A self-balancing circuit centered on MoOsm1 kinase governs adaptive responses to host-derived ROS in Magnaporthe oryzae
Figures
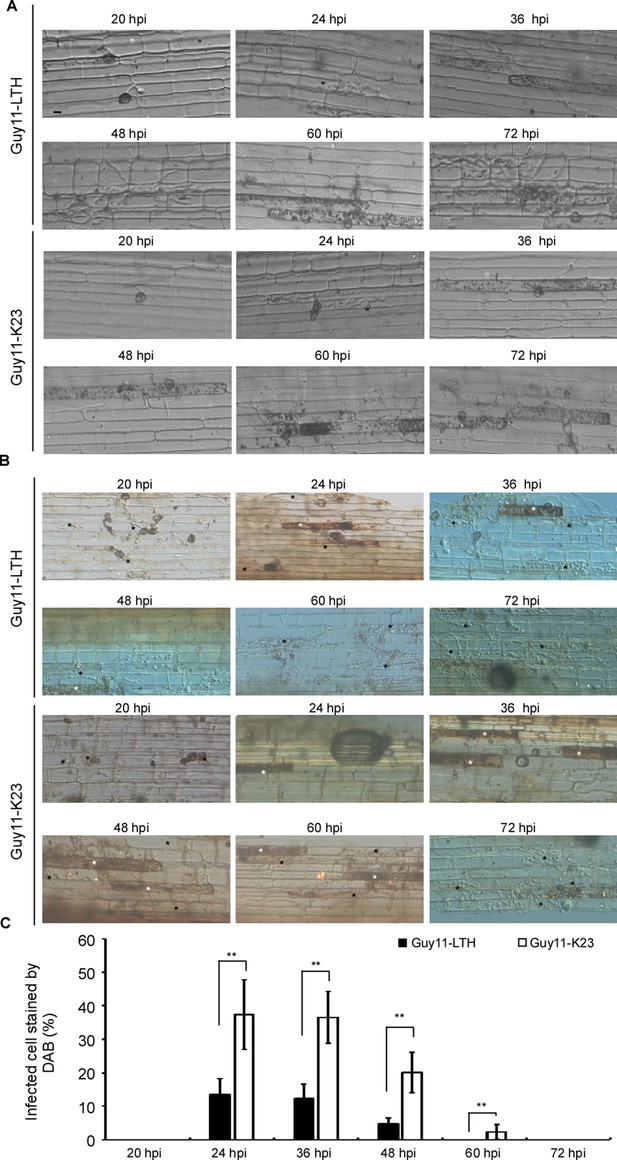
Time-course images of ROS accumulation during rice sheath infection by M. oryzae.
(A) The conidial suspension of Guy11 (1 × 105 spores/ml) was inoculated in the excised rice sheath of 4-weeks-old rice seedlings LTH and K23. The invasive hyphae growth was observed at 20, 24, 36, 48, 60, and 72 hpi. Black asterisks represent the bulbous infection hyphae (IH). (B) DAB staining shows ROS accumulation in rice LTH and K23 cells at various time points following infection. Black asterisks represent infected cells without ROS and white asterisks represent cells stained by DAB. (C) Infected cells stained by DAB. Over 50 infected rice cells were calculated with three replicates each time. ‘%” represents to the rate of infected cells which stained by DAB in all infected cells. Three independent biological experiments were performed and yielded similar results. Error bars represent standard deviation, and asterisks represent significant differences between the different strains (p<0.01).
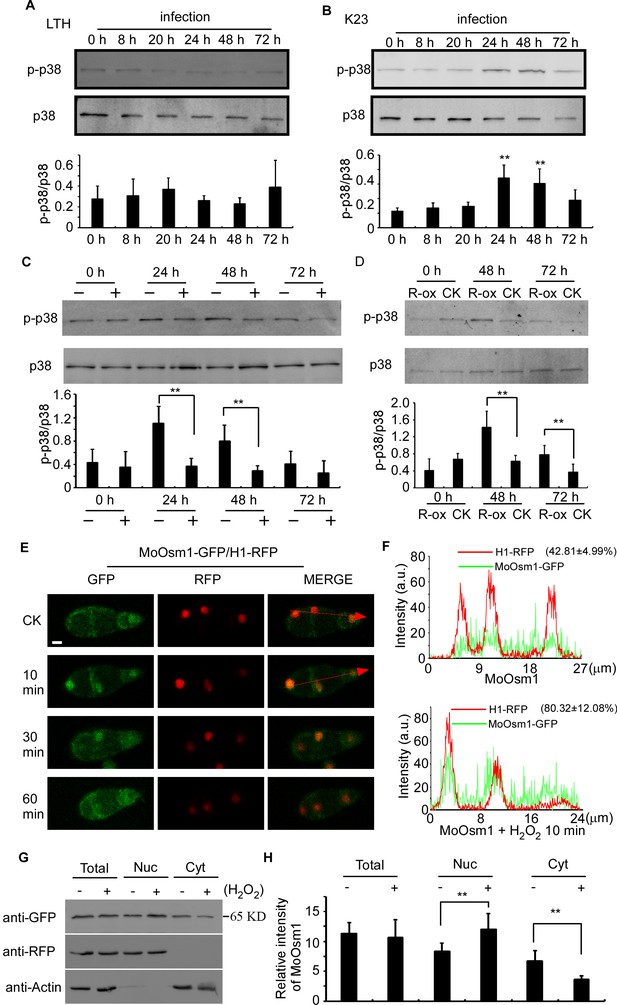
MoOsm1 phosphorylation and nuclear translocation in response to ROS stress.
(A) Phosphorylation of MoOsm1 in Guy11 in infection of LTH. Total proteins were extracted from LTH leaves 0, 8, 20, 24, 48, and 72 hr. Eluted proteins bound to the anti-GFP beads were analyzed by the antiphospho-p38 antibody, with the p38 antibody used as a control. The extent of phosphorylation was estimated by calculating the amount of antiphospho-p38 compared to the p38 (the histogram underneath the blot). Error bars represent standard deviation. (B) Phosphorylation of MoOsm1 in Guy11 in infection of K23. MoOsm1 phosphorylation was induced at 24 and 48 hpi. Error bars represent standard deviation, and asterisks represent significant differences between the different strains. Values are the means of 3 replications, and error bars represent the SD (n = 3). The asterisks indicate a significant difference (Duncan's new multiple range test, p<0.01). (C) DPI treatment in rice decreases the phosphorylation levels of MoOsm1 during infection. Total proteins were extracted from K23 leaves 0, 24, 48, and 72 hr with (+) or without (−) DPI treatment. MoOsm1 was purified and analyzed by the antiphospho-p38 antibody, with the p38 antibody used as a control. (D) Overexpression of OsRbohA induced the phosphorylation of MoOsm1. Total proteins were extracted from OsRbohA-ox leaves at 0, 48 and 72 hpi. MoOsm1 was purified and analyzed by the antiphospho-p38 antibody. NPB was used as the CK. R-ox represents OsRbohA-ox lines. (E) Localization of MoOsm1 under oxidative stress. Fluorescence observation of conidia treated with H2O2 for 10, 30, and 60 min. MoOsm1-GFP and H1-RFP were observed by confocal fluorescence microscopy. Bars = 5 μm. (F) Fluorescence intensity of MoOsm1-GFP/H1-RFP was observed with 10 min H2O2 treatment and CK. The green line represents MoOsm1-GFP while the red line represents H1-RFP. Insets highlight areas analyzed by line-scan. The number represents the quantification of GFP and RFP signals by ImageJ. (G) An equal amount (8 × 106 spore/ml x 60 ml) of conidia (with 10 min H2O2 treatment or not) were divided into three parts for extraction of the total, nuclear, and cytoplasm proteins. Equal amounts of the total, nuclear and cytoplasm proteins were separated by SDS-PAGE, and MoOsm1 was detected by western blotting using the anti-GFP antibody. Bands of MoOsm1-GFP were detected at 65kD. The intensity of western blotting bands was quantified with the ODYSSEY infrared imaging system (application software Version 2.1). The intensity of MoOsm1 was compared between the conidia without treatment (-) and conidia with 10 min of H2O2 treatment (+) among total proteins, nuclear proteins, and cytoplasmic proteins. H1 (a nucleus marker) and actin (a cytoplasm marker) were detected by western blotting analysis. Bars denote standard errors from three independent experiments. Asterisks indicate significant differences (Duncan's new multiple range test p<0.01).
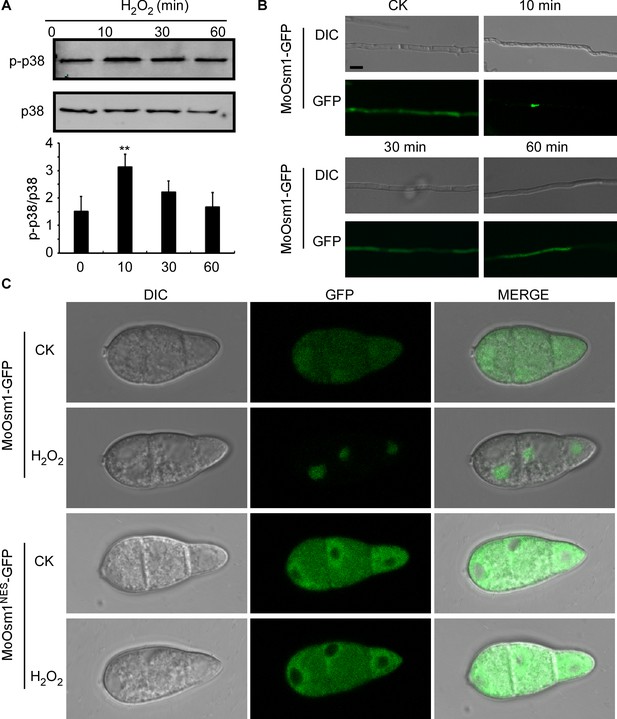
Localization of MoOsm1 under H2O2 stress in the conidium and mycelium.
(A) Phosphorylation of MoOsm1 in Guy11 under oxidative stress. Immunoblots were performed with total proteins from hyphae of Guy11 treated with H2O2 for 0, 10, 30, and 60 min. MoOsm1 phosphorylation was induced at 10 min with H2O2 treatment. Values are the means of 3 replications, and error bars represent the SD (n = 3). The asterisks indicate a significant difference (Duncan's new multiple range test, p<0.01). (B) Fluorescence observation of mycelium treated with H2O2 for 10, 30, and 60 min. (C) Fluorescence observation of conidia treated with H2O2 for 10 min. MoOsm1NES-GFP (NES: CTTCAACTTCCTCCTCTTGAAAGACTTACTCTT) was used as a negative control.
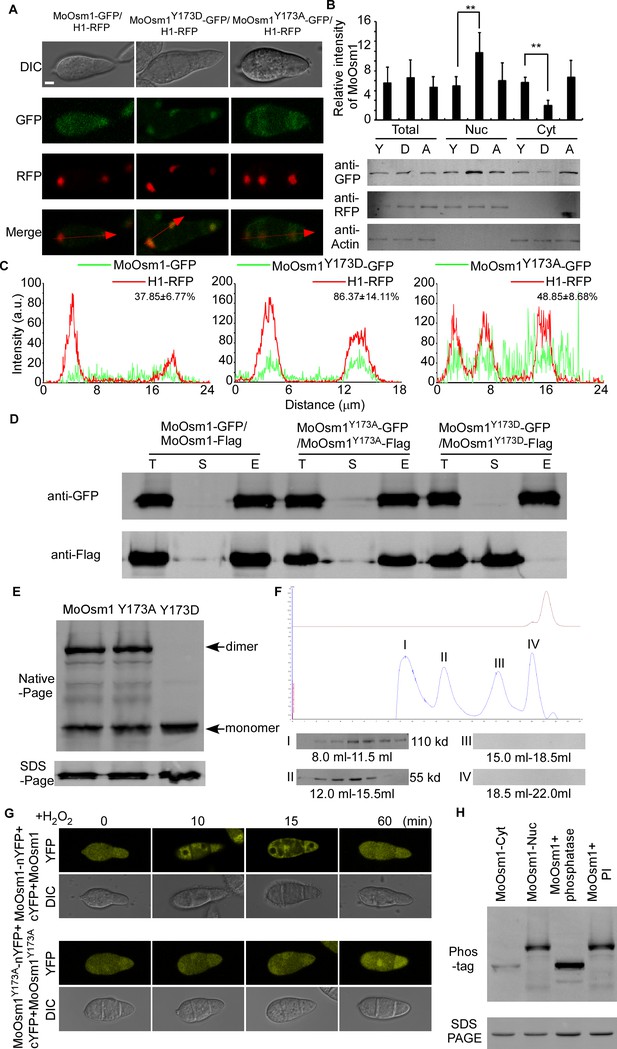
Phosphorylation-mediated monomerization of MoOsm1 results in its nuclear accumulation.
(A) Localization of MoOsm1, MoOsm1Y173D, and MoOsm1Y173A in conidia. MoOsm1-GFP, MoOsm1Y173D-GFP, MoOsm1Y173A-GFP, and H1-RFP were observed by confocal fluorescence microscopy. Bars = 5 μm. (B) Nuclear/cytoplasmic proteins harvested from conidia of ∆Moosm1/MoOsm1-GFP, ∆Moosm1/MoOsm1Y173D-GFP, and ∆Moosm1/MoOsm1Y173A-GFP strains were separated by SDS-PAGE. The intensity of MoOsm1 was compared among total proteins, nuclear proteins, and cytoplasmic proteins. ‘Y’ represents MoOsm1, ‘D’ represents MoOsm1Y173D, and ‘A’ represents MoOsm1Y173A. Bars denote standard errors from three independent experiments. Asterisks indicate significant differences (Duncan's new multiple range test p<0.01). (C) Fluorescence intensity of MoOsm1-GFP/H1-RFP in ∆Moosm1/MoOsm1-GFP, ∆Moosm1/MoOsm1Y173A-GFP, and ∆Moosm1/MoOsm1Y173D-GFP strains. The number represents the quantification of GFP and RFP signals by ImageJ. (D) Co-IP assay. Western blot analysis of total proteins (T) extracted from various transformants, suspension proteins (S), and elution proteins (E) eluted from anti-GFP beads. MoOsm1-GFP, MoOsm1Y173D-GFP, MoOsm1Y173A-GFP, and MoOsm1-FLAG were detected with respective antibodies. (E) Immunoblot analysis of proteins extracted from MoOsm1-GFP/∆Moosm1, MoOsm1Y173A-GFP/∆Moosm1, and MoOsm1Y173D-GFP/∆Moosm1 strains. (F) Gel-filtration chromatography assay of MoOsm1 dimerization. MoOsm1 was separated by the AKTA protein purification system (GE healthcare). Four protein peaks (I, II, III, and IV) were detected by western blot analysis. The brown line represents the ion peak. (G) BiFC assays for MoOsm1-nYFP and cYFP-MoOsm1 interactions. Conidia were treated with H2O2. MoOsm1Y173A-nYFP and cYFP-MoOsm1Y173A were used as control. (H) In vivo phosphorylation analysis of MoOsm1. Nuclear and cytoplasmic MoOsm1 phosphorylation was analyzed by Mn2+-Phos-tag SDS-PAGE and normal SDS-PAGE, respectively. Total proteins treated with alkaline phosphatase (phosphatase) and phosphatase inhibitor (inhibitor) were used as control.
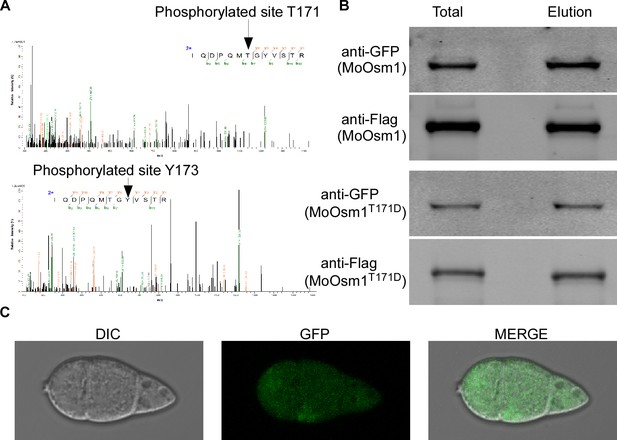
Thr171 and Tyr173 are important phosphorylation sites in MoOsm1.
(A) MoOsm1 phosphopeptide (QDPQMTGYVSTR) in the strain, which treated with H2O2 was identified by mass spectrometer analysis and the phosphorylated sites were Thr171 and Tyr-173. (B) Co-IP assay showed that phosphomimic mutation of Thr171 caused no effect on MoOsm1 to form a dimer. (C) Localization of MoOsm1T171D. The observation showed that the MoOsm1T171D located in the cytoplasm.
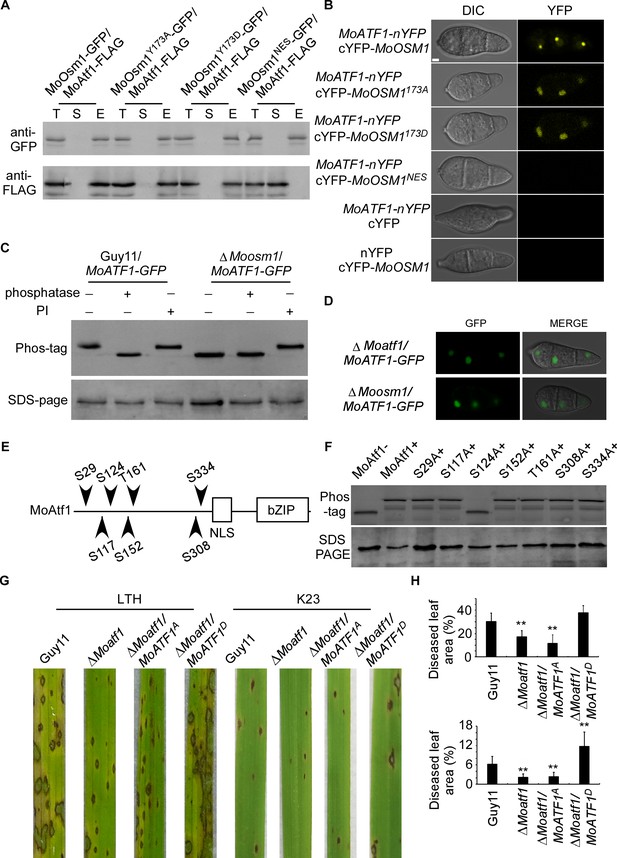
MoOsm1 phosphorylates MoAtf1 in the nucleus.
(A) Co-IP assay. Western blot analysis of total proteins (T) extracted from MoOsm1-GFP/MoAtf1-FLAG, MoOsm1Y173A-GFP/MoAtf1-FLAG, MoOsm1Y173D-GFP/MoAtf1-FLAG, MoOsm1NES-GFP/MoAtf1-FLAG strains, suspension proteins (S), and elution proteins (E) eluted from anti-GFP beads. The presence of MoAtf1 and MoOsm1 was detected with the anti-GFP and anti-FLAG antibodies, respectively. MoOsm1NES-GFP was used as a negative control. (B) BiFC assays for the interaction between MoOsm1 and MoAtf1. Strains expressed MoAtf1-nYFP and empty cYFP, cYFP-MoOsm1 and empty nYFP, MoAtf1-nYFP and MoOsm1NES-GFP were used as negative controls. Bars = 5 μm. (C) Phosphorylation of MoAtf1 by MoOsm1. MoAtf1-GFP proteins treated with alkaline phosphatase and phosphatase inhibitors (PIs) were separated by Mn2+-Phos-tag and normal SDS-PAGE, respectively, and detected by the GFP antibody. (D) The localization of MoAtf1 in ∆Moosm1 and ∆Moatf1 mutants. MoAtf1-GFP was introduced in the ∆Moosm1 and ∆Moatf1 mutants, and the localization was observed by confocal fluorescence microscopy. (E) A model of MoAtf1 and mutants’ constructs. White bars indicate the conserved bZIP domain and nuclear localization signal (NLS) domain. The black triangle indicates putative serine and threonine sites and the numbers indicate amino acid positions. (F) Identification of the phosphorylation sites of MoAtf1 by MoOsm1. In vivo phosphorylation analysis of MoAtf1 and the inactivation mutants (S to A and T to A). MoAtf1-GFP proteins treated with alkaline phosphatase (MoAtf1-), PIs (MoAtf1+), and the phosphorylation inactivation mutants treated with PIs (S29A+, S117A+, S124A+, S152A+, T161A+, S308A+, and S334A+) were separated by Mn2+-Phos-tag SDS-PAGE and normal SDS-PAGE, respectively. (G) Pathogenicity assay. Four milliliters of conidial suspension (5 × 104 spores/ml) of each strain were used for spraying on LTH and K23 and photographed 5 d after inoculation. (H) Diseased leaf area analysis. Data are presented as a bar chart showing the percentage of lesion area analyzed by Image J. Error bars represent SD and asterisks represent significant differences (‘**” represents p<0.01).
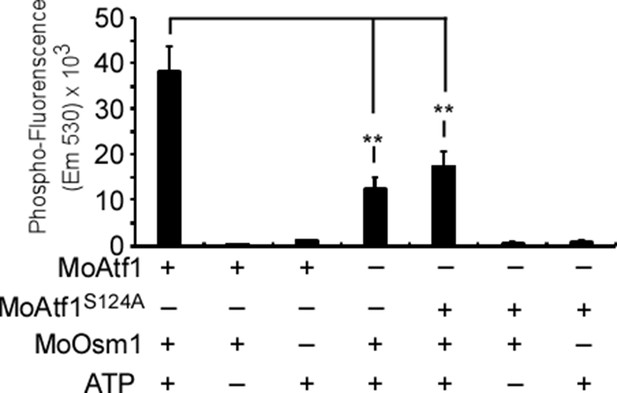
Phosphorylation of MoAtf1 in vitro.
In vitro phosphorylation analysis by the fluorescence detection in the tube (FDIT) method. Purified proteins of MoOsm1-HIS, MoAtf1-GST, and MoAtf1S124A-GST were used for the protein kinase reaction in the presence of 50 μM ATP and the Pro-Q Diamond Phosphorylation Gel dye. Fluorescence signal at 590 nm (excited at 530 nm) was measured in a Cytation3 microplate reader (Biotek, Winooski, VT, USA). Asterisks denote statistical significances (p<0.01).
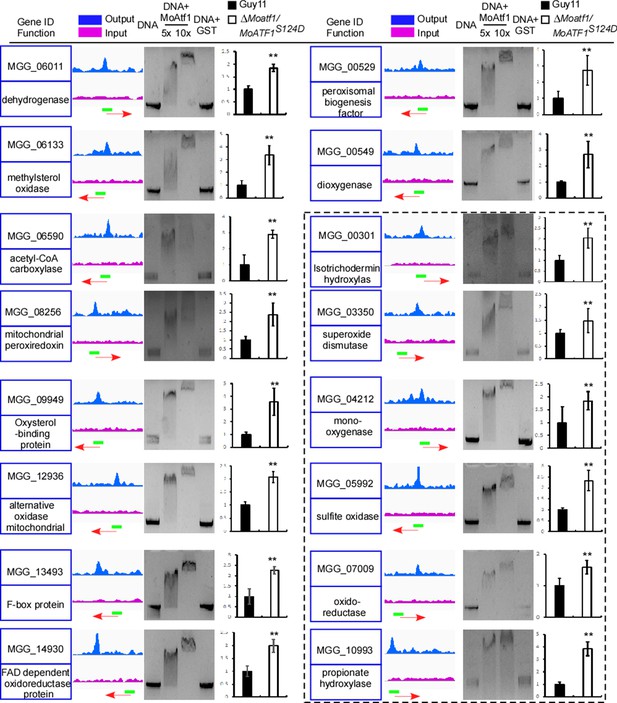
MoAtf1 is one of the regulators of the oxidation regulatory pathway.
ChIP-Seq assays showed that MoAtf1 binds to the promoters of 16 oxidation regulation pathway genes. Output- and input-DNA was visualized in blue and pink. The red arrow indicates gene direction. The green bar represents the promoter region covered during ChIP-Sequencing. Blank in the black dashed line indicates genes encoding proteins containing signal peptides. The EMSA assay was performed to evaluate the relevant promoter of these genes binding with MoAtf1. The purified MoAtf1 was mixed with DNA, incubated for 20 min at 25°C in binding buffer, and separated by 1.5% agarose gel. The qRT-PCR assay showed the expression of these genes in Guy11 and ∆Moatf1/MoAtf1S124D. Three independent biological experiments were performed, with three replicates each time that yielded similar results. Error bars represent standard deviation, and asterisks represent significant differences between the different strains (p<0.01).
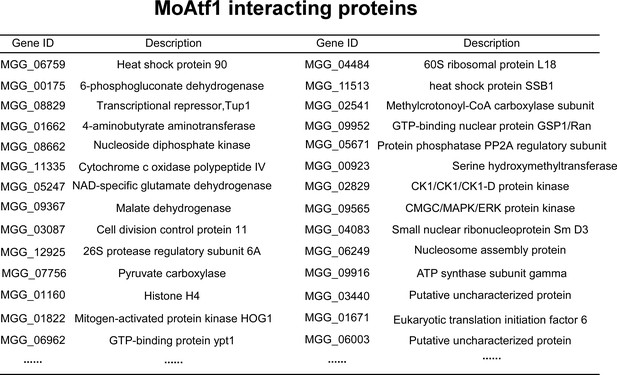
Putative MoAtf1-interacting proteins identified by affinity purification.
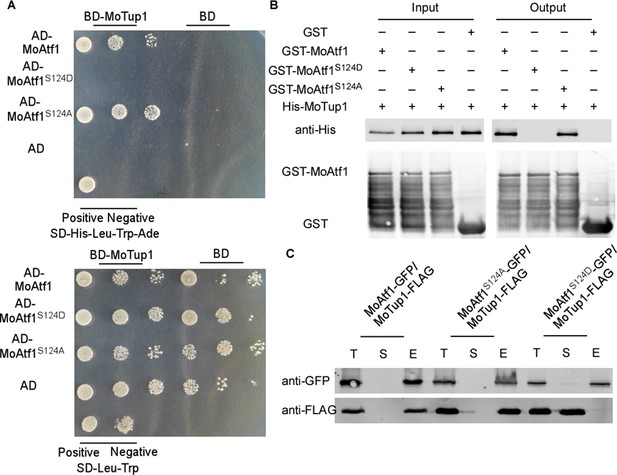
Phosphorylation of MoAtf1 on S124 blocks MoAtf1 and MoTup1 interaction.
(A) Y2H analysis of the interaction between MoAtf1 and MoTup1. The pGADT7 and pGBKT7 fused with specific genes were co-introduced into the yeast AH109 strain, and transformants were plated on SD-Leu-Trp as control and on selective SD-Leu-Trp-His-Ade for 3 days. The pair of plasmids pGBKT7-Lam and pGADT7-T were used as the negative controls. The pair of plasmids pGBKT7-(53+) and pGADT7-T was used as the positive controls. (B) The interaction between MoAtf1, MoAtf1S124A, MoAtf1S124D, and MoTup1 in vitro. In vitro GST-pull down assays to confirm the interaction among MoAtf1-MoTup1, MoAtf1S124A-MoTup1, and MoAtf1S124D-MoTup1 pairs. (C) The MoAtf1-GFP, MoAtf1S124A-GFP, MoAtf1S124D-GFP, and MoTup1 were pulled down using anti-GFP agarose beads in vivo and then subjected to SDS-PAGE followed by immunoblotting. T: total protein; S: suspension; E: Elusion.
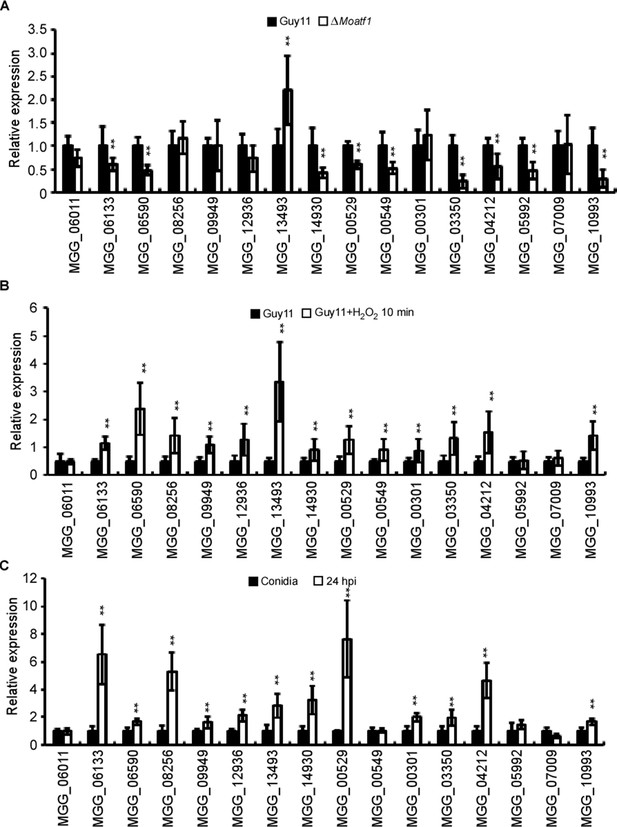
Expression levels of the oxidation regulatory pathway genes in the ∆Moatf1 mutant.
(A) The relative expression levels of putative oxidation regulation pathway genes in ∆Moatf1 mutant were analyzed by qRT-PCR and normalized to that of ACTIN. Error bars represent the standard deviations and asterisks represent significant differences (p<0.01). (B) The relative expression levels of putative oxidation regulation pathway genes with H2O2 treatment for 10 min were analyzed by qRT-PCR and normalized to that of ACTIN. Error bars represent the standard deviations and asterisks represent significant differences (p<0.01). (C) The relative expression levels of putative oxidation regulation pathway genes in conidia and 24 hpi were analyzed by qRT-PCR and normalized to that of ACTIN. Error bars represent the standard deviations and asterisks represent significant differences (p<0.01).
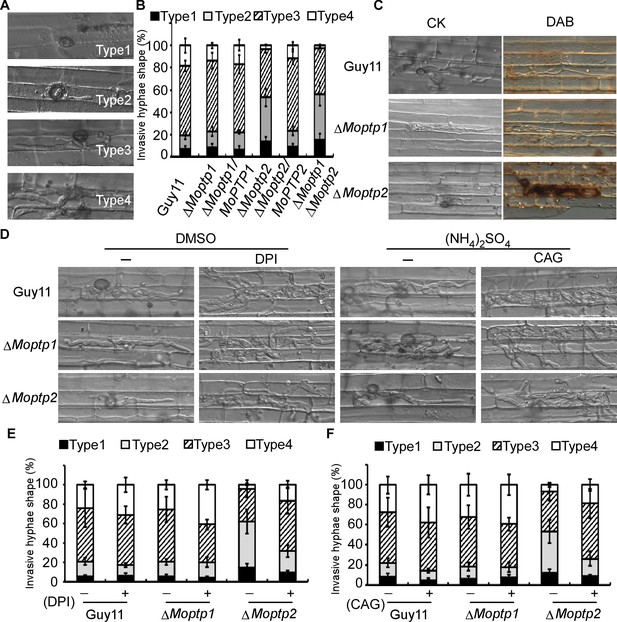
MoPtps are important in host-derived ROS scavenging by M. oryzae.
(A) and (B) Excised rice sheaths from 3-week-old rice seedlings were inoculated with conidial suspension (1 × 105 spores/ml). Infectious growth was observed at 24- and 36-hr post-inoculation (hpi). Appressorium penetration sites (n = 100) were observed and invasive hyphae (IH) were rated from type 1 to 4 (type1, no hyphal penetration with only appressoria formation; type2, IH with 1 or two short branches; type3, IH with at least three branches but the IH are short and extending within a plant cell; type 4, IH that has numerous branches and fully occupies the plant cell or even extended to an adjacent plant cell). The experiment was repeated three times. (C) DAB staining of the excised leaf sheath of infected rice 24 hpi. 50 infecting hyphae were counted per replicate and the experiment was repeated three times. (D) The excised sheath of rice was inoculated with conidial suspension after treated with or without 0.5 μM DPI dissolved in DMSO by three independent experiments. The rice sheath was also inoculated with the conidial suspension after treating with or without 0.2 U Aspergillus niger catalase (CAG, Sigma) dissolved in 10 mM (NH4)2SO4. Samples were harvested and observed 36 hr after inoculation. (E) and (F) Quantification of the infection progress with DPI and CAG treatment, over 50 infecting hyphae were counted per replicate, and the experiment was repeated three times.
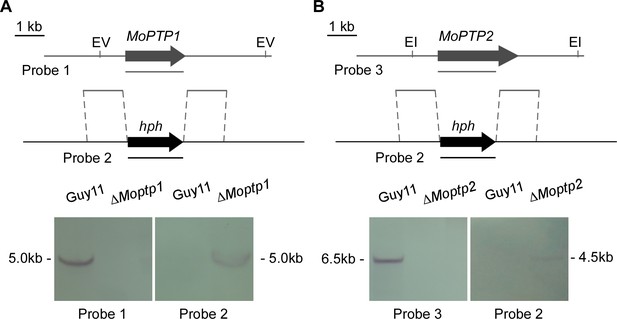
Southern blot analysis of MoPTP1 and MoPTP2 deletional mutants.
Southern blot analysis of the gene knockout mutant with gene-specific probe (probe one for MoPTP1 and probe three for MoPTP2) and hygromycin phosphotransferase (HPH) probe (probe 2). The scheme of MoPTP1 and MoPTP2 gene disruption.
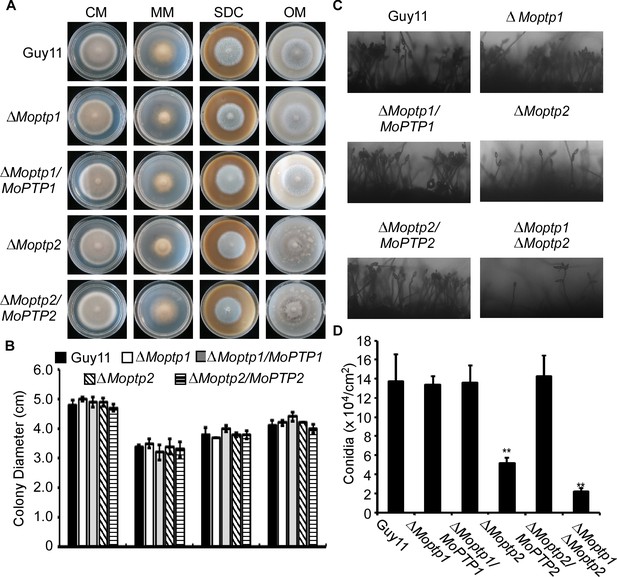
MoPtps are not involved in mycelial growth and conidiation.
(A) Comparison of ∆Moptps and wild-type strain in colony morphology. Wild type, the ∆Moptps mutant and complementation strains were cultured at 28°C in darkness for 7 days. (B) Statistical analyses of the diameter of hypha of wild-type Guy11, the ∆Moptps mutant, and the complemented strain on different medium. Error bars represent the standard deviations and asterisks denote statistical significances (p<0.01) (C) Conidia development of the wild type, ∆Moptp1, ∆Moptp2, and the complement strains on SDC medium for 7 days were examined by light microscopy. (D) Statistical analysis of conidia production. Conidia produced by the wild-type, the mutants, and complemented strains on SDC medium for 10 days were collected, counted, and analyzed. ± SD is calculated from three repeated experiments and asterisks indicate statistically significant differences (Duncan's new multiple range test, p<0.01).
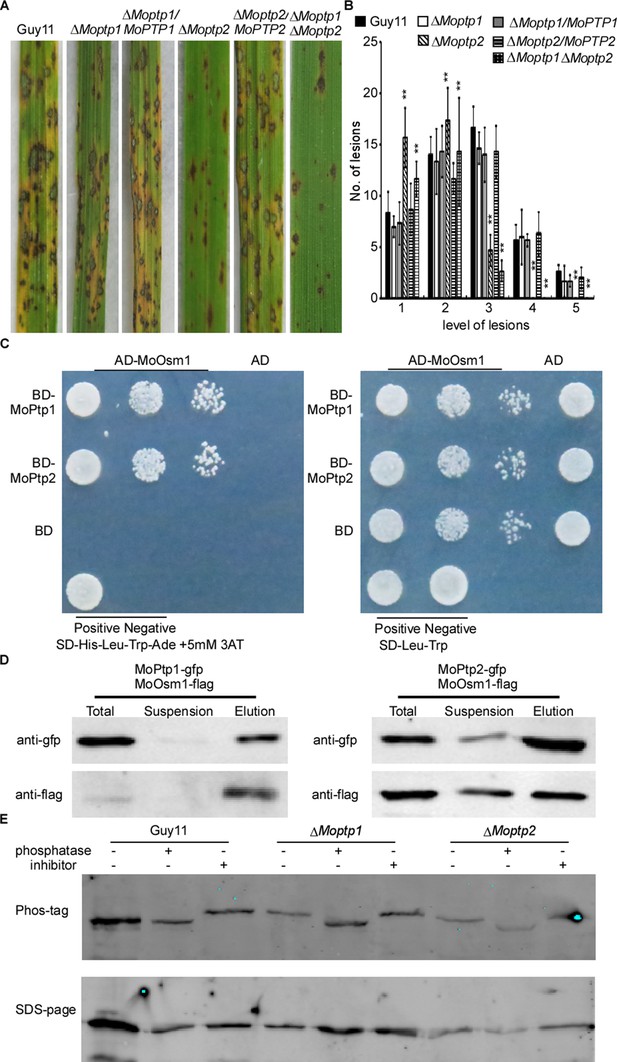
MoPtp1 and MoPtp2 are involved in dephosphorylation of MoOsm1.
(A) Pathogenicity assay. Conidial suspensions were sprayed onto two-week-old rice seedlings (CO-39). Diseased leaves were photographed after 7 days of inoculation. (B) Quantification of lesion type. Lesions were photographed and measured at 7 days post-inoculation (dpi); Numbers of lesions were counted in a total area of 4 cm2. Experiments were repeated three times with similar results. Asterisks indicate significant differences at p=0.01. (C) Y2H analysis of interactions between MoOsm1 and MoPtp1 and MoPtp2. Plates were incubated at 30°C for 3 days before being photographed. (D) Co-IP assay. Western blot analysis of total proteins extracted from mycelia of various transformants, protein suspensions, and eluted proteins. (E) Dephosphorylation of MoOsm1 by MoPtp1/2. Various sources of MoOsm1-GFP proteins treated with phosphatase and phosphatase inhibitors were respectively separated by Mn2+-Phos-tag SDS-PAGE and normal SDS-PAGE and detected with the GFP antibody.
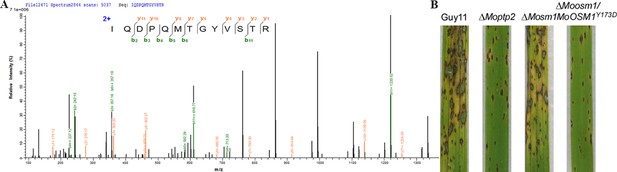
Hyperphosphorylation on MoOsm1 Tyr173 causes virulence defect in M. oryzae.
(A) MoOsm1 phosphopeptide (QDPQMTGYVSTR) in the ΔMoptp2/MoOSM1-GFP strain was identified by mass spectrometer analysis and the phosphorylated sites were Tyr-173. (B) The pathogenicity assay on rice leaves. Conidial suspensions of strains were sprayed onto two-week-old rice seedlings (CO-39). Diseased leaves were photographed after 7 days of inoculation.
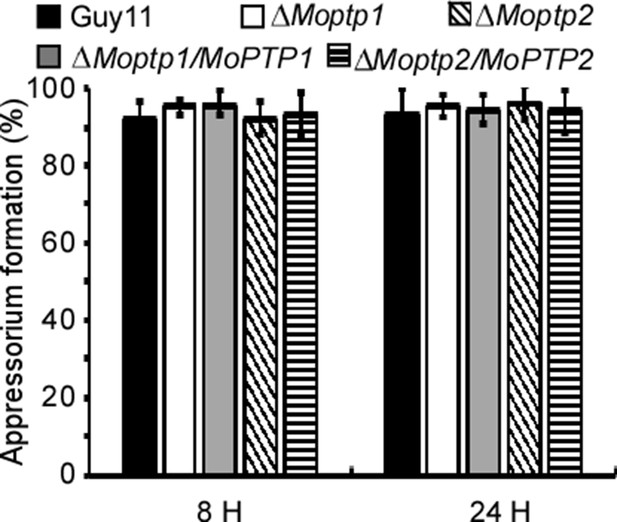
Appressorium formation in the ∆Moptps mutants.
Appressorium formation rates at different time points were calculated and statistically analyzed. The percentage at a given time was recorded by observing at least 200 conidia for each strain and the experiment was repeated three times. Error bars represent SD.
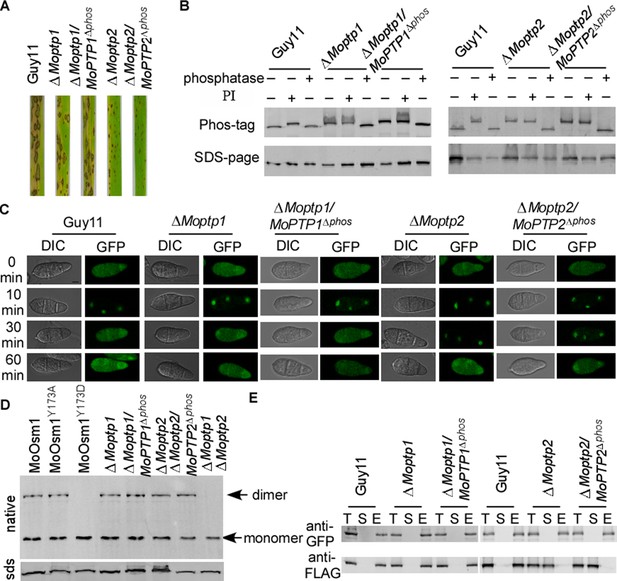
MoPtps-mediated dephosphorylation of MoOsm1 leads to its nuclear exporting and monomerization.
(A) Pathogenicity assay. Conidial suspensions were sprayed onto two-week-old rice seedlings (CO-39). Diseased leaves were photographed after 7 days of inoculation. (B) Dephosphorylation of MoOsm1 dependent on the phosphatase activity of MoPtp1/2. MoOsm1-GFP proteins of various sources treated with alkaline phosphatase and phosphatase inhibitors were separated by Mn2+-Phos-tag SDS-PAGE. (C) Fluorescence observation of conidia untreated (top panels) and treated with 5 mM H2O2 for 10, 30, and 60 min (lower panels). Bar = 5 μm. (D) The total proteins of MoOsm1-GFP/∆Moosm1, MoOsm1Y173A-GFP/∆Moosm1, MoOsm1Y173D-GFP/∆Moosm1, ∆Moptp1, ∆Moptp1/MoPTP1∆ptpc, ∆Moptp2, and ∆Moptp2/MoPTP2∆ptpc were subjected to Native-PAGE followed by immunoblotting analysis. (E) Western blot analysis of MoAtf1-MoTup1 interactions in ∆Moptp1/2 mutant strains. The presence of MoAtf1-GFP and MoTup1-FLAG was detected with the anti-GFP and anti-FLAG antibodies, respectively. T: total proteins; S: suspension proteins; and E: elution proteins.
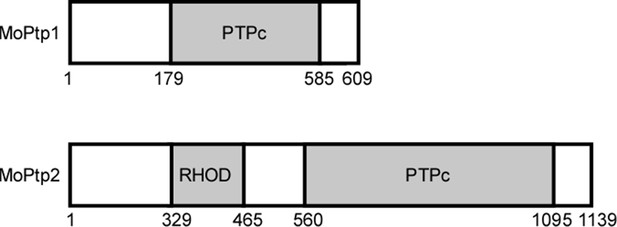
A comparison of MoPtp1 and MoPtp2 functional domains.
A model of MoPtp1, MoPtp2, and mutants constructs. Black bars indicate the conserved phosphatase-domain (PTPc) in MoPtp1 and MoPtp2 that were mutanted in ∆Moptp1/MoPTP1∆ptpc and ∆Moptp2/MoPTP2∆ptpc. RHOD represents the Rhodanese Homology Domain in MoPtp2. Numbers indicate amino acid positions. The expressions of MoPTP1∆ptpc and MoPTP2∆ptpc were evalutaed by qRT-PCR.
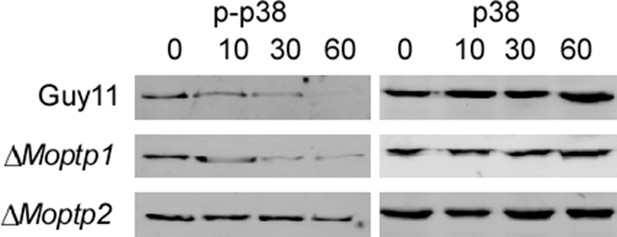
MoOsm1 phosphorylation levels in ∆Moptp1/2 mutants under oxidative stress.
Immunoblots were performed with total proteins from Guy11, ΔMoptp1, and ΔMoptp2 mutant strains without or with 5 mM H2O2 for 10, 30, and 60 min. The MoOsm1 phosphorylation level was detected using the P-P38 antibody. MoOsm1 was detected by the p38 antibody as control.
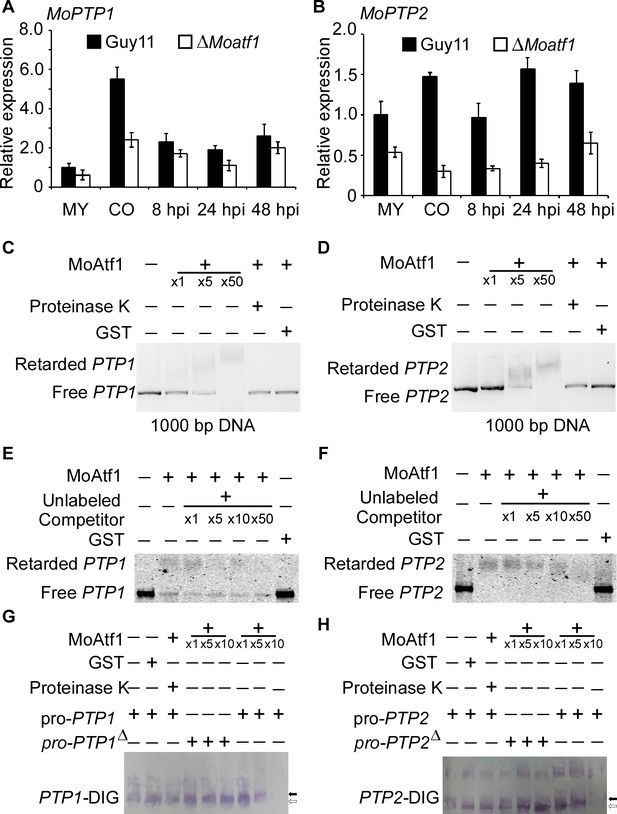
MoAtf1 binds to the promoter regions of MoPTP1 and MoPTP2.
(A) Expression analysis of MoPTP1 in the ∆Moatf1 mutant and wild-type strains. The expression of MoPTP1 was analyzed by qRT-PCR and normalized to that of actin gene (MGG_03982). MY represents mycelium and CO represents conidium. Error bars represent the standard deviations and asterisks represent significant differences (p<0.01). (B) Expression analysis of MoPTP2 in the ∆Moatf1 mutant and wild-type strains. (C) and (D) The full-length promoter sequence of MoPTP1 and MoPTP2 was incubated in the absence (leftmost lane) or presence (second to the fourth lane with increasing amounts of MoAtf1) of purified MoAtf1 and GST protein (rightmost lane). The proteinase K was added after the incubation of MoAtf1 with the DNA (fifth lane). DNA-protein complexes were separated by electrophoresis on a 1.2% agarose gel. (E) and (F) The Alex660-labeled full-length DNA of promoter was incubated in the absence (leftmost lane) or presence (second to the sixth lane) of the purified MoAtf1 and GST protein (rightmost lane). Unlabeled DNA was added as a binding competitor with increasing amounts in lanes from third to sixth. DNA-protein complexes were separated by electrophoresis on a 1.2% agarose gel. (G) The DIG-labeled promoter of MoPTP1 was incubated with an increasing amount of MoAtf1 prior to separation by PAGE. pro-PTP1: full-length promoter. pro-PTP1∆: the cis-element deletion promoter. The white arrow represents free DNA, the black arrow represents migrated DNA. (H) The DIG-labeled promoter of MoPTP2 was incubated with an increasing amount of MoAtf1. The white arrow represents free DNA, the black arrow represents migrated DNA.
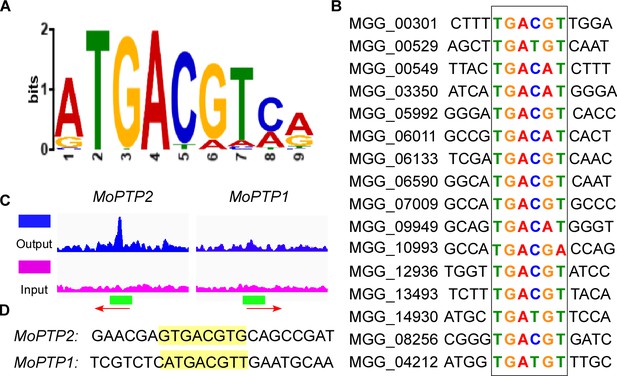
The ChIP assay identifies MoPTP1 and MoPTP2 as putative targets of MoAtf1.
(A) The cis-element of MoAtf1 was predicted by MEME. The putative sequence of the cis-element obtained by analyzing the promoters of MoAtf1 binding genes with the MEME program. (B) Schematic representation of cis-elements in the promoters of oxidation regulation pathway genes. The conserved TGAY(C/T)R(G/A)W(T/A) motif is indicated by the black squares. (C) ChIP-Seq assays show that MoAtf1 binds to the promoter of MoPTP2. Output- and input-DNA samples were visualized in blue and pink. The red arrow indicates the gene direction from 5’ to 3’. The green bar represents the gene promoter region, which was revealed during ChIP-Sequencing. (D) Schematic representation of the cis-element in the promoters of oxidation regulation pathway genes. Conserved TGACGT motifs within the cis-elements are highlighted.
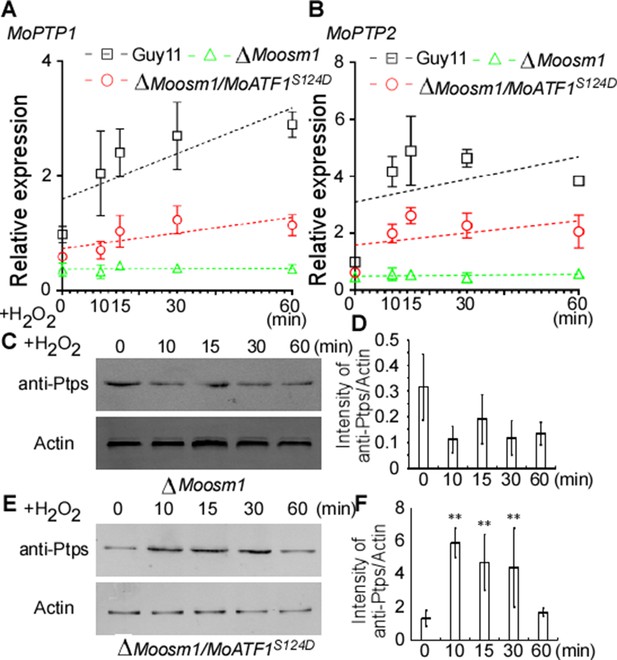
MoAtf1 phosphorylation controls the transcription of MoPTP1 and MoPTP2 in response to oxidative stress.
(A) and (B) MoPTP1 and MoPTP2 expression analysis in Guy11, ∆Moosm1 mutant, and ∆Moosm1/MoAtf1S124D strains treated with H2O2 for 15, 30, and 60 min. Three independent biological experiments were performed, with three replicates each time, and yielded similar results in each independent biological experiment. Dotted lines represent the expression of MoPTP1 and MoPTP2 in these strains under H2O2 stress. Error bars represent standard deviation. (C) Total proteins were extracted from ∆Moosm1 treated with H2O2 for 15, 30, and 60 min. MoPtp1/2 was detected by western blotting analysis using anti-ptp1/2 antibodies. An anti-Actin antibody was used as control. (D) Western blotting bands were quantified with an ODYSSEY infrared imaging system (application software Version 2.1). Bars denote standard errors from three independent experiments. Asterisks indicate significant differences (Duncan's new multiple range test p<0.01). (E) Total proteins were extracted from ∆Moosm1/MoAtf1S124D strain for MoPtp1/2 detection. (F) Western blotting bands of MoPtp1 in ∆Moosm1/MoAtf1S124D strain was quantified with an ODYSSEY infrared imaging system.
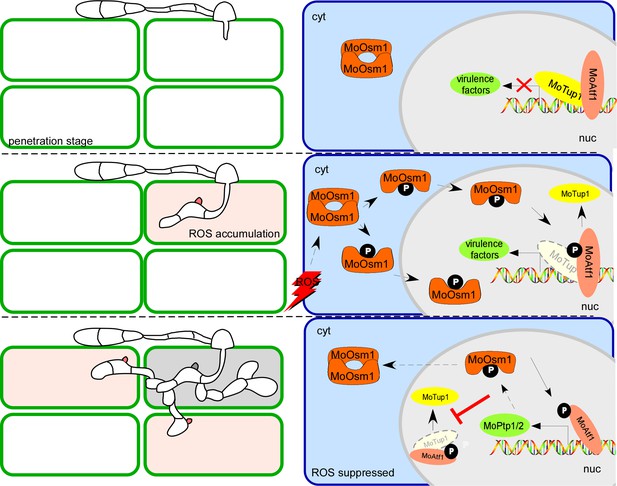
A proposed model depicting MoOsm1/MoAtf1/MoTup1/MoPtp1/2 mediated ROS signaling and responses to host immunity.
Rice generates immunity, including ROS burst during its interaction with M. oryzae. Once host perception, M. oryzae induces MoOsm1 phosphorylation that disintegrates MoOsm1 dimerization leading to enhanced nuclear translocation of MoOsm1. MoOsm1 phosphorylates MoAtf1 uncoupling MoAtf1-MoTup1 interaction that induces the expression of oxidation regulation pathway genes. At the same time, the phosphorylated MoAtf1 promotes the expression of two phosphatases, MoPTP1 and MoPTP2, that dephosphorylate MoOsm1 to suppress MoAtf1-MoTup1 dissociation. MoPtp1/2-mediated MoOsm1 dephosphorylation provides an act balancing infection and its hemibiotrophic growth in rice.
Additional files
-
Supplementary file 1
574 putative binding proteins of MoAtf1 by CHIP assay.
Genomic location and Annotation ID of MoAtf1 binding genes.
- https://cdn.elifesciences.org/articles/61605/elife-61605-supp1-v1.docx
-
Transparent reporting form
- https://cdn.elifesciences.org/articles/61605/elife-61605-transrepform-v1.docx