combine data from multiple viral preparations and independent runs in a single plot, all data were normalized by setting DsRed signal produced by virus generated in wild-type 293T to 10,000 normalized MFI or 100% normalized DsRed positive value. (D) Viral titration study performed with Spike-mutant virus shows complete loss of viral infection over a wide range. (E) Western blot of Spike protein using anti-S2 Ab shows reduced proteolysis of Spike-mut compared to Spike-WT. The full Spike protein and free S2-subunit resulting from S1-S2 cleavage is indicated. Molecular mass is reduced in [N]-293T products due to truncation of glycan biosynthesis. (F) Anti-FLAG Ab binds the C-terminus of Spike-mutant. Spike produced in [N]-293Ts is almost fully proteolyzed during viral production (red arrowhead). *p<0.05 with respect to all other treatments.
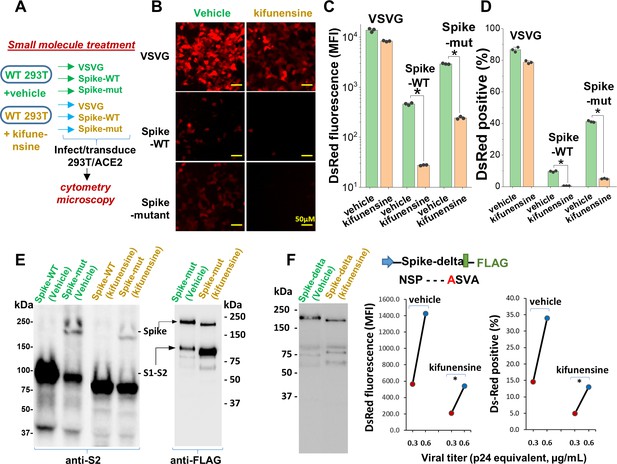
Mannosidase-I blockade using kifunensine inhibits SARS-CoV-2 pseudovirus entry into 293T/ACE2 cells.
(A) VSVG, Spike-WT and Spike-mutant pseudovirus were produced in the presence of 15 µM kifunensine or vehicle control. The six viruses were added to 293T/ACE2 at equal titer. (B–D) Microscopy (panel B) and cytometry (panel C, D) show ~90% loss of viral infection in the case of Spike-WT and Spike-mutant virus upon kifunensine treatment (*p<0.05). (E) Spike molecular mass is reduced in the western blots due to high-mannose glycan synthesis in runs with kifunensine. Intact Spike is reduced in the presence of kifunensine, in anti-FLAG blot. (F) The polybasic furin ‘RRAR’ site was substituted by a single ‘A’ amino acid in Spike-delta. Virus with Spike-delta were expressed both in the presence of vehicle and kifunensine. Western blot shows lack of S1-S2 cleavage in this construct. In viral entry assay, kifunensine reduced DsRed expression in 293T/ACE2 cells, even in the case of Spike-delta pseudovirus (*p<0.05). Similar observation was made at two different viral titers (0.3 and 0.6 μg/mL p24 equivalent).
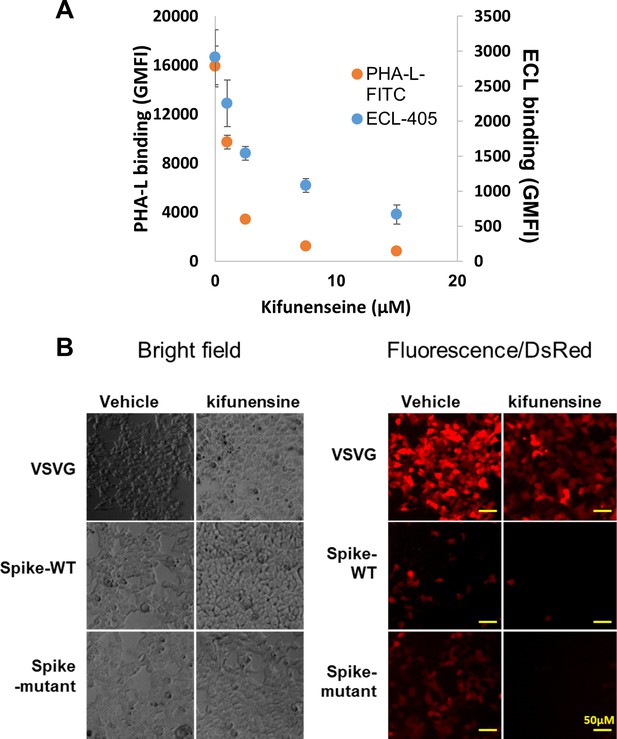
Effect of kifunensine.
(A) Lectin binding upon kifunensine treatment. HEK293T cells were cultured with varying concentrations of kifunensine or vehicle for 2 days. PHA-L binding measurement shows marked reduction in the cell-surface expression of complex glycans and also a decrease in ECL engagement (recognizes lactosamine chains). Kifunensine did not affect cell growth rate or viability. It also did not reduce pseudovirus production titers. Data are Mean ± STD (N = 3). (B) Kifunensine reduced Spike viral infection. Bright field images for cell monolayer (left) for microscopy data shown in main manuscript (reproduced in right panels). Kifunensine reduces Spike-WT and Spike-mutant pseudoviral entry by ~85–90%.
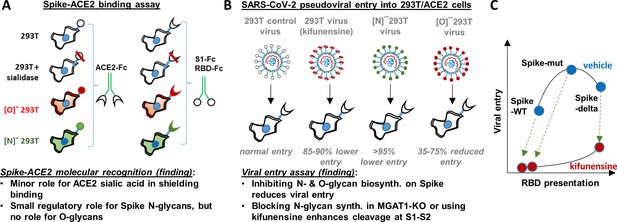
Principal findings and conceptual model.
(A) ACE2-Fc binding was measured to wild-type or glycoEnzyme-KO 293 T cells expressing Spike. Sialidase treatment of cells was performed in some cases. Similar studies also measured S1-Fc and RBD-Fc binding to cell-surface expressed ACE2. (B) SARS-CoV-2 pseudovirus (bearing Spike-WT, Spike-mut, Spike-delta variants) were generated in wild-type or glycoEnzyme-KO 293Ts, in the presence and absence of kifunensine. Main results of binding (A) and viral entry (B) assay are listed. (C) Conceptual model shows that kifunensine can induce S1-S2 site proteolysis on Spike-WT and Spike-mut virus, but not Spike-delta virus. This proteolysis reduces RBD presentation and attenuates viral entry into 293T/ACE2. Without affecting S1-S2 cleavage, kifunensine also partially reduced Spike-delta pseudovirus entry function. The data suggest additional roles for Spike N-glycans during viral entry.

Distribution of N-glycans in the Fc-section of ACE2-Fc (top, Asn815) and S1-Fc (bottom, Asn 757).
Label-free quantitative analysis helped classify glycans located at individual peptide sites into different sub-groups. This classification was based on: Group 1: relative abundance of high-mannose, hybrid, complex and unoccupied sites on the peptide backbone.; Group 2: relative abundance of fucosylated glycans containing either only core-fucose (i.e. α1,6 linked), terminal-fucose, or both core and terminal fucose. The remaining (glycol)peptide spectra did not contain fucose. Group 3: relative abundance of complex glycans in mono-, bi-, tri and tetra-antennary branched structures; Group 4: Relative abundance of terminal chains on complex glycans, that were capped by either Gal, GlcNAc or Neu5Ac (sialic acid). Each dot is from a single MS run that was either trypsin or Glu-C digested. For each site, we present: i. site of glycosylation, ii. # of PSM analyzed corresponding to this site, iii. Number of unique glycans detected at that location and iv. total number of unique glycopeptides that were analyzed at that site. All identifications are manually verified, and detailed MS/MS annotations are available upon request. Number of PSMs analyzed at each site is provided along with the number of unique glycans at that site. Data are Mean +/- STD for >3 independent LC-MS/MS runs.
Tables
Reagent type (species) or resource | Designation | Source or reference | Identifiers | Additional information |
---|---|---|---|---|
Antibody | Anti-RBD Spike (rabbit polyclonal) | Sino Biologicals (Beijing, China) | Cat#: 40592-T62 | WB (1:5000) |
Antibody | Anti-S2 Spike (rabbit polyclonal) | Sino Biologicals (Beijing, China) | Cat#: 40590-T62 | WB (1:5000) |
Antibody | Anti-human ACE2 AF933 (goat polyclonal) | R and D Systems | Cat#: AF933 RRID:AB_355722 | Binding assay (1 ul) |
Antibody | Anti-human ACE2 (mouse monoclonal) | R and D Systems | Cat#: MAB9332 | Binding assay (1 ul) |
Antibody | Anti-FLAG clone L5, (rat monoclonal) | Biolegend (San Diego, CA) | Cat# 637303 RRID:AB_1134265 | WB (1:5000) |
Antibody | Anti-human F(ab’)two conjugated with Alexa Fluor FITC (goat polyclonal) | Jackson ImmunoResearch | Cat# 109-096-098 RRID:AB_2337668 | Binding assay (1:150) |
Antibody | Anti-human F(ab’)two conjugated with Alexa Fluor 647 (goat polyclonal) | Jackson ImmunoResearch | Cat# 109-606-098 RRID:AB_2337899 | Binding assay (1:150) |
Other (plant lectin) | PHA-L (Phaseolus vulgaris Leucoagglutinin, binds Galβ1,4GlcNAcβ1,6(GlcNAcβ1,2Manα1,3)Manα1,3 on complex N-glycans) | Vector Laboratories | Cat# FL-1111 RRID:AB_2336655 | Lectin binding study (1:200) |
Other (plant lectin) | VVA-lectin (binds GalNAcα) | Vector Laboratories | Cat# AL-1233 RRID:AB_2336854 | Lectin binding study (1:200) |
Other (plant lectin) | SNA (Sambucus nigra lectin, binds primarily α2,6 sialic acid) | Vector Laboratories | Cat# FL-1301 RRID:AB_2336719 | Lectin binding study (1:200) |
Other (plant lectin) | ECL (Erythrina cristagalli lectin, binds desialylated lactosamine Galβ1,4GlcNAc chains) | Vector Laboratories | Cat# L-1140 RRID:AB_2336438 | Lectin binding study (1:500) |
Strain, strain background (HIV-1 lentivirus) | Lentivirus with DsRed reporter and various envelope proteins | This paper | Various pseudovirus types are made in this paper by authors | |
Peptide, recombinant protein | Sialidase (Arthrobacter ureafaciens α2–3,6,8,9-Neuraminidase) | New England BioLabs | Cat#: P0722S | |
Chemical compound, drug | Kifunensine | Toronto Research Chemicals | Cat#: K450000 | |
Cell line (Homo-Sapiens) | HEK 293T | ATCC | Cat# KCB 200744YJ RRID:CVCL_0063 | |
Cell line (Homo-sapiens) | HEK 293T Lenti-X | Clontech/Takara Bio | Cat#: 632180 | |
Cell line (Homo-sapiens) | HEK/ACE2 | Michael Farzan (Scripps Research, Jupiter, FL) | ||
Cell line (Homo-sapiens) | [O]-293T | This paper | C1GalT1 Knock out HEK 293 T cell | |
Cell line (Homo-sapiens) | [N]-293T | This paper | MGAT1 Knock out HEK 293 T cell | |
Recombinant DNA reagent | Spike-WT [v1] | Dr. Haisheng Yu (Institute of Laboratory Animal Science, Peking Union Medical College) | Plasmid to express full length Spike | |
Recombinant DNA reagent | ACE2 [v2] | This paper | Derived from RRID:Addgene_1786 | Plasmid to express full length human ACE2 |
Recombinant DNA reagent | S1-Fc [v3] | This paper | Plasmid to express S1 as an human Fc IgG1 fusion protein | |
Recombinant DNA reagent | RBD-Fc [v4] | This paper | Plasmid to express RBD as an human Fc IgG1 fusion protein | |
Recombinant DNA reagent | ACE2-Fc [v5] | This paper | Plasmid to express ACE2 as an human Fc IgG1 fusion protein | |
Recombinant DNA reagent | Spike-mutant | Michael Farzan (Scripps Research, Jupiter, FL) | Plasmid to express Spike-mutant | |
Recombinant DNA reagent | Spike-delta | This paper | Plasmid to express Spike-delta on viral coat | |
Recombinant DNA reagent | psPAX2 | Addgene | Cat#: 12260 RRID:Addgene_12260 | |
Recombinant DNA reagent | pLKO.1 TRC-DsRed | Buffone et al., 2013 | ||
Recombinant DNA reagent | VSVG | Addgene | Cat#: 12259 RRID:Addgene_12259 |