Pollen Tube Guidance: Growing straight through walls
In a flowering plant, reproduction begins when grains of pollen stick to cells called stigma papillae that are located at the top of the pistil, which is the female part of the flower. A cell called a pollen tube then delivers the sperm cells contained in the pollen grains to the female gametes for fertilization. This is a long journey that involves the pollen tube travelling from the stigma papillae at the top of the pistil to the ovules that contain the female gametes, which are at the bottom of the pistil.
So how does the plant ensure that the pollen tube – which is a single cell that grows longer over time – finds the ovules and does not get lost en route? Several molecules and nutrients secreted by the pistil direct the growth of the pollen tube (Higashiyama and Takeuchi, 2015). However, the identity of the cues that guide the pollen tube in the first stages of its journey have remained a mystery.
Most plant cells grow by increasing their surface area while remaining attached to neighboring cells: pollen tubes are different in that they are tip-growing cells that can grow through the walls of other cells to reach their target. When the pollen tube first enters the pistil, it remains within the cell wall of the stigma papillae (Figure 1; left): could the components of this cell wall, or the mechanical properties of these cells, influence the growth of the pollen tube?
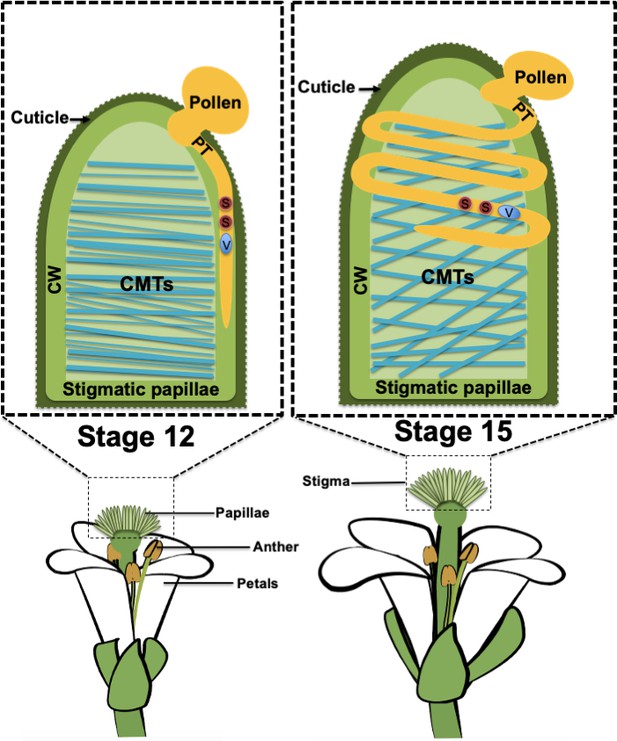
Pollen tube growth in stigma papillae.
When a grain of pollen (shown in mustard) lands on a papilla in the stigma (green) of a flowering plant, a pollen tube (PT; also shown in mustard) begins to grow through the cell wall (CW) of the papilla so that the sperm cells (S; red) in the pollen can be delivered to the female gametes, which are located in ovules deep inside the plant. In stage 12 flowers (left), the organization of the cortical microtubules (CMTs; blue lines) inside the papilla is highly anisotropic and the pollen tube grows in a straight line. In older stage 15 flowers (right), the organization of the microtubules is isotropic and the pollen tube forms a coil around the papilla as it grows. The vegetative cell (V) makes up the body of the pollen tube and encloses the sperm cells.
A number of studies have demonstrated how mechanical properties can influence a variety of cellular processes – including proliferation, differentiation, migration and cell signaling – in animal cells (Discher et al., 2005; Fu et al., 2010; Provenzano and Keely, 2011), and there is evidence that mechanical properties can also shape plant growth and development (Eng and Sampathkumar, 2018; Sampathkumar et al., 2019). For example, it is known that when a pollen tube penetrates the cell wall of a stigma papilla, it causes changes in the mechanical properties of the cell wall by exerting pressure (Zerzour et al., 2009; Sanati Nezhad and Geitmann, 2013).
However, the role of these mechanical properties in regulating the growth of pollen tube has not been explored in detail. Moreover, although the pollen tube is a good model for understanding the behavior of plant cells, and has been used in numerous in vitro studies of tip growth, it has proved challenging to study the directed growth of pollen tubes through the cell walls of stigma papillae in vivo. Now, in eLife, Thierry Gaude and co-workers at the Université de Lyon – including Lucie Riglet as first author – report the results of experiments on the model plant Arabidopsis thaliana that combine the power of microscopy, genetics, and chemical biology to provide new insights into the regulation of pollen tube growth (Riglet et al., 2020).
As stigmas age, they become less receptive to pollen (Gao et al., 2018), and the observation that pollen tubes tend to coil around papillae in aging stigmas forms the basis of this study. Riglet et al. found that aging was associated with changes in the organization of the cortical microtubules in the cytoskeleton: the orientations of these microtubules were more isotopic in older stigmas than in younger stigmas (Figure 1). To test the hypothesis that the organization of these microtubules has a role in directing pollen tube growth, the researchers examined plants that had a loss of function mutation in an enzyme called KATANIN (KTN1): this enzyme can sever microtubules, and thus allows microtubules to be re-oriented following mechanical stimulation (Sampathkumar et al., 2014). Riglet et al. found that pollen tubes coiled around the papillae in both young and old mutant plants: this indicates that the arrangement of the microtubules affects the ability of pollen tubes to grow straight through the cell walls and into the rest of the pistil.
Cortical microtubules are associated with cellulose synthesis, so the researchers tested whether the stiffness and composition of the cell wall in mutant and aging papillae was associated with pollen tube coiling. They found that softer cell walls and isotropic arrangements of cellulose microfibrils in mutant and aging papillae were associated with faster pollen tube growth and loss of directionality. Overall, the latest work supports the thesis that the mechanical properties and cell wall composition of the stigma papillae have an influence on pollen tube growth and help to guide it through the stigma. Moreover, by providing fundamental insights into the process of sexual reproduction in plants, the work is also relevant in the context of global food security as pollen-stigma interactions are critical for successful pollination and seed production in flowering plants.
Apart from pollen tubes, several types of plant, animal and fungal cells grow invasively, including root hairs, fibroblasts, cancer cells and fungal hyphae. In the future, it will be important to determine the contribution of mechanical forces to invasive growth. New technological advances such as lab-on-a-chip, MEMS (micro-electro-mechanical systems), deep-tissue imaging and computational tools will help researchers to measure the mechanical forces operating on and in cells (Nezhad et al., 2013). The pollen tube/pistil system will also make it possible to explore how chemical guidance cues work together with mechanical forces to regulate directional cell growth.
References
-
Getting into shape: the mechanics behind plant morphogenesisCurrent Opinion in Plant Biology 46:25–31.https://doi.org/10.1016/j.pbi.2018.07.002
-
The mechanism and key molecules involved in pollen tube guidanceAnnual Review of Plant Biology 66:393–413.https://doi.org/10.1146/annurev-arplant-043014-115635
-
Physical forces regulate plant development and morphogenesisCurrent Biology 24:R475–R483.https://doi.org/10.1016/j.cub.2014.03.014
-
The cellular mechanics of an invasive lifestyleJournal of Experimental Botany 64:4709–4728.https://doi.org/10.1093/jxb/ert254
Article and author information
Author details
Publication history
Copyright
© 2020, Sankaranarayanan and Kessler
This article is distributed under the terms of the Creative Commons Attribution License, which permits unrestricted use and redistribution provided that the original author and source are credited.
Metrics
-
- 4,126
- views
-
- 252
- downloads
-
- 1
- citations
Views, downloads and citations are aggregated across all versions of this paper published by eLife.
Download links
Downloads (link to download the article as PDF)
Open citations (links to open the citations from this article in various online reference manager services)
Cite this article (links to download the citations from this article in formats compatible with various reference manager tools)
Further reading
-
- Plant Biology
It is well documented that type-III effectors are required by Gram-negative pathogens to directly target different host cellular pathways to promote bacterial infection. However, in the context of legume–rhizobium symbiosis, the role of rhizobial effectors in regulating plant symbiotic pathways remains largely unexplored. Here, we show that NopT, a YopT-type cysteine protease of Sinorhizobium fredii NGR234 directly targets the plant’s symbiotic signaling pathway by associating with two Nod factor receptors (NFR1 and NFR5 of Lotus japonicus). NopT inhibits cell death triggered by co-expression of NFR1/NFR5 in Nicotiana benthamiana. Full-length NopT physically interacts with NFR1 and NFR5. NopT proteolytically cleaves NFR5 both in vitro and in vivo, but can be inactivated by NFR1 as a result of phosphorylation. NopT plays an essential role in mediating rhizobial infection in L. japonicus. Autocleaved NopT retains the ability to cleave NFR5 but no longer interacts with NFR1. Interestingly, genomes of certain Sinorhizobium species only harbor nopT genes encoding truncated proteins without the autocleavage site. These results reveal an intricate interplay between rhizobia and legumes, in which a rhizobial effector protease targets NFR5 to suppress symbiotic signaling. NFR1 appears to counteract this process by phosphorylating the effector. This discovery highlights the role of a bacterial effector in regulating a signaling pathway in plants and opens up the perspective of developing kinase-interacting proteases to fine-tune cellular signaling processes in general.
-
- Plant Biology
- Structural Biology and Molecular Biophysics
The Calvin-Benson-Bassham cycle (CBBC) performs carbon fixation in photosynthetic organisms. Among the eleven enzymes that participate in the pathway, sedoheptulose-1,7-bisphosphatase (SBPase) is expressed in photo-autotrophs and catalyzes the hydrolysis of sedoheptulose-1,7-bisphosphate (SBP) to sedoheptulose-7-phosphate (S7P). SBPase, along with nine other enzymes in the CBBC, contributes to the regeneration of ribulose-1,5-bisphosphate, the carbon-fixing co-substrate used by ribulose-1,5-bisphosphate carboxylase/oxygenase (Rubisco). The metabolic role of SBPase is restricted to the CBBC, and a recent study revealed that the three-dimensional structure of SBPase from the moss Physcomitrium patens was found to be similar to that of fructose-1,6-bisphosphatase (FBPase), an enzyme involved in both CBBC and neoglucogenesis. In this study we report the first structure of an SBPase from a chlorophyte, the model unicellular green microalga Chlamydomonas reinhardtii. By combining experimental and computational structural analyses, we describe the topology, conformations, and quaternary structure of Chlamydomonas reinhardtii SBPase (CrSBPase). We identify active site residues and locate sites of redox- and phospho-post-translational modifications that contribute to enzymatic functions. Finally, we observe that CrSBPase adopts distinct oligomeric states that may dynamically contribute to the control of its activity.